Correlation of serum nicotinamide phosphoribosyl transferase with hypoxia and Framingham risk score in patients with obstructive sleep apnea-hypopnea syndrome
Introduction
Niacinamide phosphoribosyltransferase (NAMPT) plays an important role in the biological activities of the intracellular and extracellular environments. Intracellular NAMPT (iNAMPT) is the rate-limiting enzyme of the nicotinamide adenine dinucleotide (NAD+) remediation pathway and is involved in regulating cellular redox reactions and maintaining mitochondrial function (1,2). Extracellular NAMPT (eNAMPT) is closely related to the occurrence and development of atherosclerosis (AS) (3-5). Recent studies have reported that NAMPT can promote the expression and release of adhesion molecules and proinflammatory factors to cause vascular injury (6-9). NAMPT can also accelerate plaque formation by inducing the formation of macrophage foam cells (10,11) and the proliferation of vascular smooth muscle cells (12,13). Additionally, NAMPT can promote plaque vulnerability and accelerate AS complications by regulating extracellular matrix degradation and angiogenesis (14,15). Therefore, NAMPT, as a marker of vascular injury and a potential therapeutic target for AS, has received increasing attention (16-18).
Although different from the classic causes of AS (such as hyperlipidaemia and hyperglycaemia), obstructive sleep apnea-hypopnea syndrome (OSAHS), based on chronic intermittent hypoxia (CIH), has been reported in epidemiological studies to be an independent risk factor for cardiovascular adverse events and AS (19-25). Clinical studies have confirmed that OSAHS is associated with many cardiovascular complications and is an independent risk factor for the occurrence, progression, and mortality of cardiovascular and cerebrovascular disease (CVD) (26,27). A meta-analysis of 16 studies showed that severe OSAHS [apnea-hypopnea index (AHI) ≥30] was associated with increased all-cause and cardiovascular mortality (28). However, the specific mechanism and therapeutic target remain unclear. Recently, hypoxia has been reported to promote the expression of eNAMPT in vascular endothelial cells, thereby activating a series of signalling pathways to cause endothelial dysfunction (29,30). Therefore, we speculated that NAMPT could also play an important role in AS induced by OSAHS.
Currently, the expression of NAMPT in patients with OSAHS has not been reported, and further studies are required to determine if polysomnography (PSG)-derived hypoxia-related parameters have an impact on NAMPT in patients with OSAHS. Therefore, from a clinical research perspective, this paper clarified the activity and expression of serum NAMPT in patients with OSAHS and its correlation with the severity of OSAHS. Additionally, the effects of PSG-derived hypoxia-related parameters on the expression of NAMPT in patients with OSAHS were investigated using further multivariate regression analysis. Finally, the Framingham risk score (FRS), which predicts the degree of cardiovascular injury at 10 years, was used as an outcome index to analyze the correlation between NAMPT and cardiovascular disease risk in patients with OSAHS. The relationship and value of NAMPT in OSAHS patients with disease degree and cardiovascular disease risk were preliminarily evaluated to lay a foundation for subsequent basic research on the prevention and treatment of cardiovascular and cerebrovascular diseases caused by OSAHS. We present the following article in accordance with the STROBE reporting checklist (available at https://apm.amegroups.com/article/view/10.21037/apm-22-975/rc).
Methods
Study participants
A cross-sectional study design was adopted for this study. Patients diagnosed with OSAHS and admitted to the Department of Otolaryngology, Head and Neck Surgery, The First Hospital of Hebei Medical University between November 2019 and December 2021 were selected. General characteristics and clinical information of the patients were prospectively collected through continuous enrolment.
According to the diagnostic guidelines for patients with OSAHS and the study requirements, the inclusion criteria were as follows: (I) adult patients (age >18 years), (II) typical symptoms such as nocturnal snoring with apnea and daytime somnolence [Epworth Sleepiness Scale (ESS) score ≥9], and (III) physical examination showing stenosis and obstruction in any part of the upper airway and recurrent apnea and hypopnea occurring more than 30 times or sleep AHI ≥5 times/hour during the 7-hour sleep cycle each night.
The exclusion criteria were as follows: (I) patients with chronic obstructive pulmonary disease, pulmonary hypertension, bronchial asthma, other pulmonary diseases, and other sleep breathing disorders, including upper airway resistance syndrome, restless leg syndrome or narcolepsy, who were diagnosed before or during hospitalization; (II) patients with heart, liver, or respiratory failure, chronic kidney disease, mental disorders, and other systemic diseases; (III) patients who had taken or were taking sleep-affecting respiratory drugs and lipid-lowering drugs within a short period before the visit; (IV) patients with upper respiratory tract infection within a short time before the visit; and (V) patients who did not agree to join the group and did not provide informed consent.
Through the inclusion and exclusion criteria, a total of 82 patients (17 in the mild moderate group and 65 in the severe group) with OSAHS were finally included in the study, and 18 healthy control subjects matched with their baseline characteristics of age, gender, smoking and drinking were randomly selected from outpatients who received regular medical examinations at our institution.
Medical ethics
This study was approved by the Ethics Review Committee of the First Hospital of Hebei Medical University (Ethics Approval No. 20200623) and conducted in accordance with the declaration of Helsinki (as revised in 2013). All clinical information was obtained from the clinical sample database of the hospital, and all patients gave written informed consent.
General data collection
A detailed medical history and questionnaire survey were conducted for each participant, which included: (I) basic personal information, including gender, age, detailed smoking and drinking history, and family history; (II) OSAHS-related symptoms, including snoring, waking up in the middle of the night, feeling dizzy in the morning, and mental state during the day; (III) detailed inquiries about the history of hypertension, diabetes, hyperlipidaemia, and other related diseases; (IV) the severity of daytime subjective sleepiness, which was assessed using ESS in Chinese.
Physical measurement data
Anthropometric indicators, including height, weight, and waist circumference (WC), were measured at baseline by an experienced physician. The patient’s weight was measured using electronic scales. WC was measured at the midpoint perimeter between the lowest rib and iliac crest. After a 15-minute rest, the patient was seated and blood pressure, systolic pressure (SBP), and diastolic pressure (DBP) were measured using a mercury sphygmomanometer. All of the above basic parameters were measured twice and averaged.
Biochemical index measurement data
Fasting blood samples were collected from the anterior elbow vein of all participants the morning after admission. Blood biochemical examination was conducted using routine procedures, and metabolic indicators such as blood glucose and lipid markers were collected to measure fasting blood glucose, triglyceride (TG), total cholesterol (TC), low-density lipoprotein cholesterol (LDL-C), and high-density lipoprotein cholesterol (HDL-C).
PSG
Sleep monitoring included the electroencephalogram (EEG), electrocardiogram (ECG), electronystagmogram (EOG), electromyogram (EMG) of submental muscles, blood oxygen saturation, airflow of the nose and mouth, thoracoabdominal motility, and body position. Sleep event data included apnea, oxygenated haemoglobin saturation, AHI, mean pulse oxygen saturation (MSpO2), oxygen desaturation index (ODI); lowest pulse oxygen saturation (LSpO2), and time when blood oxygen saturation was below 90% (TS90).
Calculation of FRS
Calculation of FRS was performed based on the method by McPherson et al. (31) Variables required for the calculation of FRS included gender, age, HDL, TC, SBP, and smoking. The scores corresponding to each factor were collected to obtain the FRS value for each patient.
Determination of NAMPT concentration in the peripheral blood
The serum concentration of NAMPT was measured using a double-antibody sandwich enzyme-linked immunosorbent assay following the manufacturer’s instructions.
Sample size
According to studies on influencing variables, sample size is considered sufficient if the number of patients is at least 5–10 times the number of independent variables. As 12 independent variables were examined in the present study, the sample size was estimated to be at least 60–120 cases.
Statistical analysis
Data analysis was performed using SPSS 21.0 and R software. Measurement data conforming to normal distribution are expressed as mean ± standard deviation, and the comparison of the mean between 2 groups was performed using a student’s t-test. Measurement data that did not conform to normal distribution are represented as the median (interquartile spacing). The comparison of the median between 2 groups was performed using the nonparametric Mann-Whitney U test, and the chi-square test was used for count data. Univariate and multivariate linear regression models were established to analyze the effect of NAMPT on FRS in patients with OSAHS. Spearman correlation analysis was used to clarify the clinical and PSG indicators closely related to the activity level of NAMPT in OSAHS patients and to remove confounding factors through multiple regression analysis to explore the exact effect of hypoxia on NAMPT in OSAHS patients. P<0.05 was considered statistically significant.
Results
General clinical data of participants
A total of 160 hospitalized patients with OSAHS were enrolled consecutively. Of these patients, there were 17 with chronic obstructive pulmonary disease and lung diseases such as bronchial asthma and other sleep disorders, 30 associated with sleep-affecting respiratory drugs within a short period before the visit, 13 suffering from upper respiratory tract infection before the visit, and 18 who did not agree to be included in the study or did not sign the informed consent, and therefore they were excluded. Finally, 82 patients with OSAHS were included in the study.
Among the patients included in the study, 17 had mild to moderate OSAHS and 65 had severe OSAHS. Additionally, 18 healthy controls who were sex- and age-matched were included. General clinical data and 10-year FRS were compared among the 3 groups. No significant differences in age, sex, smoking history, drinking history, SBP, TC, HDL, LDL, fast blood glucose (FBG), and high-sensitivity C-reactive protein (HS-CRP) were observed among the 3 groups (P>0.05). Body mass index (BMI), WC, DBP, and TG were significantly different among the 3 groups (P<0.05) and increased with the severity of OSAHS. Additionally, FRS increased significantly with the severity of OSAHS (Table 1).
Table 1
Index | Healthy control group (n=18) | OSAHS mild to moderate group (n=17) | OSAHS severe group (n=65) | P value |
---|---|---|---|---|
Age (years) | 42.00±7.28 | 42.88±12.21 | 41.27±9.86 | 0.542 |
Male | 7 (38.9) | 14 (82.4) | 60 (92.3) | 0.427b |
Smoking | 1 (12.5) | 6 (35.3) | 29 (44.6) | 0.200b |
Drinking | 1 (12.5) | 6 (35.3) | 9 (47.7) | 0.136b |
BMI (kg/m2) | 21.00 [5.2] | 26.00 [6.78] | 29.40 [6.40] | ≤0.001a** |
WC (cm) | 74.00 [3.00] | 91.00 [25.25] | 103.50 [13] | ≤0.001a** |
SBP (mmHg) | 124.87±25.47 | 127.93±14.01 | 134.56±15.38 | 0.137c |
DBP (mmHg) | 78.63±11.45 | 82.86±8.63 | 88.91±11.50 | 0.023c* |
TG (mmol/L) | 1.43±0.81 | 1.62±0.75 | 2.16±1.26 | 0.036c* |
TC (mmol/L) | 4.86±0.98 | 4.91±1.13 | 4.93±0.91 | 0.941c |
HDL-C (mmol/L) | 1.01±0.22 | 0.98±0.28 | 0.93±0.17 | 0.540c |
LDL-C (mmol/L) | 2.92±0.63 | 3.26±0.80 | 3.09±0.66 | 0.459c |
FBG (mmol/L) | 4.65 [1.18] | 4.97 [1.29] | 4.95 [1.15] | 0.256a |
HS-CRP (mmol/L) | 2.17 [2.04] | 2.14 [11.81] | 2.25 [12.25] | 0.416a |
FRS (%) | – | 11.24±5.72 | 16.48±6.72 | 0.001c |
Data were presented as n (%), mean ± SD and median [IQR]. a, non-parametric test; b, chi-square test; c, one-way analysis of variance test; *, P<0.05; **, P≤0.001. OSAHS, obstructive sleep apnea-hypopnea syndrome; BMI, body mass index; WC, waist circumference; SBP, systolic blood pressure; DBP, diastolic blood pressure; TG, triglycerides; TC, total cholesterol; HDL-C, high-density lipoprotein cholesterol; LDL-C, low-density lipoprotein cholesterol; FBG, fast blood glucose; HS-CRP, high-sensitivity C-reactive protein; FRS, Framingham risk score.
Serum NAMPT activity in patients with OSAHS
We detected the activity of NAMPT in the serum of the participants and found that it was significantly higher in the serum of the OSAHS group than that of the healthy control group. Furthermore, the higher the severity of OSAHS, the higher the activity of serum NAMPT (P<0.05) (Figure 1).
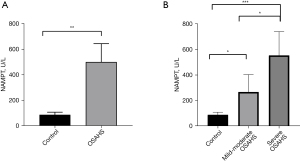
Correlation analysis of serum NAMPT level and FRS in patients with OSAHS
Studies have shown that CIH is the main pathophysiological basis of cardiovascular diseases caused by OSAHS and that the hypoxia-related parameters of patients with OSAHS can predict the risk of cardiovascular diseases. In order to explore the correlation between high activity of NAMPT, which is closely related to hypoxia, and the risk of cardiovascular disease, we performed a Spearman correlation analysis between serum NAMPT level and FRS in patients with OSAHS. A significant positive correlation was observed between NAMPT activity and FRS in patients with OSAHS (r=0.829, 95% CI: 0.751–0.942, P<0.05) (Figure 2).
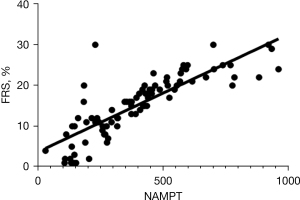
Univariate analysis of the influence of NAMPT and PSG characteristic parameters on FRS in patients with OSAHS
To further explore the correlation and influence of NAMPT activity on FRS in patients with OSAHS, multiple linear regression was performed to determine the independent influence of each indicator on OSAHS patients, with the level of serum NAMPT, structure of sleep at night, degree of hypoxia, and atmospheric tract obstruction as the influencing factors, and FRS as the outcome index. The results showed that serum NAMPT activity and minimum blood oxygen saturation were independently associated with FRS in patients with OSAHS (β=0.03, P=0.000; β=−0.13, P=0.034), with the higher the serum NAMPT activity or the higher the degree of hypoxia in patients with OSAHS, the higher the FRS (Table 2).
Table 2
Index | β | 95% CI | P value |
---|---|---|---|
NAMPT | 0.03 | 0.02 to 0.04 | 0.000* |
LSpO2 | −0.13 | −0.32 to −0.10 | 0.034* |
*, represents statistically significant results. FRS, Framingham risk score; OSAHS, obstructive sleep apnea-hypopnea syndrome; NAMPT, nicotinamide phosphoribosyl transferase; LSpO2, lowest pulse oxygen saturation.
Analysis of related factors of serum NAMPT activity in patients with OSAHS
To explore the correlation between the activity of serum NAMPT and OSAHS, Spearman’s univariate correlation analysis was conducted between the PSG characteristic parameters of patients with OSAHS and serum NAMPT level. The results showed that AHI, total number of respiratory events, ODI, and TS90 were significantly positively correlated with serum NAMPT levels (r=0.341, 95% CI: 0.119–0.545, P=0.002; r=0.290, 95% CI: 0.076–0.480, P=0.009; r=0.504, 95% CI: 0.276–0.747, P=0.000; r=0.255, 95% CI: 0.013–0.458, P=0.028). Moreover, a significant negative correlation was observed between MSpO2 and serum NAMPT level (r=−0.274, 95% CI: −0.505 to 0.009, P=0.014) (Table 3), indicating that the hypoxia parameter was significantly related to serum NAMPT in patients with OSAHS.
Table 3
Index | r value (95% CI) | P value |
---|---|---|
AHI | 0.341 (0.119 to 0.545) | 0.002* |
Total number of respiratory events | 0.290 (0.076 to 0.480) | 0.009* |
TS90 | 0.255 (0.013 to 0.458) | 0.028* |
MSpO2 | −0.274 (−0.505 to 0.009) | 0.014* |
LSpO2 | −0.123 (−0.341 to 0.103) | 0.270 |
ODI | 0.504 (0.276 to 0.747) | 0.000* |
REM | −0.172 (−0.406 to 0.045) | −0.148 |
NREM | 0.205 (−0.017 to 0.414) | 0.071 |
Deep sleep | −0.276 (−0.473 to −0.063) | 0.282 |
HRmax | −0.014 (−0.258 to 0.214) | 0.900 |
HRmin | 0.005 (−0.224 to 0.257) | 0.969 |
Average heart rate | 0.051 (−0.184 to 0.279) | 0.655 |
HRmax-HRmin | −0.044 (−0.282 to 0.170) | 0.703 |
*, represents statistically significant results. PSG, polysomnography; NAMPT, nicotinamide phosphoribosyl transferase; AHI, apnea-hypopnea Index; TS90, Time when blood oxygen saturation was below 90%; MSpO2, mean pulse oxygen saturation; LSpO2, lowest pulse oxygen saturation; ODI, oxygen desaturation index; REM, rapid eye movement; NREM, non-rapid eye movement; HRmax, maximum heart rate; HRmin, minimum heart rate; HRmax-HRmin, maximum heart rate minus minimum heart rate.
To further explore the independent influence of hypoxia parameters in patients with OSAHS, serum NAMPT activity, minimum blood oxygen saturation, average oxygen saturation, TS90, ODI, and other characteristic hypoxia-related parameters in PSG were considered as independent variables, while serum NAMPT activity was considered a dependent variable. Stepwise multivariate linear regression analysis was performed, which revealed that the ODI of patients with OSAHS maybe an independent factor influencing serum NAMPT activity after adjusting for the confounding effects of various factors (β=4.09, P=0.000) (Table 4). This suggested that specific intermittent hypoxia during sleep at night in patients with OSAHS could be the reason for increased serum NAMPT activity.
Table 4
Index | β | 95% CI | P value |
---|---|---|---|
ODI | 4.09 | 2.20 to 5.98 | 0.000 |
OSAHS, obstructive sleep apnea-hypopnea syndrome; NAMPT, nicotinamide phosphoribosyl transferase; ODI, oxygen desaturation index.
Discussion
This study preliminarily evaluated elevated serum NAMPT activity in patients with OSAHS and its effect on the risk of cardiovascular disease. First, we found that compared with healthy controls, serum NAMPT activity was significantly increased in patients with OSAHS and increased with the severity of the disease. Second, correlation and multiple linear regression analyses revealed that changes in blood oxygen levels in patients with OSAHS might be an influencing factor for serum NAMPT levels. Finally, univariate and multiple linear regression analyses showed that serum NAMPT activity and minimum blood oxygen saturation were independently associated with the risk of cardiovascular disease in the OSAHS population; that is, increased serum NAMPT activity or decreased minimum blood oxygen saturation led to higher FRS and higher risk of cardiovascular disease.
Increasing evidence shows that eNAMPT plays a vital role in the occurrence and development of AS (32-35) by mediating foam cell formation, adhesion molecule expression, endothelial dysfunction induction, and vascular wall inflammation induction. As a potential biomarker and therapeutic target, eNAMPT has gained popularity, with various studies (36,37) discussing the effect of NAMPT on AS from a viewpoint of abnormal glucose and lipid metabolism. However, AS caused by OSAHS with CIH as the main pathophysiological basis has a different mechanism from that of classic AS risk factors. Previous epidemiological studies have confirmed that OSAHS is an independent risk factor for AS (26-28,38,39), but the mechanism and therapeutic target remain unclear. Recent studies also report that hypoxia in endothelial cells can lead to upregulation of NAMPT expression, thereby activating the NF-κB and P38/PI3K/AKT signalling pathways and resulting in endothelial function impairment (10,37,40,41), which is the initial stage of AS formation. Therefore, we speculated that NAMPT played a similar role in OSAHS-induced vascular injury. To confirm this hypothesis, the activity of NAMPT in the serum of patients with OASHS was determined, revealing that NAMPT activity increased significantly with severity of the disease. This preliminarily confirmed that the activity of NAMPT was closely related to the occurrence and development of OSAHS and could be involved in a series of pathophysiological changes caused by OSAHS.
Correlation analysis found that hypoxia-related parameters (such as MSpO2, TS90, ODI, and AHI) of patients with OSAHS were significantly correlated with NAMPT activity. Furthermore, multivariate linear regression analysis showed that ODI, an indicator that reflects changes in blood oxygen, was independent associated with serum NAMPT levels, and the more severe the degree of hypoxia in patients with OSAHS at night, the higher the activity of serum NAMPT, which was consistent with previous reports that hypoxia induces increased NAMPT expression and leads to endothelial dysfunction.
Cardiovascular complications are the main cause of death in patients with OSAHS (41-43). Previous cohort studies have shown that minimum blood oxygen saturation, TS90, ODI, and other hypoxia parameters of patients with OSAHS can predict cardiovascular disease risk in this population to a certain extent (44,45). In order to preliminarily explore the correlation between increase in serum NAMPT activity, which is closely related to hypoxia in patients with OSAHS, and the increase in cardiovascular disease incidence in this population, FRS, which is widely used in the prediction and prevention of cardiovascular disease, was used as the risk outcome index (46-49). The activity of serum NAMPT and characteristic parameters of PSG were further studied. The results showed that FRS increased with the severity of OSAHS, suggesting that the higher the severity of OSAHS, the higher the risk of cardiovascular disease. Correlation analysis of serum NAMPT activity and FRS in patients with OSAHS showed that there was a significant positive correlation between NAMPT activity and FRS. Furthermore, univariate and multivariate linear regression analyses revealed that, in addition to OSAHS-related hypoxia, NAMPT activity was found to be independent associated with FRS after adjusting for confounding factors related to cardiovascular diseases, such as blood oxygen, blood lipid, and blood glucose. Therefore, we speculated that CIH could induce increased NAMPT in patients with OSAHS, leading to vascular endothelial cell dysfunction and further increasing cardiovascular disease risk.
However, this study had some limitations. Firstly, the research results were based on single-centre sample data; therefore, further validation using a multicentre large sample is needed to clarify the stability of the research results. Secondly, this study reported a preliminary result based on a cross-sectional clinical study. Further cohort studies and related basic studies are needed to explore the exact impact and specific mechanism of serum NAMPT activity in patients with OSAHS and cardiovascular disease.
In conclusion, this study revealed that serum NAMPT activity was significantly increased in patients with OSAHS and increased with the severity of the disease. Furthermore, serum NAMPT activity had an independent impact on the risk of early cardiovascular disease in patients with OSAHS, indicating the possibility of NAMPT as a potential marker or therapeutic target for cardiovascular disease in patients with OSAHS. In the future, basic studies using a multicentre, large population cohort are needed to confirm these results and further explore the role and mechanism of eNAMPT in cardiovascular disease induced by OSAHS.
Acknowledgments
The authors thank all the participants and research staff of the Departments of Otolaryngology and Clinical Biobank at the First Hospital of Hebei Medical University.
Funding: This research was funded by Hebei Province Natural Science Foundation of China (grant number H2020206472), The Provincial level Science and Technology Program of Hebei Province (S&T Program of Hebei, grant number 21377734D), and Training Programme Foundation for the Talents of Clinical Medicine sponsored by the Government (grant number LS202003).
Footnote
Reporting Checklist: The authors have completed the STROBE reporting checklist. Available at https://apm.amegroups.com/article/view/10.21037/apm-22-975/rc
Data Sharing Statement: Available at https://apm.amegroups.com/article/view/10.21037/apm-22-975/dss
Conflicts of Interest: All authors have completed the ICMJE uniform disclosure form (available at https://apm.amegroups.com/article/view/10.21037/apm-22-975/coif). The authors have no conflicts of interest to declare.
Ethical Statement: The authors are accountable for all aspects of the work in ensuring that questions related to the accuracy or integrity of any part of the work are appropriately investigated and resolved. This study was approved by the Ethics Review Committee of the First Hospital of Hebei Medical University (Ethical Approval No. 20200623) and conducted in accordance with the Declaration of Helsinki (as revised in 2013). All clinical information was obtained from the clinical sample database of the hospital, and all patients gave written informed consent.
Open Access Statement: This is an Open Access article distributed in accordance with the Creative Commons Attribution-NonCommercial-NoDerivs 4.0 International License (CC BY-NC-ND 4.0), which permits the non-commercial replication and distribution of the article with the strict proviso that no changes or edits are made and the original work is properly cited (including links to both the formal publication through the relevant DOI and the license). See: https://creativecommons.org/licenses/by-nc-nd/4.0/.
References
- Dahl TB, Holm S, Aukrust P, et al. Visfatin/NAMPT: a multifaceted molecule with diverse roles in physiology and pathophysiology. Annu Rev Nutr 2012;32:229-43. [Crossref] [PubMed]
- Fukuhara A, Matsuda M, Nishizawa M, et al. Visfatin: a protein secreted by visceral fat that mimics the effects of insulin. Science 2005;307:426-30. [Crossref] [PubMed]
- Chang YH, Chang DM, Lin KC, et al. Visfatin in overweight/obesity, type 2 diabetes mellitus, insulin resistance, metabolic syndrome and cardiovascular diseases: a meta-analysis and systemic review. Diabetes Metab Res Rev 2011;27:515-27. [Crossref] [PubMed]
- Adya R, Tan BK, Punn A, et al. Visfatin induces human endothelial VEGF and MMP-2/9 production via MAPK and PI3K/Akt signalling pathways: novel insights into visfatin-induced angiogenesis. Cardiovasc Res 2008;78:356-65. [Crossref] [PubMed]
- Wang P, Vanhoutte PM, Miao CY. Visfatin and cardio-cerebro-vascular disease. J Cardiovasc Pharmacol 2012;59:1-9. [Crossref] [PubMed]
- Ye SQ, Zhang LQ, Adyshev D, et al. Pre-B-cell-colony-enhancing factor is critically involved in thrombin-induced lung endothelial cell barrier dysregulation. Microvasc Res 2005;70:142-51. [Crossref] [PubMed]
- Adya R, Tan BK, Chen J, et al. Pre-B cell colony enhancing factor (PBEF)/visfatin induces secretion of MCP-1 in human endothelial cells: role in visfatin-induced angiogenesis. Atherosclerosis 2009;205:113-9. [Crossref] [PubMed]
- Friebe D, Neef M, Kratzsch J, et al. Leucocytes are a major source of circulating nicotinamide phosphoribosyltransferase (NAMPT)/pre-B cell colony (PBEF)/visfatin linking obesity and inflammation in humans. Diabetologia 2011;54:1200-11. [Crossref] [PubMed]
- Kutuk O, Basaga H. Inflammation meets oxidation: NF-kappaB as a mediator of initial lesion development in atherosclerosis. Trends Mol Med 2003;9:549-57. [Crossref] [PubMed]
- Lu YB, Chen CX, Huang J, et al. Nicotinamide phosphoribosyltransferase secreted from microglia via exosome during ischemic injury. J Neurochem 2019;150:723-37. [Crossref] [PubMed]
- Schrijvers DM, De Meyer GR, Herman AG, et al. Phagocytosis in atherosclerosis: Molecular mechanisms and implications for plaque progression and stability. Cardiovasc Res 2007;73:470-80. [Crossref] [PubMed]
- Pillai VB, Sundaresan NR, Kim G, et al. Nampt secreted from cardiomyocytes promotes development of cardiac hypertrophy and adverse ventricular remodeling. Am J Physiol Heart Circ Physiol 2013;304:H415-26. [Crossref] [PubMed]
- Tabas I. Macrophage death and defective inflammation resolution in atherosclerosis. Nat Rev Immunol 2010;10:36-46. [Crossref] [PubMed]
- Yu XY, Qiao SB, Guan HS, et al. Effects of visfatin on proliferation and collagen synthesis in rat cardiac fibroblasts. Horm Metab Res 2010;42:507-13. [Crossref] [PubMed]
- Dakroub A, Nasser SA, Kobeissy F, et al. Visfatin: An emerging adipocytokine bridging the gap in the evolution of cardiovascular diseases. J Cell Physiol 2021;236:6282-96. [Crossref] [PubMed]
- Malyszko J, Malyszko JS, Mysliwiec M. Visfatin, a new adipocytokine, is predominantly related to inflammation/endothelial damage in kidney allograft recipients. Transplant Proc 2009;41:150-3. [Crossref] [PubMed]
- Liu SW, Qiao SB, Yuan JS, et al. Visfatin stimulates production of monocyte chemotactic protein-1 and interleukin-6 in human vein umbilical endothelial cells. Horm Metab Res 2009;41:281-6. [Crossref] [PubMed]
- Kim SR, Bae SK, Choi KS, et al. Visfatin promotes angiogenesis by activation of extracellular signal-regulated kinase 1/2. Biochem Biophys Res Commun 2007;357:150-6. [Crossref] [PubMed]
- Çetin N, Güneş Tatar İ, Yüceege M, et al. Ultrasonographic evaluation of abdominal wall fat index, carotid intima-media thickness and plaque score in obstructive sleep apnea syndrome. Med Ultrason 2019;21:422-6. [Crossref] [PubMed]
- Karkoulias K, Lykouras D, Sampsonas F, et al. The role of Endothelin-1 in obstructive sleep apnea syndrome and pulmonary arterial hypertension: pathogenesis and Endothelin-1 antagonists. Curr Med Chem 2010;17:1059-66. [Crossref] [PubMed]
- Javadi HR, Jalilolghadr S, Yazdi Z, et al. Correlation between Obstructive Sleep Apnea Syndrome and Cardiac Disease Severity. Cardiovasc Psychiatry Neurol 2014;2014:631380. [Crossref] [PubMed]
- Song D, Fang G, Greenberg H, et al. Chronic intermittent hypoxia exposure-induced atherosclerosis: a brief review. Immunol Res 2015;63:121-30. [Crossref] [PubMed]
- Arnaud C, Beguin PC, Lantuejoul S, et al. The inflammatory preatherosclerotic remodeling induced by intermittent hypoxia is attenuated by RANTES/CCL5 inhibition. Am J Respir Crit Care Med 2011;184:724-31. [Crossref] [PubMed]
- Jun J, Reinke C, Bedja D, et al. Effect of intermittent hypoxia on atherosclerosis in apolipoprotein E-deficient mice. Atherosclerosis 2010;209:381-6. [Crossref] [PubMed]
- Drager LF, Yao Q, Hernandez KL, et al. Chronic intermittent hypoxia induces atherosclerosis via activation of adipose angiopoietin-like 4. Am J Respir Crit Care Med 2013;188:240-8. [Crossref] [PubMed]
- Yeghiazarians Y, Jneid H, Tietjens JR, et al. Obstructive Sleep Apnea and Cardiovascular Disease: A Scientific Statement From the American Heart Association. Circulation 2021;144:e56-67. [Crossref] [PubMed]
- Yu J, Zhou Z, McEvoy RD, et al. Association of Positive Airway Pressure With Cardiovascular Events and Death in Adults With Sleep Apnea: A Systematic Review and Meta-analysis. JAMA 2017;318:156-66. [Crossref] [PubMed]
- Xie C, Zhu R, Tian Y, et al. Association of obstructive sleep apnea with the risk of vascular outcomes and all-cause mortality: a meta-analysis. BMJ Open 2017;7:e013983. [Crossref] [PubMed]
- Segawa K, Fukuhara A, Hosogai N, et al. Visfatin in adipocytes is upregulated by hypoxia through HIF1alpha-dependent mechanism. Biochem Biophys Res Commun 2006;349:875-82. [Crossref] [PubMed]
- Neubauer K, Bednarz-Misa I, Walecka-Zacharska E, et al. Oversecretion and Overexpression of Nicotinamide Phosphoribosyltransferase/Pre-B Colony-Enhancing Factor/Visfatin in Inflammatory Bowel Disease Reflects the Disease Activity, Severity of Inflammatory Response and Hypoxia. Int J Mol Sci 2019;20:166. [Crossref] [PubMed]
- McPherson R, Frohlich J, Fodor G, et al. Canadian Cardiovascular Society position statement--recommendations for the diagnosis and treatment of dyslipidemia and prevention of cardiovascular disease. Can J Cardiol 2006;22:913-27. [Crossref] [PubMed]
- Bułdak RJ, Bułdak Ł, Polaniak R, et al. Visfatin affects redox adaptative responses and proliferation in Me45 human malignant melanoma cells: an in vitro study. Oncol Rep 2013;29:771-8. [Crossref] [PubMed]
- Lovren F, Pan Y, Shukla PC, et al. Visfatin activates eNOS via Akt and MAP kinases and improves endothelial cell function and angiogenesis in vitro and in vivo: translational implications for atherosclerosis. Am J Physiol Endocrinol Metab 2009;296:E1440-9. [Crossref] [PubMed]
- Hognogi LD, Simiti LV. The cardiovascular impact of visfatin - an inflammation predictor biomarker in metabolic syndrome. Clujul Med 2016;89:322-6. [PubMed]
- Berndt J, Klöting N, Kralisch S, et al. Plasma visfatin concentrations and fat depot-specific mRNA expression in humans. Diabetes 2005;54:2911-6. [Crossref] [PubMed]
- Trakada G, Steiropoulos P, Nena E, et al. Plasma visfatin levels in severe obstructive sleep apnea-hypopnea syndrome. Sleep Breath 2009;13:349-55. [Crossref] [PubMed]
- Adya R, Tan BK, Chen J, et al. Nuclear factor-kappaB induction by visfatin in human vascular endothelial cells: its role in MMP-2/9 production and activation. Diabetes Care 2008;31:758-60. [Crossref] [PubMed]
- Ljunggren M, Lindberg E, Franklin KA, et al. Obstructive sleep apnea during rapid eye movement sleep is associated with early signs of atherosclerosis in women. Sleep 2018; [Crossref] [PubMed]
- Loke YK, Brown JW, Kwok CS, et al. Association of obstructive sleep apnea with risk of serious cardiovascular events: a systematic review and meta-analysis. Circ Cardiovasc Qual Outcomes 2012;5:720-8. [Crossref] [PubMed]
- Kong YY, Li GQ, Zhang WJ, et al. Nicotinamide phosphoribosyltransferase aggravates inflammation and promotes atherosclerosis in ApoE knockout mice. Acta Pharmacol Sin 2019;40:1184-92. [Crossref] [PubMed]
- Yang S, Ryu JH, Oh H, et al. NAMPT (visfatin), a direct target of hypoxia-inducible factor-2α, is an essential catabolic regulator of osteoarthritis. Ann Rheum Dis 2015;74:595-602. [Crossref] [PubMed]
- Li L, Wang L, Liu SS, et al. Association between Coronary Atherosclerotic Plaque Composition and Cardiovascular Disease Risk. Biomed Environ Sci 2019;32:75-86. [PubMed]
- Catalán V, Gómez-Ambrosi J, Rodríguez A, et al. Association of increased visfatin/PBEF/NAMPT circulating concentrations and gene expression levels in peripheral blood cells with lipid metabolism and fatty liver in human morbid obesity. Nutr Metab Cardiovasc Dis 2011;21:245-53. [PubMed]
- Labarca G, Dreyse J, Drake L, et al. Efficacy of continuous positive airway pressure (CPAP) in the prevention of cardiovascular events in patients with obstructive sleep apnea: Systematic review and meta-analysis. Sleep Med Rev 2020;52:101312. [Crossref] [PubMed]
- Malhotra A, Ayappa I, Ayas N, et al. Metrics of sleep apnea severity: beyond the apnea-hypopnea index. Sleep 2021;44:zsab030. [Crossref] [PubMed]
- Stephens JM, Vidal-Puig AJ. An update on visfatin/pre-B cell colony-enhancing factor, an ubiquitously expressed, illusive cytokine that is regulated in obesity. Curr Opin Lipidol 2006;17:128-31. [Crossref] [PubMed]
- Rausch LK, Hofer M, Pramsohler S, et al. Adiponectin, Leptin and Visfatin in Hypoxia and its Effect for Weight Loss in Obesity. Front Endocrinol (Lausanne) 2018;9:615. [Crossref] [PubMed]
- Romacho T, Azcutia V, Vázquez-Bella M, et al. Extracellular PBEF/NAMPT/visfatin activates pro-inflammatory signalling in human vascular smooth muscle cells through nicotinamide phosphoribosyltransferase activity. Diabetologia 2009;52:2455-63. [Crossref] [PubMed]
- Chen MP, Chung FM, Chang DM, et al. Elevated plasma level of visfatin/pre-B cell colony-enhancing factor in patients with type 2 diabetes mellitus. J Clin Endocrinol Metab 2006;91:295-9. [Crossref] [PubMed]
(English Language Editor: A. Muijlwijk)