A study of brain glucose metabolism in obsessive-compulsive disorder patients
Introduction
Obsessive-compulsive disorder (OCD) is a chronic neural psychological condition characterized by clinical manifestations such as obsessive thoughts and compulsive behavior. Patients often have both conscious compulsion and anti-compulsion at the same time. It means some meaningless and even unwilling thoughts or impulses repeatedly invade the patient’s daily life. Although the patients resist them vigorously, they are always uncontrollable. The strong conflicts arising from this makes patients feel great anxiety and pain. Despite drug therapy, cognitive behavior therapy, and surgical treatment, 40% to 60% of patients remain symptomatic and some patients may even develop refractory OCD (1).
The pathogenesis of OCD is not yet completely understood. Some scholars put forward the orbito-frontal gurus-striatum-pallidum-thalamic loop hypothesis (2), and a growing number of studies have found that the orbito-frontal cortex (OFC) plays a key role in the pathogenesis of OCD (3,4).
Increasingly, medical imaging, such as positron emission tomography (PET) imaging, has been applied to explore the abnormal changes in brain function in certain regions of the brain. Through PET examination, we can understand the changes in glucose metabolism of the brain, which always indicates the changes of brain function. This current study investigated the changes in the glucose metabolism of the brain before and after treatment in patients with OCD. Through the study, we hope to get further understanding of the changes in brain function in patients with OCD. Besides, the case group in this study mainly used different drug treatments, including citalopram hydrobromide and quetiapine, from other studies in order to find different results. We present the following article in accordance with the MDAR reporting checklist (available at https://apm.amegroups.com/article/view/10.21037/apm-22-1161/rc).
Methods
Subjects
A total of 23 patients presented to the Third Affiliated Hospital of Sun Yat-sen University with OCD and 10 healthy controls participated in this study. The number of participants enrolled referred to previous relevant studies (2,5). The basic characteristics of the participants are detailed in Table 1. The study was conducted in accordance with the Declaration of Helsinki (as revised in 2013). This study was approved by the Institutional Review Board (IRB) of the Fifth Affiliated Hospital of Sun Yat-sen University prior to patient enrollment and data collection [Ethics Number: 2022(K329-1)]. Patients in the case group and the control group, and/or their legal guardian provided a signed written informed consent under the IRB protocol.
Table 1
Characteristics | OCD1 group (n=10) | OCD2 group (n=13) | Controls (n=10) | P value |
---|---|---|---|---|
Sex, M/F, No. | 6/4 | 8/5 | 5/5 | NA |
Age, y, mean ± SD | 19.1±0.752 | 27.0±2.169 | 24.6±0.581 | 0.004a |
Obsessive thoughts score, mean ± SD | 11.7±2.002 | 10.9±2.840 | NA | NA |
Compulsive behavior score, mean ± SD | 10.3±2.451 | 6.8±4.571 | NA | NA |
HAMD, mean ± SD | 9.3±1.44 | 8.1±1.20 | NA | NA |
a, there was a significant difference in age between three groups, a significant difference was found between the OCD1 group and the control group in post hoc multiple comparisons (P<0.05/3). OCD, obsessive-compulsive disorder; M, male; F, female; HAMD, Hamilton Scale for Depression; SD, standard deviation; NA, not applicable.
Patients were diagnosed with OCD and they scored 16 or above on the Yale Brown Obsessive Compulsive Scale (YBOCS), in accordance with the Diagnostic and Statistical Manual of Getting Disorders, Fifth Edition (DSM-V). If patients had only compulsive thought or only behavior, the YBOCS score must reach 8 or above. Patients with other serious diseases, craniocerebral trauma history, or other concurrent mental illnesses such as major depression [24-item Hamilton Scale for Depression (HAMD) >35 points], were excluded.
In this study, patients were divided into case group 1 and case group 2. The former group consisted of patients who received no drugs or recently stopped drug therapy for more than 5 half-life periods (OCD1 group). Patients in case group 2 received drug treatment ranging from 3 months to 3 years before enrollment (OCD2 group). There were 10 participants in the control group, consisting of hospital staff personnel, students, and volunteers from the surrounding community. The healthy controls had no history of mental illness nor other serious disease.
Treatment
Within the OCD2 group who were on medication (listed in Table 2), 7 patients underwent a repeat PET scan after three months, and these patients were classified as group 3 (OCD3 group). The therapeutic regimens of these 7 patients before and after enrollment are shown in Table 2 and their respective clinical symptoms are shown in Table 3. There was a significant difference in the obsessive thought score before and after treatment; but no differences in compulsive behavior nor HAMD score were found. The remaining 6 patients in the OCD2 group did not adhere to the treatment for the next 3 months or were lost to follow up.
Table 2
Medication | Before enrollment | After enrollment | |||
---|---|---|---|---|---|
No. | Percentage (%) | No. | Percentage (%) | ||
Fluvoxamine | 1 | 14.3 | 0 | 0 | |
Fluoxetine | 1 | 14.3 | 1 | 14.3 | |
Citalopram hydrobromide | 5 | 71.4 | 6 | 85.7 | |
Quetiapine | 6 | 85.7 | 7 | 100 | |
Alprazolam | 3 | 42.9 | 2 | 28.6 | |
Clonazepam | 1 | 14.3 | 2 | 28.6 | |
Sodium valproate | 1 | 14.3 | 1 | 14.3 | |
Lithium carbonate | 1 | 14.3 | 4 | 57.1 |
Table 3
Characteristics | Before enrollment | After medication | Average score-reducing rate (%) | P value |
---|---|---|---|---|
Obsessive thoughts score, mean ± SD | 10.29±1.190 | 6.86±0.892 | 32.03 | 0.009a |
Compulsive behavior score, mean ± SD | 5.29±2.020 | 3.57±1.343 | 30.45 | 0.068 |
HAMD, mean ± SD | 6.43±1.412 | 4.00±0.378 | 23.47 | 0.112 |
a, there was a significant difference in the obsessive thoughts score before enrollment and three months after medication. OCD, obsessive-compulsive disorder; HAMD, Hamilton Scale for Depression; SD, standard deviation.
Imaging
All participants underwent head PET-CT examinations with a GE Discovery Elite imaging machine, using 2-fluoro-2-deoxidation-D-glucose [fluorine-18 fluorodeoxyglucose (18F-FDG); Guangzhou Isotopes Company, Guangzhou, China] as the imaging agent. All subjects in the OCD3 group underwent a second PET scan again after three months of treatment with medication.
Statistical analysis
The Statistical Parametric Mapping (SPM) 8 software (version 6313) was used on the MATLAB software platform (version 7.11.0) to perform image preprocessing, including standardization and smoothing. In the software, the brain atlas space defined by Talairach and Tournoux (6) was used as the basis of the transformation, and standard brain reference images of different imaging methods [such as magnetic resonance imaging (MRI), PET, etc.] were produced. Differences in the brain structure among different subjects were normalized through image standardization using the PET template within SPM. By doing so, we could determine the corresponding brain structure of each pixel of the image according to the spatial coordinates of the standard brain in Talairach space. The image was then smoothed using 8 mm × 8 mm × 8 mm half high width to acquire the target image. After image preprocessing, the SPM software was used to compare the results between the different groups. Image comparison between three groups was performed using variance analysis followed by post-hoc analysis, using age as a covariate, to acquire the pixels that exhibited differences in glucose metabolic between different groups (P<0.005/3). To compare the image of the cases in the OCD3 group, two independent sample t-tests were performed (P<0.005). After this process, the pixel coordinates were acquired and AAL (Anatomical Automatic Labeling) was used to correspond coordinates to the standard brain. Finally, the brain regions that corresponds to the pixels were identified.
At present, there is no image standardization method that can accurately align each groove of a tested brain to another, however, SPM software is the preferred choice among the currently available methods.
Results
Differences in the PET scan between the OCD1 group and the control group
Compared to the control group, we found abnormally enhanced glucose metabolism in the left medium orbito-frontal region of the brain in OCD1 group, and abnormally reduced glucose metabolism in brain areas including the right insula, right caudate nucleus, and left middle temporal gyrus. Table 4 and Figure 1 show differences in the PET scan between participants in the OCD1 group and the control group.
Table 4
Left/right | Encephalic region | X | Y | Z | Z value | P value |
---|---|---|---|---|---|---|
Enhanced glucose metabolism left | Medium orbito-frontal | −34 | 62 | −38 | 3.33 | 0.000 |
Reduced glucose metabolism | ||||||
Right | Insula | 34 | 16 | −18 | 4.32 | 0.000 |
Right | Caudate nucleus | 18 | 10 | 12 | 3.34 | 0.000 |
Left | Middle temporal gyrus | −68 | −50 | 6 | 3.22 | 0.001 |
X, Y, and Z are coordinates. X represents the distance from the brain regions to the median sagittal plane, left of sagittal plane as “+”, right as “−”. Y represents the distance from the brain regions to the anterior commissure, in front of anterior commissure as “+”, behind as “−”. Z represents the distance from the brain regions to the anterior-posterior commissure plane, above the plane as “+”, inferior as “−”. P values <0.005/3 has statistical significance. PET, positron emission tomography; OCD, obsessive-compulsive disorder.
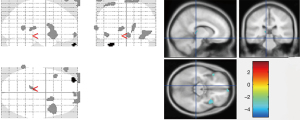
Differences in the PET scans between the OCD2 group and the control group
In this comparison, the abnormally enhanced glucose metabolism was only found in left calcarine cortex region of the brain in OCD2 group. Table 5 and Figure 2 show the differences in the PET scans between the OCD2 group and the control group.
Table 5
Left/right | Encephalic region | X | Y | Z | Z value | P value |
---|---|---|---|---|---|---|
Reduced glucose metabolism: left | Calcarine cortex | −12 | −110 | −4 | 3.15 | 0.001 |
X, Y and Z are coordinates. The establish rules of coordinates are the same as Table 4. P values <0.005/3 has statistical significance. PET, positron emission tomography; OCD, obsessive-compulsive disorder.
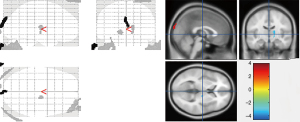
Differences in the PET scans between the OCD1 group and the OCD2 group
Compared to the OCD1 group, we found abnormally enhanced glucose metabolism in right paracentral lobule and right insula region in the OCD2 group, while glucose metabolism was abnormally reduced in the region of left inferior temporal gyrus and right fusiform gyrus. Table 6 and Figure 3 show the differences in the PET scans between the OCD1 group and the OCD2 group.
Table 6
Left/right | Encephalic region | X | Y | Z | Z value | P value |
---|---|---|---|---|---|---|
Enhanced glucose metabolism | ||||||
Right | Paracentral lobule | 6 | −24 | 84 | 3.36 | 0.000 |
Right | Insula | 42 | 12 | −12 | 3.01 | 0.001 |
Reduced glucose metabolism | ||||||
Left | Inferior temporal gyrus | −52 | −28 | −28 | 3.41 | 0.000 |
Right | Fusiform gyrus | 32 | −4 | −34 | 3.29 | 0.001 |
X, Y and Z are coordinates. The establish rules of coordinates are the same as Table 4. P values <0.005/3 has statistical significance. PET, positron emission tomography; OCD, obsessive-compulsive disorder.
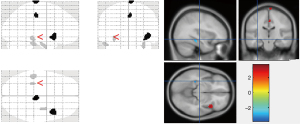
Differences between the PET-CT scans before treatment and after 3 months of treatment in the OCD3 group
After 3 months of drug therapy, we found abnormally enhanced glucose metabolism in left supplementary motor area region, and abnormally reduced glucose metabolism in superior parietal gyrus region. A comparison of the 2 PET-CT scans of the OCD3 group before treatment and at 3 months post-treatment is shown in Table 7 and Figure 4.
Table 7
Left/right | Encephalic region | X | Y | Z | Z value | P value |
---|---|---|---|---|---|---|
Enhanced glucose metabolism: left | Supplementary motor area | −4 | 10 | 60 | 3.08 | 0.001 |
Reduced glucose metabolism: left | Superior parietal gyrus | −26 | −52 | 58 | 2.96 | 0.002 |
X, Y and Z are coordinates. The establish rules of coordinates are the same as Table 4. P values <0.005 has statistical significance. PET, positron emission tomography; CT, computed tomography; OCD, obsessive-compulsive disorder.
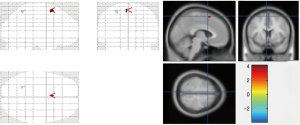
The correlation analysis between the change of brain glucose metabolism and the curative effect
Correlation analysis between the 2 PET scans and the YBOCS score in the OCD3 group suggested that changes in brain glucose metabolism were not related to curative effect.
Discussion
Changes in abnormal glucose metabolism in OCD patients
The orbito-frontal-striatum-thalamic loop theory is a classic doctrine in the pathogenesis of OCD, which believed dysfunction in the loop may play significant role in the occurrence of the disease. However, many studies examining the PET scan results of patients with OCD have shown that abnormities are not only found in the loop, but also in other areas (4,7). Since the neurocognitive functions related to OCD cannot be explained by the loop, the dorsolateral-prefrontal cortex-striatum models have been proposed (8). The latter theory suggests that in addition to orbito-frontal loop dysfunction, the dorsolateral prefrontal cortex and parietal lobe are also involved in the pathogenesis of OCD. Piras et al. (9) conducted a systematic review on a large number of imaging studies and suggested that the mechanism of OCD includes a wide range of brain abnormalities, which would explain the diversity of symptoms and disease severity. In recent years, further studies have confirmed that the frontal cortex plays an important role in OCD, and put forward the cortico-striato-thalamo-cortical (CSTC) loop theory (10,11).
Based on the CSTC loop theory, we herein conducted functional imaging analysis on OCD patients. This study found that patients who received no drugs or recent drug withdrawal showed a moderate increase in glucose metabolism in the orbito-frontal brain (Table 4, Figure 1), and decreased glucose metabolism in the caudate nucleus, which involves the CSTC circuits. These results were consistent with previous research findings (3,5). However, this research also found that the glucose metabolism of the insula and middle temporal gyrus (which are outside the loop) was unusually reduced.
Many publications have suggested that the insula plays an important role in the development of OCD. Berlin et al. (12) found that the anterior insula may play a central role in the emotional modulation of response inhibition in contamination-type OCD. They therefore speculated that the insula may serve as a potential treatment target. A study (13) has shown a positive correlation between the YBOCS of OCD patients and the left anterior insula volume, suggesting that insula abnormalities may be involved in the pathophysiological mechanisms of OCD.
Regarding the reduced glucose metabolism in the middle temporal gyrus, many studies have found that the temporal lobe may be involved in the pathophysiology of OCD. Sanematsu et al. (14) enrolled 17 OCD patients and performed functional MRI before and 12 weeks after fluvoxamine treatment. They found that the left superior temporal gyrus and right cerebellum were related to the improvement of the compulsive symptom scores. The study showed that the above-mentioned brain area may predict the degree of the compulsive symptom improvement. In addition, Rotge et al. (15) found that the dorsolateral prefrontal cortex, precuneus, and superior temporal gyrus can appear obviously activated in patients with OCD.
Taken together, we speculate that abnormal brain function in OCD patients may not only be limited to the usual loop model, but may involve a wide range of brain regions. There is also study suggesting that OCD may involve dysfunction within networks of brain regions (16).
In addition, this study compared brain glucose metabolism between recent drug withdrawal patients and patients under treatment. We found enhanced glucose metabolism in the paracentral lobule and insula, and reduced glucose metabolism in the temporal lobe, and fusiform gyrus of the brain in patients currently under treatment, suggesting that these drugs, including citalopram hydrobromide, quetiapine and other drugs as listed in Table 2, may have a strong effect on brain glucose metabolism.
Patients in the case group, who were under medication before enrollment, also showed reduced glucose metabolism in the calcarine cortex. However, since the glucose metabolism of these patients is not only affected by metabolic abnormalities caused by the disease itself, but also metabolic abnormalities caused by the drugs, we cannot comment further on this.
The relationship between changes in brain glucose metabolism and curative effect
A previous study indicates that there may be two types of brain function changes in OCD patients undergoing treatment. One type is abnormal structural and functional change which gradually returns to normal, and the other type is a compensatory mechanism which gradually improves (17).
In this study, the OCD3 group of patients undergoing drug therapy showed reduced glucose metabolism in the superior parietal gyrus. The superior parietal gyrus belongs to the dorsolateral prefrontal cortex-striatum loop, in which another imaging study has shown abnormalities in OCD patients (18). This result suggested that treatment aimed at the superior parietal gyrus may improve obsessive-compulsive symptoms. In addition, the glucose metabolism of the supplementary motor area in the OCD3 group appeared enhanced, suggesting that the dysfunction of the area may participate in the development of OCD. An increasing number of clinical trials have demonstrated that excessive activation in the supplementary motor area occurs in OCD patients. A meta-analysis of transcranial direct current stimulation (tDCS) in OCD (19) showed that it appears to activate most of the brain regions associated with OCD with cathode over pre-supplemental exercise area. On this basis, some scholars (20) used transcranial direct current stimulation to stimulate the supplementary motor area of refractory OCD patients, and some used repetitive transcranial magnetic stimulation over the same brain region (21). These studies all found significantly improved clinical symptoms, suggesting that excessive activation of certain brain regions may cause OCD symptoms.
However, this current study demonstrated that improved symptoms was associated with increased glucose metabolism in the supplementary motor area. We speculate that this result may be due to incompletely improved symptoms and delayed illness.
Correlation analysis of brain metabolism and efficacy failed to find any association between a brain region and the YBOCS score. However, some previous studies (22,23) examining brain function changes before and after treatment in OCD patients have found a correlation between brain function and changes in symptom scores.
Apostolova et al. (24) found that effective treatment in OCD patients increased the glucose metabolic rate in the local right caudate nucleus, and the degree of the enhanced metabolic rate tended to be associated with the level of symptom improvement. Most studies have suggested that part of the brain function has a role in predicting therapeutic effect. Brody et al.’s (22) research suggested that glucose metabolism in the cingulate gyrus and orbito-frontal is related to an OCD patient’s response to drugs. Patients with lower glucose metabolism can achieve better symptom improvement after drug treatment. Another study has suggested that patients with lower glucose metabolism in the right caudate nucleus have better curative effect (23).
Different studies have used different methods to assess a patient’s curative effect and to draw a comparison of brain function, such as the score-reducing rate after treatment and the change in the glucose metabolic rate index in brain regions. Due to the small sample size in this current study, we only conducted correlation analysis between the brain regions and curative effect, but can’t directly compare brain metabolism of patients who obtained satisfactory efficacy and patients with poor efficacy. This may be why we did not find the brain regions associated with YBOCS score. In addition, the small sample size of the OCD3 group may a significant factor leading to the negative result. Finally, although the process of this study found that the improvement of symptoms was associated with increased glucose metabolism in the superior parietal gyrus, but we did not find any correlation between therapeutic effect and the superior parietal gyrus. It may be due to the reducing slope of the superior parietal gyrus metabolism is not as same as the slope of symptoms improvement.
Glucose metabolism is one of the reflects of brain function, and we cannot totally deny its significant role in the research of OCD. However, it may be not the main cause leading to OCD. Many patients with OCD have dysfunction of serotonin, so in future research, we may try to use contrast agents that track serotonin to conduct more in-depth research of the mechanism. In this study, patients were mainly treated with drugs, including citalopram hydrobromide and quetiapine, and their symptoms improved significantly after 3 months. This may suggest that these two drugs have promising prospect for treatment in OCD.
Acknowledgments
Funding: The study was supported by the Science and Technology Program Project of Guangdong Province (Grant No. 2012B031800283).
Footnote
Reporting Checklist: The authors have completed the MDAR reporting checklist. Available at https://apm.amegroups.com/article/view/10.21037/apm-22-1161/rc
Data Sharing Statement: Available at https://apm.amegroups.com/article/view/10.21037/apm-22-1161/dss
Conflicts of Interest: All authors have completed the ICMJE uniform disclosure form (available at https://apm.amegroups.com/article/view/10.21037/apm-22-1161/coif). All authors report funding support from the Science and Technology Program Project of Guangdong Province (Grant No. 2012B031800283). The authors have no other conflicts of interest to declare.
Ethical Statement: The authors are accountable for all aspects of the work in ensuring that questions related to the accuracy or integrity of any part of the work are appropriately investigated and resolved. The study was conducted in accordance with the Declaration of Helsinki (as revised in 2013). This study was approved by the Institutional Review Board (IRB) of the Fifth Affiliated Hospital of Sun Yat-sen University prior to patient enrollment and data collection [Ethics Number: 2022(K329-1)]. Patients in the case group and the control group, and/or their legal guardian provided a signed written informed consent under the IRB protocol.
Open Access Statement: This is an Open Access article distributed in accordance with the Creative Commons Attribution-NonCommercial-NoDerivs 4.0 International License (CC BY-NC-ND 4.0), which permits the non-commercial replication and distribution of the article with the strict proviso that no changes or edits are made and the original work is properly cited (including links to both the formal publication through the relevant DOI and the license). See: https://creativecommons.org/licenses/by-nc-nd/4.0/.
References
- Husted DS, Shapira NA. A review of the treatment for refractory obsessive-compulsive disorder: from medicine to deep brain stimulation. CNS Spectr 2004;9:833-47. [Crossref] [PubMed]
- Perani D, Colombo C, Bressi S, et al. [18F]FDG PET study in obsessive-compulsive disorder. A clinical/metabolic correlation study after treatment. Br J Psychiatry 1995;166:244-50. [Crossref] [PubMed]
- Baxter LR Jr, Phelps ME, Mazziotta JC, et al. Local cerebral glucose metabolic rates in obsessive-compulsive disorder. A comparison with rates in unipolar depression and in normal controls. Arch Gen Psychiatry 1987;44:211-8. [Crossref] [PubMed]
- Swedo SE, Schapiro MB, Grady CL, et al. Cerebral glucose metabolism in childhood-onset obsessive-compulsive disorder. Arch Gen Psychiatry 1989;46:518-23. [Crossref] [PubMed]
- Millet B, Dondaine T, Reymann JM, et al. Obsessive compulsive disorder networks: Positron emission tomography and neuropsychology provide new insights. PLoS One 2013;8:e53241. [Crossref] [PubMed]
- Talairach J, Tournoux P. Coplanar Stereotaxic Atlas of the Human Brain. M Stuttgart: Thieme Medical; 1988.
- Saxena S, Brody AL, Ho ML, et al. Differential brain metabolic predictors of response to paroxetine in obsessive-compulsive disorder versus major depression. Am J Psychiatry 2003;160:522-32. [Crossref] [PubMed]
- Menzies L, Chamberlain SR, Laird AR, et al. Integrating evidence from neuroimaging and neuropsychological studies of obsessive-compulsive disorder: the orbitofronto-striatal model revisited. Neurosci Biobehav Rev 2008;32:525-49. [Crossref] [PubMed]
- Piras F, Piras F, Chiapponi C, et al. Widespread structural brain changes in OCD: a systematic review of voxel-based morphometry studies. Cortex 2015;62:89-108. [Crossref] [PubMed]
- Ting JT, Feng G. Neurobiology of obsessive-compulsive disorder: insights into neural circuitry dysfunction through mouse genetics. Curr Opin Neurobiol 2011;21:842-8. [Crossref] [PubMed]
- Milad MR, Rauch SL. Obsessive-compulsive disorder: beyond segregated cortico-striatal pathways. Trends Cogn Sci 2012;16:43-51. [Crossref] [PubMed]
- Berlin HA, Schulz KP, Zhang S, et al. Neural correlates of emotional response inhibition in obsessive-compulsive disorder: A preliminary study. Psychiatry Res 2015;234:259-64. [Crossref] [PubMed]
- Tang W, Huang X, Li B, et al. Structural brain abnormalities correlate with clinical features in patients with drug-naïve OCD: A DARTEL-enhanced voxel-based morphometry study. Behav Brain Res 2015;294:72-80. [Crossref] [PubMed]
- Sanematsu H, Nakao T, Yoshiura T, et al. Predictors of treatment response to fluvoxamine in obsessive-compulsive disorder: an fMRI study. J Psychiatr Res 2010;44:193-200. [Crossref] [PubMed]
- Rotge JY, Guehl D, Dilharreguy B, et al. Provocation of obsessive-compulsive symptoms: a quantitative voxel-based meta-analysis of functional neuroimaging studies. J Psychiatry Neurosci 2008;33:405-12. [PubMed]
- Goodman WK, Storch EA, Sheth SA. Harmonizing the Neurobiology and Treatment of Obsessive-Compulsive Disorder. Am J Psychiatry 2021;178:17-29. [Crossref] [PubMed]
- van den Heuvel OA, van Wingen G, Soriano-Mas C, et al. Brain circuitry of compulsivity. Eur Neuropsychopharmacol 2016;26:810-27. [Crossref] [PubMed]
- Peng Z, Xu C, Ma N, et al. White Matter Alterations of the Goal-Directed System in Patients With Obsessive-Compulsive Disorder and Their Unaffected First-Degree Relatives. Biol Psychiatry Cogn Neurosci Neuroimaging 2021;6:992-1001. [Crossref] [PubMed]
- Senço NM, Huang Y, D'Urso G, et al. Transcranial direct current stimulation in obsessive-compulsive disorder: emerging clinical evidence and considerations for optimal montage of electrodes. Expert Rev Med Devices 2015;12:381-91. [Crossref] [PubMed]
- D'Urso G, Brunoni AR, Anastasia A, et al. Polarity-dependent effects of transcranial direct current stimulation in obsessive-compulsive disorder. Neurocase 2016;22:60-4. [Crossref] [PubMed]
- Akbarzadeh F, Mohammadzadeh A, Ghoshuni M, et al. Efficacy of repetitive transcranial magnetic stimulation in patients with obsessive-compulsive disorder: a pilot study. Neuropsychiatr 2021;35:192-8. [Crossref] [PubMed]
- Brody AL, Saxena S, Schwartz JM, et al. FDG-PET predictors of response to behavioral therapy and pharmacotherapy in obsessive compulsive disorder. Psychiatry Res 1998;84:1-6. [Crossref] [PubMed]
- Baxter LR Jr, Schwartz JM, Bergman KS, et al. Caudate glucose metabolic rate changes with both drug and behavior therapy for obsessive-compulsive disorder. Arch Gen Psychiatry 1992;49:681-9. [Crossref] [PubMed]
- Apostolova I, Block S, Buchert R, et al. Effects of behavioral therapy or pharmacotherapy on brain glucose metabolism in subjects with obsessive-compulsive disorder as assessed by brain FDG PET. Psychiatry Res 2010;184:105-16. [Crossref] [PubMed]
(English Language Editor: J. Teoh)