Lung ultrasound-guided treatment for acute respiratory distress syndrome in a critically ill patient with severe COVID-19: a case report
Highlight box
Key findings
• LUS can help diagnose ARDS and guide its treatment, which accuracy and portability are better than CT, so it can be used repeatedly in special environments such as the COVID-19 ward.
What is known and what is new?
• The role of LUS in the diagnosis and treatment of ARDS is very important;
• LUS can be well used in the COVID-19 ward.
What is the implication, and what should change now?
• To optimize the homogenized management of LUS, which can make LUS more accurate in the diagnosis and treatment of ARDS, thus to improve the prognosis of patients.
Introduction
Severe acute respiratory syndrome coronavirus 2 (SARS-CoV-2) belongs to the β-type of coronaviruses. Several patients have been continuously affected by this viral disease in Wuhan City, Hubei Province, China, since December 2019 (1). Omicron is the latest strain. The source of infection that has been seen thus far is close contact with patients infected with this disease, including those with asymptomatic infection. The virus is mainly transmitted through infected droplets and close contact with infected people. The population is generally vulnerable. There are no specific clinical manifestations after infection, with most of those infected showing symptoms similar to those of the common viral pneumonia, such as fever, cough, muscle soreness, and so on. The diagnosis of the disease is mainly made using throat swab results and imaging findings. The main features of computed tomography (CT) imaging (2) are early multiple small patchy shadows and interstitial changes, evident in the extrapulmonary zone, multiple ground glass infiltrations and infiltrates in both lungs, and pulmonary consolidation in severe cases. Pleural effusions are infrequent. Clinical classification is divided into mild, typical, severe, and critical. Those meeting one of the following conditions are considered critically ill: (I) respiratory failure that requires mechanical ventilation; (II) shock; (III) organ failure or requiring intensive care unit (ICU) monitoring and treatment. Severe and critical illness can quickly progress to dyspnea and respiratory failure. LUS is handy, non-radioactive and basically free from environmental restrictions, which is commonly used in acute and critical environment. Acute respiratory distress syndrome (ARDS) is usually characterized by pulmonary edema, lung consolidation and pleural effusion. The ultrasonographic findings of ARDS pulmonary edema were earlier than chest radiographs, the main manifestations are diffuse comet tail signs. LUS can also measure pleural effusion. In addition, lung consolidation caused by ARDS often requires lung reexpansion or prone ventilation. Through the changes of B-line, consolidation and other signs, lung ultrasound (LUS) plays a very important role in the evaluation of lung reexpansion and prone ventilation, so as to avoid the complications. The manifestations of respiratory failure and lung injury caused by coronavirus disease 2019 (COVID-19) are similar to those of ARDS, and may also progress to ARDS. Therefore, pulmonary ultrasound plays an important role in the treatment of COVID-19. We present the following article in accordance with the CARE reporting checklist (available at https://apm.amegroups.com/article/view/10.21037/apm-22-1319/rc).
Case presentation
Chief complaints
An 82-year-old male patient was admitted to a local hospital on 30 January 2020 due to worsening cough, expectoration, and fever of 38 ℃ for 3 days. His symptoms were noted to have been progressively worsening after light activity and he now required long-term oxygen therapy for his daily life activities. At the local hospital, he was treated with piperacillin-tazobactam and ambroxol, but his symptoms showed no improvement. A throat swab was sent for the nucleic acid detection of SARS-CoV-2, which tested positive on the following day. These results, combined with the CT scan findings, confirmed the diagnosis of coronavirus disease of 2019 (COVID-19). The patient was then transferred to the Department of Intensive Care Medicine, Affiliated Jinhua Hospital, Zhejiang University School of Medicine for further treatment. All procedures performed in this study were in accordance with the ethical standards of the institutional and/or national research committee(s) and with the Declaration of Helsinki (as revised in 2013). Written informed consent was obtained from the patient for publication of this case report and accompanying images. A copy of the written consent is available for review by the editorial office of this journal.
History of illness
He had a history of asthma and chronic obstructive pulmonary disease (COPD) for more than 10 years, with the cough and expectoration always worsening during the winter and spring seasons.
Physical examination
On examination, the patient was consciousness but in poor spirits. He had shortness of breath, slightly pursed lips, and a barrel chest. His temperature was 36.5 ℃, blood pressure 170/84 mmHg, and oxygen saturation (SpO2) 78%. In both lungs, wet and dry rales could be heard. Arrhythmia and proiosystole were also identified. Both lower limbs did not have edema.
Laboratory examinations
The blood tests showed the following results: C-reactive protein (CRP) 20.1 mg/L; white blood cells 7.39×109/L; neutrophils (N) 0.926; absolute lymphocyte count 0.27×109/L; hemoglobin 155 g/L; and platelets 112×109/L.
Imaging examinations
Chest CT showed chronic bronchitis, chronic right lower lung infection and emphysema, and bullae of the left upper lung.
Final diagnosis
A diagnosis of (I) new coronavirus (SARS-CoV-2) infected pneumonia (critical illness); (II) respiratory failure; and (III) acute exacerbation of COPD was made.
Treatment
The patient was put on high-flow nasal oxygen (flow of 60 L/min and oxygen concentration of 100%) and treated with oseltamivir (75 mg, nasal feeding, twice daily), lopinavir/ritonavir (3 tablets, nasal feeding, twice daily), abidol (2 tables, nasal feeding, 3 times daily), and cefoperazone-sulbactam (2 g, intravenously, 3 times daily). His blood gas results were as follows: lactic acid (Lac) 1.8 mmol/L, PH (potential of hydrogen) 7.46, partial pressure of carbon dioxide (PCO2) 32.5 mmHg, partial pressure of oxygen (PO2) 61.7 mmHg, oxygen saturation (SO2) 91.8%, PO2/fraction of inspired oxygen (FiO2) (oxygen index) 62. On the morning of the following day (31 January 2020), the patient was placed on non-invasive mechanical ventilation. In the afternoon, the patient began resisting the machine. We reverted back to the high-flow oxygen, but the patient started desaturating, which led us to intubate the patient using invasive mechanical ventilation that evening (ventilator parameters: mode pressure control ventilation, pressure control 12 cmH2O, positive end expiratory pressure 6 cmH2O, respiratory rate times per minute, oxygen concentration 60%). LUS was performed immediately after intubation, which showed the following: an enhanced pleural line echo at each scan point; weak or non-existent pleural sliding sign; no A-line; B-lines at each scanning point, which increased in amount with gravity distribution; fragment sign near the pleural line at the upper blue point, lower blue point, and posterior blue point of the left lung; and the curtain sign at both diaphragmatic points. His blood gas results were as follows: Lac 3.1 mmol/L, PH 7.46, PCO2 32.6 mmHg, PO2 50.3 mmHg, SO2 83.9%, and PO2/FiO2 50. Lung recruitment could have been effective, but we could not maintain oxygenation for a prolonged period. On 1 February 2020, we performed prone position ventilation. The LUS revealed that there were huge amounts of B-lines at the posterior blue point on each side. After 9 h, his blood gases were reviewed, which revealed that Lac was 2.5 mmol/L, PH 7.37, PCO2 42.3 mmHg, PO2 50.5 mmHg, and SO2 84.4%. The LUS showed that B-lines had decreased at the left posterior blue point, had disappeared at the right posterior blue point, and had increased at the posterolateral alveolar and/or pleural syndrome (PLAPS) point of each side. After 16 h, his blood gas levels were as follows: Lac 2.0 mmol/L, PH 7.40, PCO2 39.3 mmHg, PO2 70.4 mmHg, and SO2 92.7%. The LUS showed that the B-lines had disappeared at the left and right posterior blue points. The fragment sign could be seen at the left upper blue points. When returned to supine position, we spotted that the B-lines had increased at the upper blue point, lower blue point, and at the PLAPS point in both the left and right lungs. The side effects of lopinavir and Abidor were mildly, and there were no symptoms such as nausea, vomiting and diarrhea, so the nutritional administration was smoothly during the treatment. In addition, the inflammatory markers were stable, and the antibiotics did not escalate.
Outcome and follow-up
Following these treatments, the whole condition was gradually relieved, and prone position ventilation was stopped on 16th hour of admission. After 2 days, the patient was transferred to the First Hospital of Zhejiang province for further treatment. Timeline is shown in Table 1.
Table 1
Date | Event |
---|---|
1.27.2020 | Presented with cough, expectoration, and fever |
1.27.2020 | Admission to local hospital |
1.27.2020 | Treated with piperacillin-tazobactam and ambroxol |
1.28.2020 | Nucleic acid detection of SARS-CoV-2 was tested positive |
1.30.2020 | Admission to our hospital |
1.31.2020 | High-flow & NIV, intubation |
2.1.2020 | Prone position ventilation |
2.3.2020 | Transferred to the First Hospital of Zhejiang province for further treatment |
SARS-CoV-2, severe acute respiratory syndrome coronavirus 2; NIV, non-invasive ventilation.
Discussion
Bedside LUS is convenient, economical, non-invasive, dynamic, real time, and repeatable. Cortellaro et al. (3) reported that the sensitivity of chest X-rays (CXR) and bedside ultrasound for the diagnosis of pneumonia is 69% and 96%, respectively, showing a significantly higher sensitivity for ultrasound. Parlamento et al. (4) reported sensitivities of 75% and 96.9% for these techniques, respectively, to diagnose pneumonia. Thus, the value of ultrasound for the diagnosis of pneumonia is better than that of CXR.
The condition of the patient we present herein advanced from severe to critical soon after admission, before progressing to ARDS (5). During this time, the assessment of the lung condition was very important for the evaluation of the effect of treatment and to guide further treatment, regardless of diagnosis. Chest radiographs and CT scan are commonly used in assessing the lung condition. These methods are, however, difficult to perform and difficult to repeat for patients with ARDS, given that they need to be in an isolation ward and are critically ill. Therefore, LUS is the best choice. The use of ultrasound as a diagnostic tool for lung conditions dates back to the 1960s (6), but it has not been used widely in intensive care settings until recently. The lung is composed of both gases and liquids. The gas rises and the liquid sinks. Ultrasound completely reflects the normal lung tissue, producing artifacts such as A-lines or a small number of B-lines. The proportion of fluid changes and different types of phenomena appear when ultrasound detects different degrees of deaeration of lung tissue. It is these artifacts, such as fragment sign, tissue-like sign, and more than a dozen other signs, that provide us with evidence (7,8). Therefore, bedside LUS can be used as a new tool for the diagnosis of ARDS in patients with COVID-19 (9-11).
In patients with ARDS, the presence, severity, or timing of ARDS is not an independent risk factor for short-term death. However, ARDS does significantly contribute to 2-year mortality (12). This suggests that even if the patient does not die from ARDS itself, they could die from other primary diseases. Therefore, in the acute phase of ARDS, clinicians should strive to improve treatment strategies for the primary disease that caused ARDS.
The patient had been diagnosed with COPD and hypertension many years before his current admission. Circulatory failure occurred following tracheal intubation, and norepinephrine was administered to maintain blood pressure. At this point, the reason for the occurrence of shock had to be found immediately. The fluid administration limited by lung sonography (FALLS) protocol (7,13) is a method of integrating cardiac ultrasound based on the blue or blue-plus protocol, as described by Lichtenstein, for the identification of shock causes, which is classified by hemodynamic reasons. As the patient had a barrel-shaped chest with significant weight loss, the cardiac sections could not be easily obtained. Judging from the available subxiphoid 4-chamber cardiac section and inferior vena cava section, the heartbeat was normal, the ejection fraction (EF) was about 30–50% (Figure 1), and there was no severe cardiogenic pulmonary edema. Respiratory variation could be measured in the inferior vena cava, and its value was 20% (Figure 2). We considered that the patient had fluid responsiveness, as the respiratory variation of the inferior vena cava was greater than 18% during mechanical ventilation. We temporarily ruled out obstructive shock due to the comprehensive assessment of the size ratio of the 4 chambers of the heart and the variation of the inferior vena cava. Other sections of the heart could not be assessed, and invasive hemodynamic monitoring, such as pulse index continuous cardiac output (PiCCO) and the Swan-Ganz catheter, was unavailable in the isolation wards. Hence, the cardiac output could not be accurately measured, and septic shock could not be completely ruled out. In summary, we believed that the patient’s cardiac function was acceptable and that there was volume responsiveness, but there were B-lines in multiple parts of the lungs indicated by LUS (Figure 3). To avoid the possibility of aggravating pulmonary edema due to fluid administration, we used colloidal fluids. We later discovered that when the amount of colloid had reached about 600 mL, blood pressure improved and norepinephrine administration was gradually stopped. After the blood pressure was stable, colloidal fluids were continued for resuscitation. We had hoped to improve the condition of lung edema by using diuretics, but this approach was unsuccessful.
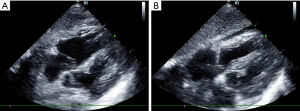
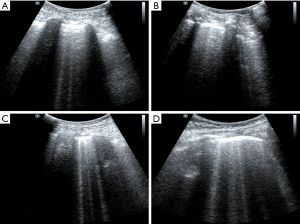
It was difficult to establish whether ARDS was the main effect that had led to the lung edema. It is difficult to distinguish ARDS from acute cardiogenic pulmonary edema by only relying on the B-lines (14). In this case, by this point, the causes of shock had been identified using the FALLS protocol and dealt with accordingly. The features of ARDS on the ultrasound were consistent with what was expected including the following characteristics: consolidation of the anterior wall of the subpleura; weakened or disappeared sliding pleural sign; the presence of normal lung parenchyma; irregular thickening of the pleural line; and asymmetrical B-line distribution. These findings suggested that the pathophysiology of ARDS showed heterogeneous changes in the lungs. Given the poor SpO2 and the improved circulation, prone position ventilation was performed as the next step. Guérin et al. (15) reported that in patients with severe ARDS, early implemented prone position ventilation can significantly reduce mortality at 28 and 90 days. Immediately after the patient was in the prone position (16), LUS was performed. Both left and right posterior blue points showed B-lines, with the left side being more obvious and heterogeneous than the right side (Figure 4). This was also consistent with the characteristics of ARDS pathophysiological changes. After 9 h, we repeated the LUS. The B-lines were still showing on the left posterior blue point but they were less than before. There were no B-lines on the right posterior blue point (Figure 5), but they were present on both sides of the PLAPS points, in greater number than before (Figure 6). At this time, the oxygenation situation had improved from about 60% to 100%, and the SpO2 had been maintained between 88% and 92%, which suggested that the lung tissue in the gravity-dependent parts was involved. We performed LUS again, after 16 h, in the prone position. B-lines were not observed on both posterior blue points and light fragment sign could be seen on the left posterior blue point (Figure 7). After the patient was placed into the supine position again, many B-lines were visible on the upper blue point, lower blue point, and PLAPS points on both sides (Figures 8,9). The most basic pathophysiological change of ARDS is damage to the alveolar capillaries, which increases the permeability of the alveolar-capillary and decreases the effective lung volume due to the large amount of exudate. These injuries are significantly gravity dependent (17). Monitored by LUS, changes we observed were consistent with these pathophysiological changes of ARDS. The uneven distribution of the B-line and the consolidation of the lungs under the pleura also confirmed that the damaged and collapsed lung tissue could be involved. The changes in B-lines and oxygenation at different periods after prone ventilation also showed that the patient’s damaged and collapsed lung tissue had expandability, which provided direction for further treatment.
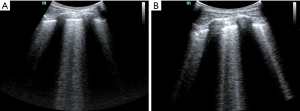
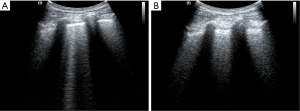
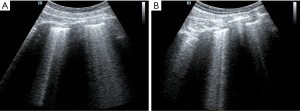
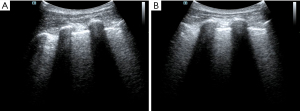
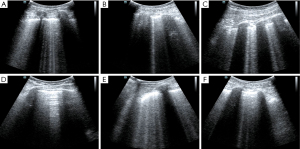
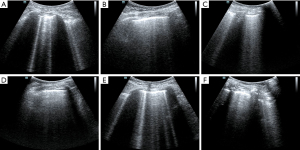
As mentioned above, the lung injury and exudative changes in ARDS are gravity dependent. After 16 h of prone position ventilation, the number of B-lines significantly increased in the lung tissue near the anterior chest wall. Changes in prone position ventilation suggested that the injured lung tissue had good expandability. Therefore, after returning to the supine position, we performed a ventilation strategy for this patient with small tidal volume ventilation and intermittent lung retention. We provided a tidal volume of about 6 mL/kg and tested lung retention every half an hour. About 2 h later, the B-line at the upper and lower blue points had decreased significantly.
The fundamental purpose of various treatment schemes for patients with ARDS is to improve oxygenation while minimizing further lung injury. However, patients with ARDS often have combined problems such as shock and multiple organ failure. In addition to correcting oxygenation, we also need to solve problems such as circulation and volume status. We used the FALLS protocol, combined with pulmonary and cardiac ultrasound, to clarify the cause of the hemodynamic instability and to lay the foundation for further prone position ventilation. Before and after prone position ventilation, the recoverability of the lung was reflected by the bedside lung ultrasound in emergency (BLUE) protocol more intuitively, comparing the parameters of oxygenation and circulation. In summary, the timing of high-frequency oscillating ventilation and implementation of extracorporeal membrane oxygenation (ECMO) should be more accurate.
Conclusions
The fundamental purpose of various treatment schemes for patients with ARDS is to improve oxygenation while minimizing further lung injury. However, patients with ARDS often have combined problems such as shock and multiple organ failure. In addition to correcting oxygenation, we also need to solve problems such as circulation and volume status. We used the FALLS protocol, combined with pulmonary and cardiac ultrasound, to clarify the cause of the hemodynamic instability and to lay the foundation for further prone position ventilation. Before and after prone position ventilation, the recoverability of the lung was reflected by the BLUE protocol more intuitively, comparing the parameters of oxygenation and circulation.
Acknowledgments
Funding: None.
Footnote
Reporting Checklist: The authors have completed the CARE reporting checklist. Available at https://apm.amegroups.com/article/view/10.21037/apm-22-1319/rc
Conflicts of Interest: All authors have completed the ICMJE uniform disclosure form (available at https://apm.amegroups.com/article/view/10.21037/apm-22-1319/coif). The authors have no conflicts of interest to declare.
Ethical Statement: The authors are accountable for all aspects of the work in ensuring that questions related to the accuracy or integrity of any part of the work are appropriately investigated and resolved. All procedures performed in this study were in accordance with the ethical standards of the institutional and/or national research committee(s) and with the Declaration of Helsinki (as revised in 2013). Written informed consent was obtained from the patient for publication of this case report and accompanying images. A copy of the written consent is available for review by the editorial office of this journal.
Open Access Statement: This is an Open Access article distributed in accordance with the Creative Commons Attribution-NonCommercial-NoDerivs 4.0 International License (CC BY-NC-ND 4.0), which permits the non-commercial replication and distribution of the article with the strict proviso that no changes or edits are made and the original work is properly cited (including links to both the formal publication through the relevant DOI and the license). See: https://creativecommons.org/licenses/by-nc-nd/4.0/.
References
- Carlos WG, Dela Cruz CS, Cao B, et al. Novel Wuhan (2019-nCoV) Coronavirus. Am J Respir Crit Care Med 2020;201:7-8. [Crossref] [PubMed]
- Lei J, Li J, Li X, et al. CT Imaging of the 2019 Novel Coronavirus (2019-nCoV) Pneumonia. Radiology 2020;295:18. [Crossref] [PubMed]
- Cortellaro F, Colombo S, Coen D, et al. Lung ultrasound is an accurate diagnostic tool for the diagnosis of pneumonia in the emergency department. Emerg Med J 2012;29:19-23. [Crossref] [PubMed]
- Parlamento S, Copetti R, Di Bartolomeo S. Evaluation of lung ultrasound for the diagnosis of pneumonia in the ED. Am J Emerg Med 2009;27:379-84. [Crossref] [PubMed]
- Solomon JJ, Heyman B, Ko JP, et al. CT of Post-Acute Lung Complications of COVID-19. Radiology 2021;301:E383-95. [Crossref] [PubMed]
- Weil MH, Shubin H. Proposed reclassification of shock states with special reference to distributive defects. Adv Exp Med Biol 1971;23:13-23. [Crossref] [PubMed]
- Lichtenstein D. Lung ultrasound in the critically ill. Curr Opin Crit Care 2014;20:315-22. [Crossref] [PubMed]
- Pesenti A, Musch G, Lichtenstein D, et al. Imaging in acute respiratory distress syndrome. Intensive Care Med 2016;42:686-98. [Crossref] [PubMed]
- Kumar A, Weng Y, Graglia S, et al. Interobserver Agreement of Lung Ultrasound Findings of COVID-19. J Ultrasound Med 2021;40:2369-76. [Crossref] [PubMed]
- Peixoto AO, Costa RM, Uzun R, et al. Applicability of lung ultrasound in COVID-19 diagnosis and evaluation of the disease progression: A systematic review. Pulmonology 2021;27:529-62. [Crossref] [PubMed]
- Villén Villegas T. Lung ultrasound in COVID-19: What has it contributedand what can it contribute? Emergencias 2021;33:331-2. [PubMed]
- Fuchs L, Feng M, Novack V, et al. The Effect of ARDS on Survival: Do Patients Die From ARDS or With ARDS? J Intensive Care Med 2019;34:374-82. [Crossref] [PubMed]
- Lichtenstein DA. BLUE-protocol and FALLS-protocol: two applications of lung ultrasound in the critically ill. Chest 2015;147:1659-70. [Crossref] [PubMed]
- Seiler C, Klingberg C, Hårdstedt M. Lung Ultrasound for Identification of Patients Requiring Invasive Mechanical Ventilation in COVID-19. J Ultrasound Med 2021;40:2339-51. [Crossref] [PubMed]
- Guérin C, Reignier J, Richard JC, et al. Prone positioning in severe acute respiratory distress syndrome. N Engl J Med 2013;368:2159-68. [Crossref] [PubMed]
- González-Seguel F, Pinto-Concha JJ, Aranis N, et al. Adverse Events of Prone Positioning in Mechanically Ventilated Adults With ARDS. Respir Care 2021;66:1898-911. [Crossref] [PubMed]
- Fan E, Brodie D, Slutsky AS. Acute Respiratory Distress Syndrome: Advances in Diagnosis and Treatment. JAMA 2018;319:698-710. [Crossref] [PubMed]
(English Language Editor: J. Jones)