Emergent radiotherapy for spinal cord compression/impingement—a narrative review※
Introduction
Background
Malignant epidural spinal cord compression (MESCC) is a devastating consequence of disease progression. An estimate of 3–5% of all cancer patients develop MESCC, which translates to about 20,000–30,000 yearly cases in the US (1-3). The incidence peaks at age 40–60 and commonly affects patients with breast, prostate, lung, myeloma, kidney, melanoma, and lymphoma (1,4-7). Patients most often present with back pain and commonly have motor/sensory deficits (4). Due to volume of bone and blood flow, about 70% of MESCC cases involve the thoracic spine and about 15% each involve the cervical or lumbosacral spine (4). In about 17–32% of cases, there is multilevel involvement (4,8,9). Treatment for MESCC typically involves a combination of medical management (e.g., pain medication and steroids), surgical decompression, and radiation therapy (RT).
Historically, patients with MESCC underwent laminectomy alone. Following the advent of RT, patients were treated with surgery alone or surgery followed by RT. By the 1970s, studies showed patients treated with RT alone had similar clinical outcomes to those treated with laminectomy followed by RT (4,10). Thus, RT became the definitive therapy for MESCC, until the surgical approach was modified. In 2005, Patchell et al. showed that circumferential surgical decompression ± stabilization followed by RT was superior to RT alone (11). Since then, additional studies have analyzed various conventional RT fractionation schemes for emergent treatment of MESCC, and spinal stereotactic body radiation therapy (SBRT) has been increasingly utilized in the non-emergent setting (12-19). With the evolution and availability of different treatment approaches, determining the optimal therapy for a patient may be challenging. Personalizing treatment of MESCC to each patient is paramount as outcomes (e.g., ambulation) and prognosis can be variable.
Objectives
- Review the initial work-up and medical management of MESCC;
- Discuss the emergent use of RT for MESCC and when primary surgery, primary systemic therapy, or definitive RT may be favored;
- Discuss various doses and fractionations of RT and when each may be favored;
- Discuss the non-emergent use of SBRT for MESCC;
- Comment on future areas of research for MESCC.
We present this article in accordance with the Narrative Review reporting checklist (available at https://apm.amegroups.com/article/view/10.21037/apm-23-342/rc).
Methods
A PubMed database search was conducted on March 24, 2023 using the terms “spinal cord compression” and “radiation therapy” for all published articles in the English language with full text available (Table 1). The search yielded 1,672 results, of which 103 were clinical trials. After manually filtering for prospective trials involving RT for MESCC, 44 remained, and a total of 29 incorporated conventional radiation. These articles were analyzed and supplemented with an additional trial listed in the references, review papers, and well-designed retrospective studies for the purpose of this narrative review. In areas where there was limited data, discussion consisted of expert opinion based on the authors’ clinical experiences.
Table 1
Items | Specification |
---|---|
Date of search | March 2023 |
Databases and other sources searched | PubMed |
Search terms used | Combinations of keywords such as, but not limited to spinal cord compression and radiation therapy |
Timeframe | All publication years considered |
Inclusion criteria | All English language full manuscripts and abstracts were eligible for consideration; preference was given to prospective trials (when applicable) |
Selection process | Initial selection was conducted by P Zaki and SS Lo, supplemented by AG Amin and A Sahgal, and approved by all authors |
Primary work up and medical management
If MESCC is clinically suspected, full neurologic examination and complete spine imaging are recommended. While computed tomography (CT) depicts bony anatomy well and may be completed first due to logistics, magnetic resonance imaging (MRI) is recommended in addition to CT of the spine due to better soft tissue delineation which helps detect MESCC and characterize the degree of compression. A CT myelogram can be used for patients who cannot undergo an MRI spine and/or if there is significant hardware artifact on MRI. An MRI brain is not routinely obtained but may be recommended depending on the case, symptoms, or neurologic exam. The extent of spinal compression is commonly described using a 6-point scale developed by Bilsky et al. in 2010 (20). As depicted in Figure 1, bone-only disease is described as grade 0, epidural impingement as grade 1a, deformation of thecal sac as grade 1b, spinal cord abutment as grade 1c, spinal cord compression as grade 2, and spinal cord compression without visible cerebrospinal fluid as grade 3 (20). In general, grade ≥2 is considered high grade. The radiographic grade of compression as well as clinical symptoms suggest the level of urgency for treatment. Although a focused MRI on just the spine level of interest would be faster to obtain, an MRI of the full spine is generally recommended since MESCC can affect multiple levels (4,8,9) and symptoms could be localized to different sites of spinal compression.
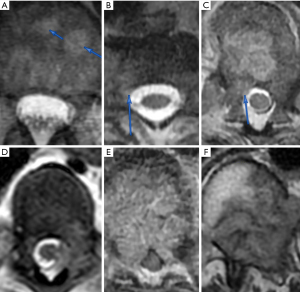
If the patient has significant MESCC symptoms (e.g., pain, urinary/bowel continence/retention, and/or weakness) then steroids should be promptly initiated to help improve symptoms (21) as long as infectious etiology is unlikely or ruled out. If the patient does not have symptoms related to MESCC, then steroids may be reasonably omitted (22). The optimal dexamethasone steroid dose is unclear as there is limited data, but the available research shows that 16 mg total daily dose has similar effectiveness to 96–100 mg and less side effects (23-26). The typical dexamethasone steroid regimen consists of 10 mg IV initial dose followed by 4 mg IV or PO every 6 hours and tapered according to the patient’s response. Although less studied in MESCC, 8 mg twice per day may be a reasonable alternative to 4 mg every 6 hours, based on the half-life of dexamethasone, in an effort to minimize disruption in the patient’s sleep/wake cycle. If present, acute pain should be adequately managed medically (with the assistance of pain specialists if needed) while still allowing the patient to provide informed consent for procedures if recommended. Staging scans (e.g., CT of the chest, abdomen, and pelvis) should be performed, if not done recently, to confirm a primary malignancy and/or the extent of metastasis as this information would help guide a biopsy if needed or the next steps in management. Additional preoperative workup may include deep venous thrombosis (DVT) screening in high-risk patients, as 24% of non-ambulatory patients with spine metastasis have been shown to have a DVT (27). Spine surgery (e.g., neurosurgery or orthopedic surgery), radiation oncology, and medical oncology should be consulted. Management of these complex cases involves a multidisciplinary approach considering the neurological status, radiosensitivity of the pathology, mechanical spinal instability, overall systemic disease burden (28), prognosis, and performance status. Depending on various factors, primary treatment usually consists of either surgery followed by RT or RT alone. Thereafter, systemic therapy may be given if indicated to help provide overall disease control. Less commonly, systemic therapy may be the primary treatment modality. Physical medicine and rehabilitation should be consulted to help optimize patient functionality and independence (29,30). As many MESCC patients harbor life-limiting illness, a palliative care consult should also be considered to help ensure treatment recommendations align with patient goals and values.
Primary surgery followed by RT or primary RT
Since the pivotal randomized control trial by Patchell et al., surgical decompression followed by RT is recommended when patients have an expected survival of at least 3 months (11,31). Patchell et al. showed circumferential surgical decompression ± stabilization followed by RT had significantly better rates of maintained/improved strength (P=0.0064), maintained/regained function (P=0.0008), maintained urinary continence (P=0.016), steroid use (P=0.0093), morphine use (P=0.002), maintained ambulation (P=0.024), regained ambulation (P=0.012), and survival (P=0.033) compared to RT alone (11). On intention-to-treat analysis, 84% of patients in the surgery + RT group could ambulate following treatment compared to 57% of patients in the RT alone group [odds ratio (OR) =6.2; 95% confidence interval (CI): 2.0–19.8; P=0.001] which resulted in meeting the predetermined early stopping rule criterion and early closure of the trial (11). Notably, 20% of patients in the RT alone group had worsening motor strength during RT and underwent surgery (11). Furthermore, a prospective quality of life (QoL) analysis by Morgen et al. in 2016 showed patients who underwent surgery + RT had a higher Euroqol-5 dimensions questionnaire (EQ-5D) QoL score (0.71; 95% CI: 0.64–0.71) than patients treated with RT alone (0.63; 95% CI: 0.56–0.70) even though patients who underwent surgery had lower baseline EQ-5D scores (0.28; 95% CI: 0.19–0.36 compared to 0.42; 95% CI: 0.38–0.46) (31). Table 2 summarizes the results of prospective trials of MESCC in patients treated with primary surgery followed by RT, and Table 3 summarizes those with primary RT using the most common conventional palliative radiation schedules. Additionally, a propensity matched analysis by Rades et al. in 2022 compared patients who received surgery + RT to patients who received RT alone and showed improved motor function occurred more often (39.2% vs. 21.5%, P=0.015) in patients who received surgery + RT (32). However, not everyone may benefit from surgery. Patients with tandem stenosis, or distinct levels of MESCC, were excluded from the Patchell study. Hence, the primary treatment for these patients is less clear but may include a variety of approaches such as surgery to multiple sites of MESCC, particularly if accessible from a single incision, surgery to only the most advanced site of MESCC with RT to the remaining sites, or RT to all sites. The previously mentioned study by Morgen et al. showed that patients who underwent surgery and survived less than 6 months had a decline in EQ-5D QoL score (baseline of 0.21; 95% CI: 0.08–0.35) at each follow-up and measured 0.12 (95% CI: −0.09 to 0.34) after 12 weeks (31). In addition, Rades et al. showed 15.2% of patients died within 30 days following surgery (compared to 12.7% following RT alone, P=0.65), and 36.7% of surgically treated patients did not complete RT as planned due to death or decreased performance following surgery (36). Also, the need for adequate wound healing may potentially delay RT. Therefore, when considering surgery, one should consider the total disease burden of the patient—including stage, presence of multilevel disease, and non-skeletal involvement—as well as available therapies. Prognostic tools such as the revised Tokuhashi scoring system and the more recently validated SORG Orthopaedic Research Group machine learning algorithm and nomogram can aid in survival estimation of patients with spinal metastases (37-42). Medical comorbidities and age should be taken into consideration for perioperative risk and survival. A secondary analysis of the landmark Patchell study showed that patients 65 years of age and older benefited less, in terms of ambulation and survival, from surgery than patients less than 65 years of age (43). Thus, surgery followed by RT should be considered first-line as primary therapy for MESCC, but a patient’s expected survival, risk of perioperative mortality, and rehabilitative capability should be considered alongside the benefits of surgery.
Table 2
Patient cohort | N | Baseline ambulatory | Radiosensitive histology | Response | Survival |
---|---|---|---|---|---|
30 Gy in 10 fractions | |||||
Young (10), laminectomy only | 16 | 38% | 25% | Pain: 38% improved; urinary continence: 75% maintained, 12.5% regained; ambulation: 50% maintained, 44% regained | Mean 6.4 months |
Patchell (11), circumferential decompression ± stabilization | 50 | 68% | 0% | Medications: MDDED 1.6 mg, MDMED 0.4 mg; urinary continence: median 5.2 months maintained; strength: 86% maintained/improved; ambulation: 94% maintained, 62% regained, median 4.1 months | Median 4.2 months |
Morgen (31) | 47 | NR | NR | QoL: EQ-5D score increased from 0.28 to 0.44 after 6 weeks and 0.71 after 1 year | Median 10.8 months |
MESCC, malignant epidural spinal cord compression; RT, radiation therapy; N, number of patients; MDDED, mean daily dexamethasone equivalent dose; MDMED, mean daily morphine equivalent dose; NR, not reported; QoL, quality of life; EQ-5D, Euroqol-5 dimensions questionnaire.
Table 3
Patient cohort | N | Baseline ambulatory | Radiosensitive histology | Response | Survival |
---|---|---|---|---|---|
30 Gy (3 Gy × 10 fractions) | |||||
Maranzano (22) | 20 | 80% | 0% | Pain: 85% improved; ambulation: 100% maintained, 100% regained | Median 14 months |
Rades (32) | 110 | 53% | 16% | Strength: 73% maintained/improved; ambulation: 88% maintained, 29% regained, 75% ambulatory after 6 months | 6-month OS 69% |
Patchell (11) | 51 | 69% | 0% | Medications: MDDED 4.2 mg, MDMED 4.8 mg; urinary continence: median 17 days maintained; strength: 60% maintained/improved; ambulation: 74% maintained, 19% regained, median 13 days | Median 3.3 months |
Graham (25) | 20 | 75% | 0% | Pain: mean score of 4.8 decreased to 2.7 after 1 day; ambulation: 81% after 1 month | Median 2.3 months |
Abu-Hegazy (33) | 100 | 61% | 12% | Pain: 56% improved; sensation: 32% regained; urinary continence: 70% regained; ambulation: 100% maintained, 67% regained; 2-year IFR: 16% occurred at med 5 months | 2-year OS 56% |
Rades (15) and Rades (16) | 102 | 58% | 8% | Pain: 57% improved, 20% resolved; strength: 90% maintained/improved; ambulation: 74% after 1 months; 6-month LPFS: 82% | 6-month OS 38% |
20 Gy (4 Gy × 5 fractions) | |||||
Rades (15) and Rades (16) | 101 | 59% | 8% | Pain: 53% improved, 24% resolved; strength: 87% maintained/improved; ambulation: 72% after 1 month; 6-month LPFS: 75% | 6-month OS 42% |
Thirion (12) and Lee (13) | 59 | 76% | 0% | EORTC QLQ-C30 QoL: summary score 10.8 improved, function 8.1 improved, and pain 25.7 improved; urinary continence: 75% maintained/improved; ambulation: 57% maintained, 44% regained; re-irradiation: 5% after med 6.6 months | Median 6 months |
Hoskin (34) | 176 | 66% | 0% | Ambulation: 83% maintained, 41% regained | 3-month OS 55% |
8 Gy (8 Gy × 1 fraction) | |||||
Maranzano (18) | 153 | 64% | 4% | Pain: 57% improved, 27% resolved; urinary continence: 95% maintained, 35% regained; ambulation: 88% maintained, 16% regained | Median 4 months |
Abu-Hegazy (33) | 95 | 60% | 7% | Pain: 55% improved; sensation: 28% regained; urinary continence: 70% regained; ambulation: 100% maintained, 66% regained; 2-year IFR: 22% occurred at median 5 months | 2-year OS 57% |
Giraldo (35) | 35 | 43% | 0% | Pain: mean score of 8 decreased to 5; urinary continence and ambulation: 100% maintained, 0% regained | Median 1.5 months |
Hoskin (34) | 166 | 66% | 0% | Ambulation: 78% maintained, 50% regained | 3-month OS 50% |
MESCC, malignant epidural spinal cord compression; RT, radiation therapy; N, number of patients; OS, overall survival; MDDED, mean daily dexamethasone equivalent dose; mg, milligram; MDMED, mean daily morphine equivalent dose; IFR, in-field recurrence; LPFS, local progression free survival; EORTC, European Organization for Research and Treatment of Cancer; QLQ-C30, Quality of Life Questionnaire Core 30; QoL, quality of life.
In addition to survival and surgical tolerability, there are several factors to consider when deciding between primary surgery or radiation. If there is spinal instability associated with the lesion of interest, then surgical stabilization would be favored to primary RT. Spinal stability should be characterized by the Spine Instability Neoplastic Score (SINS) described by Fisher et al.; a score of 0 to 6 is considered stable, 7 to 12 indeterminate or possible impending instability, and 13 to 18 unstable (44). A score of greater than 7 warrants surgical evaluation for consideration of spinal stabilization. Orthotic bracing, with confirmatory upright X-rays of the spine, may also assist with spinal stabilization. However, if there is displacement of normal bone causing spinal cord compression, then surgery is recommended (45). Primary surgery is typically favored when tumors cause high grade Bilsky compression or significant symptoms since surgery is more likely to improve symptoms (11) and offer more rapid decompression than a progressive response from radiation. Still, significant responses are still possible with RT alone (11,12,15,18,22,25,32-34). Time to developing motor symptoms can be a prognostic indicator of response to RT. Rades et al. categorized the time from onset of any symptoms to time of developing motor deficits into three groups: 1–7, 8–14, and >14 days (46). Patients in the >14 day group were significantly more likely to have functional improvement (86% vs. 29% and 10%, P<0.001) and ambulate post-RT (86% vs. 55% and 35%, P=0.026) than those in the 8–14 day group and 1–7 day group, respectively (46). Furthermore, patients who developed motor deficits in <48 hours mostly had irreversible symptoms with RT alone (46,47). The reasoning is that acute onset of motor symptoms is due to arterial blockage leading to spinal cord infarction, while slow onset is due to venous congestion. Based on this data, for patients with rapid onset of motor symptoms, emergent surgery should be preferred over RT. Besides time to developing motor deficits, duration of symptoms is also prognostic. Symptoms lasting <48 hours are favorably prognostic for regaining ambulatory function with primary surgery followed by RT (48). Regardless of whether RT is delivered adjuvantly or as primary treatment, history of RT should be taken into account, and re-irradiation should only occur if there is acceptable risk from the cumulative dose of radiation. Radiation dosing will be discussed below. Another instance when primary surgery may be preferred is when a diagnosis needs to be established, and emergent treatment cannot await a biopsy result. However, when patients are known to have a radioresponsive tumor (e.g., lymphoma, multiple myeloma, leukemia, Ewing sarcoma, germ cell, and small cell lung cancer), patients may achieve excellent response with primary RT (7,49-51). The decision regarding primary surgery followed by RT versus primary RT is multifactorial and should involve input from both spine surgeons and radiation oncologists.
When to consider primary systemic therapy
Systemic therapy, or chemotherapy, is rarely given emergently for MESCC, and primary therapy usually consists of tumor directed treatment either with surgery or RT (1,4,11,52). Systemic therapy can be given after primary treatment, and the decision is based on several factors including but not limited to tumor histology, stage, metastatic extent of disease, performance status, tolerability, and patient goals. If given, systemic therapy may help provide control to both MESCC and other sites of disease. A comprehensive discussion on systemic therapy options following primary treatment of MESCC is beyond the scope of this paper. However, depending on tumor histology, systemic therapy may be given as primary treatment for MESCC. Tumors that may benefit from primary chemotherapy include chemoresponsive histologies such as germ cell, neuroblastoma, Ewing sarcoma, leukemia, lymphoma, and myeloma, which have an 86–100% response rate with nonsurgical management of MESCC (53-56).
In some cases, chemotherapy may be safer given alone, while in other cases, more effective when combined with RT. For patients with germ cell tumors, a retrospective study by Grommes et al. with unbalanced patient characteristics showed worse survival in patients who received chemotherapy combined with RT compared to those who received chemotherapy alone (53). For patients with MESCC as the initial manifestation of lymphoma, a randomized controlled trial by Avilés et al. showed a combination of systemic therapy and RT provided greater event-free survival and overall survival but similar neurologic recovery compared to either modality alone (57). In a group of patients with MESCC secondary to lymphoma or myeloma, Wallington et al. reported 6 of 24 patients (25%) who received chemotherapy died due to toxicity from chemotherapy (52). If considering combined modality, one should weigh the potential benefits with the increased risk of toxicity on a case-by-case basis. Furthermore, if treatment intent is curative, one should convert to a definitive dose of radiotherapy after emergent initiation for durable local control. Medical oncology should be involved in the care of patients with MESCC to help provide additional information on prognosis and potential systemic therapy options whether given emergently or following primary treatment.
Is there an optimal RT dose and schedule?
Clinical outcomes of primary RT for MESCC vary widely so it is important to compare RT regimens using randomized control trials and well designed, balanced retrospective studies. Recovery of ambulation ranges from 0–100%, 6-month overall survival 38–90%, and 2-year survival 4–81% depending on factors such as tumor histology, number of involved vertebra, ambulatory function pre and post treatment, time from diagnosis to development of MESCC, time to development of motor symptoms, response to treatment, presence of non-skeletal metastasis, receipt of systemic therapy, and performance status (4,6,7,11,12,15,18,22,25,32-35,48,50,58-65). Table 3 summarizes the results of prospective MESCC trials involving the most common palliative radiation schedules (30 Gy in 10 fx, 20 Gy in 5 fx, and 8 Gy in 1 fx), although several other regimens have been reported (6,7,11,12,14,15,18,21,22,25,32-35,46,49,50,54,66-68).
Multiple studies have analyzed different conventional palliative RT regimens for MESCC. A multicenter randomized control trial by Hoskin et al. showed patients treated with 8 Gy in 1 fx had non-inferior ambulation at 12 weeks compared to patients who received 20 Gy in 5 fx (34). Also, a randomized control trial by Maranzano et al. compared 8 Gy in 1 fx to 16 Gy in 2 fx (separated by 1 week) in patients with a life expectancy ≤6 months and found similar duration of response (4.5 vs. 5 months, respectively, P=0.4) (18). A randomized control trial by Abu-Hegazy et al. showed similar improvement in ambulation (P=0.32) and sphincter control (P=0.41) for patients treated with 8 Gy in 1 fx, 30 Gy in 10 fx, and 40 Gy in 20 fx, respectively (33). Potential disadvantages of single fx RT, however, include a greater risk of recurrence and need for re-treatment. Abu-Hegazy et al. found that the risk of recurrence at 2 years was 22% with 8 Gy in 1 fx, 16% with 30 Gy in 10 fx, and 14% with 40 Gy in 20 fx (P=0.01); yet, recurrences occurred at a median of 5 months following RT (33) which may not be relevant in patients with shorter survival. In another randomized control trial, Thirion et al. showed non-inferior treatment responses, but re-treatment at a median follow-up of 6.6 months was more common (25% vs. 5.4%, P=0.024) in patients who received 10 Gy in 1 fx than 20 Gy in 5 fx (12). A balanced multicenter retrospective analysis of 5 fractionation schedules (8 Gy in 1 fx, 20 Gy in 5 fx, 30 Gy in 10 fx, 37.5 Gy in 15 fx, and 40 Gy in 20 fx) by Rades et al. showed similar post-treatment ambulatory response (69%, 68%, 63%, 66%, and 74%, P=0.578, respectively) and improved motor function; although, in-field recurrence at 2 years (24%, 26%, 14%, 9%, and 7%, P<0.001) was more common with short course than with long course RT (60). Neither the difference between 8 Gy in 1 fx and 20 Gy in 5 fx (P=0.44) nor among 30 Gy in 10 fx, 37.5 Gy in 15 fx, and 40 Gy in 20 fx (P=0.71) was significant (60). Similarly, the SCORE-1 prospective trial by Rades et al. showed that short course (8 Gy in 1 fx or 20 Gy in 5 fx) RT compared to long course (30 Gy in 10 fx, 37.5 Gy in 15 fx, or 40 Gy in 20 fx) RT achieved similar improvement in motor function (P=0.95) but was associated with lower 1-year local control (61% vs. 81%, P=0.005, multivariate P=0.018) (14,64). The SCORE-2 randomized control trial by Rades et al. compared 20 Gy in 5 fx to 30 Gy in 10 fx and found non-inferior 1-month motor function overall response (87% and 90%, P=0.73) (15). Motor function improvement (P=0.44) and 6-month local progression-free survival (LPFS) (75% vs. 82%, P=0.51) were also similar between 20 Gy in 5 fx and 30 Gy in 10 fx (15). Still, since longer-term outcomes at 1- and 2-years were superior with 30 Gy in 10 fx than 20 Gy in 5 fx (14,60), but the first regimen is twice as long, Rades et al. designed the multicenter prospective pre-mode trial (17). Volumetric modulated arc therapy (VMAT) was used to deliver 25 Gy in 5 fx, which has similar biologic effectiveness to 30 Gy in 10 fx (17). When propensity matched to patients who received 20 Gy in 5 fx, patients who received 25 Gy in 5 fx had similar improvement in motor function (P=0.51) but superior 6-month LPFS (95% vs. 79%, P=0.026) (17). Patients who received 25 Gy in 5 fx in the Pre-mode trial were also propensity matched to patients who received 30 Gy in 10 fx, and both patient groups had similar response in motor function (P=0.71) as well as 6-month LPFS (94% vs. 87%, P=0.36) (69). A multicenter prospective randomized control trial by Rades et al. compared 30 Gy in 10 fx to 40 Gy in 20 fx and showed similar improvement in motor function (P=0.928) as well as post-RT ambulatory rates (P=0.777) (32). The RT schedules mentioned in the above studies all had similar overall survival when baseline patient characteristics were controlled and were associated with minimal toxicity, which would be expected within the spinal cord tolerance dose (11-18,22,25,32-35,70).
Based on the available studies, the following interpretations can be made regarding conventional RT for MESCC. Split course regimens have not been shown to be advantageous compared to daily schedules (7,18,66) and prolong treatment time in a population of patients that have limited survival, so split course RT is not recommended. For patients with poor expected survival (i.e., ≤4–5 months), 8 Gy in 1 fx is generally preferred as it involves the least patient time and would likely have an initial response similar to that of multi-fraction regimens, (12,14,18,33,34,60,64) although 20–25 Gy in 5 fx may be used in select patients (e.g., those with radioresistant tumors). For patients with longer expected survival (i.e., ≥6 months), 30 Gy in 10 fx is generally favored to help reduce the risk of recurrence (14,33,60,64), and 20–25 Gy in 5 fx may be considered (15,17). While alternate RT schedules (e.g., 28 Gy in 7 daily fx or 16–20 Gy in 4 fx given twice daily) exist (6,67), these have not been directly compared to more common fractionations for MESCC and may have less re-irradiation options due to the maximum cumulative spinal cord tolerance. Treatment courses longer than 30 Gy in 10 fx are not recommended as these have not been shown to be more effective (32,60), would prolong the treatment course, as well as limit the ability to provide re-irradiation if needed. Additional factors to consider when determining the optimal RT schedule include but are not limited to the patient’s goals of care, systemic therapy plan, ability to return for re-treatment, and disposition.
The discussion on different radiation schedules thus far has been regarding primary RT for MESCC. In contrast, post-operative RT fractionation is less heterogenous. Post-operative conventional RT studies typically utilizes 30 Gy in 10 fx (10,11,31,32) given 2–4 weeks following surgery. Compared to some primary RT regimens, smaller fraction sizes (e.g., 3 Gy instead of 8 Gy fx) are used postoperatively as this likely reduces late normal tissue toxicity and there is less need for accelerated RT since the spinal cord is already surgically decompressed. In addition, Koswig et al. showed bone remineralization is less compromised with 30 Gy in 10 fx than 8 Gy in 1 fx (71). Patients who undergo surgery are typically those expected to have appreciable expected survival. Therefore, in the post-operative setting, 30 Gy in 10 fx is favored to minimize risk of recurrence and toxicity.
If recurrence occurs (expected in 23–39% of patients depending on chosen RT schedule), treatment with surgery, re-irradiation, and/or systemic therapy may be considered (33,60,64,72). If re-irradiation is considered, the cumulative biological effective dose is recommended to be below 120–140 Gy2 and ideally at least 6 months would have passed from the initial treatment to minimize risk of RT myelopathy (62,72-75). In a multicenter retrospective study by Rades et al., patients who were initially treated with primary RT and recurred had 85%, 35%, and 0% improvement in motor function with surgery, re-irradiation, and chemotherapy, respectively (60). Patients who initially received a longer course of RT were less likely to receive retreatment (60). Additional studies showed 0–36% of patients who received re-irradiation had improvement in motor function and 50–86% had stable motor function (62,72) with a median duration of 4.5 months (72). While these studies used conventional RT, SBRT may be safely used for re-irradiation with local control rates ranging from 83–95% at 1 year (76-79). Although data is limited, surgery and SBRT may be preferred to conventional RT for MESCC recurrence.
Stereotactic body RT
Primary spinal SBRT, which involves a relatively high dose of radiation delivered to a focused area with steep dose-falloff, may potentially offer better local control and symptom improvement than conventional RT (11,15,80-84). Figure 2 shows an example case of SBRT for decompression of MESCC. As can be appreciated in the figure, SBRT minimizes dose to surrounding organs, particularly bowel in this case, compared to conventional RT with anterior/posterior beams. However, SBRT is generally less feasible for emergent treatment of MESCC as the planning is more complex and time-consuming (85). There are institutions with the ability to deliver spinal SBRT within 24–48 hours of consult resulting in favorable rate of epidural tumor shrinkage (81,86-88). Nonetheless, conventional RT can be delivered more quickly, and surgery would offer the most immediate decompression which is invaluable for patients with significant motor deficits (e.g., motor strength ≤3/5) who are expected to respond to therapy. In addition, SBRT is not well suited for patients with rapid development of motor symptoms, spinal instability, compression from a retropulsed bone fracture, or high grade (Bilsky ≥2) epidural compression (81,82,89). If SBRT is used for high grade MESCC, it should be used with extreme caution, and the epidural space may need to be underdosed in order to respect the tolerance of the spinal cord, which may inadvertently reduce local control (90,91). In cases with high grade compression, patients with poor expected survival may receive primary conventional RT as previously described, while those with more favorable prognosis may benefit from primary separation surgery, which may be done with minimally invasive techniques, followed by post-operative SBRT (92-94). Of note, if significant metal artifact is present or expected on a post-operative MRI, a CT myelogram (which may be uncomfortable for some patients) may be needed to better delineate the target volume and spinal cord (30,93,95). In the non-emergent setting (i.e., patients with spine metastases or low-grade MESCC without neurologic deficits), a randomized control trial by Sahgal et al. showed SBRT of 24 Gy in 2 fx had improved complete response in pain (35% vs. 14% at 3 months, P=0.0002; 32% vs. 16% at 6 months, P=0.0036) (83). Although not statistically significant, SBRT also had improved local control rate (97% vs. 90% at 6 months) and radiation site-specific PFS (92% vs. 86% at 3 months, P=0.18; 75% vs. 69% at 6 months, P=0.34) compared to conventional RT of 20 Gy in 5 fx (83). However, SBRT is not as widely available and conventional RT may still be favored in patients with radioresponsive tumors, diffuse multilevel disease, or poor performance status (89). An in-depth analysis of spinal SBRT is beyond the scope of this paper. For additional details, please see the practice guidelines by Jabbari et al. for criteria and contraindications for SBRT (89). A review paper on spinal metastases by Spratt et al. also illustrates an algorithm which may be helpful with therapeutic decision-making (30). If SBRT is planned, contouring should be performed according to guidelines by Cox et al. and Redmond et al. for the primary and post-operative settings, respectively (95-98). Studies on dose constraints are also available for reference (74,89,99-101). Although SBRT may be advantageous for early MESCC and in the post-operative setting, either primary surgery or conventional RT would be generally preferred for emergent cases.
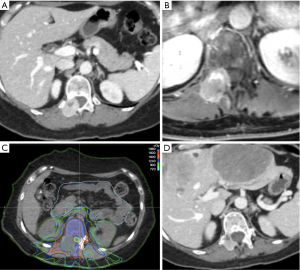
Future research
While advancements in both surgery and RT have improved clinical outcomes for the treatment of MESCC, prognosis is still poor for many patients, particularly those with loss of ambulation (65). Therefore, future research should investigate the possibility of early detection. If MESCC is detected prior to high-grade compression or deterioration of neurologic function, patients may potentially benefit more from SBRT over conventional RT. Additionally, if MESCC is controlled prior to development of significant motor deficits, emergent treatment with surgery or RT may potentially be avoided. While a randomized control trial comparing primary SBRT to conventional RT exists (83), further research should also include randomized control trials comparing primary SBRT to surgery in appropriately selected patients, post-operative SBRT to conventional RT, and SBRT to conventional RT in the re-irradiation setting. Importantly, future studies should ideally report standard response assessments when comparing treatments as recommended by the SPINO group (102,103).
Conclusions
MESCC is a devastating condition that is associated with limited survival although outcomes are variable based on prognostic factors. Patients with high grade MESCC or significant symptoms necessitate emergent treatment. Primary treatment includes steroids followed by either surgery with RT or RT alone. In select uncommon situations for chemosensitive histologies, chemotherapy ± RT may be used as primary treatment. Various fractionations of conventional RT exist, although 8 Gy in 1 fx is favored in patients with poor expected survival (i.e., ≤4–5 months) and 30 Gy in 10 fx is preferred in patients with longer (≥6 months) survival. However, the decision regarding optimal fractionation is multifactorial and should be personalized to each patient. SBRT may improve symptom and local control although level I data is limited, and SBRT in general is not well suited for emergent treatment or high grade MESCC. See Table 4 for a clinical decision-making tool summarizing scenarios when each modality (surgery, conventional RT, SBRT, or chemotherapy) may be preferred as primary treatment for MESCC. Future research should investigate the possibility of improving early detection so patients may benefit more from advancements in therapy. Emergent MESCC should be approached collaboratively by a multidisciplinary team including spine surgeons, radiation oncologists, and medical oncologists.
Table 4
Primary surgery followed by radiation therapy |
Significant motor deficit |
Rapid development of deficits (<48 h) |
Significant epidural compression (grade ≥2) |
No contraindication to surgery (e.g., life expectancy ≤3 months) |
Primary conventional radiation therapy |
Not surgical candidate |
Stable spine |
Radiosensitive/radioresponsive |
Diffuse disease |
Cumulative radiation normal tissue tolerance would not be exceeded |
Primary stereotactic body radiation therapy |
Nonemergent treatment (due to planning time) |
Stable spine |
Radioresistant |
Prior radiation |
Mild epidural compression (ideally ≥2 mm from cord) |
Longer life expectancy |
Primary chemotherapy ± radiation therapy |
Chemosensitive/chemoresponsive* |
Chemotherapy can be started urgently |
No contraindication to chemotherapy (e.g., low blood cell counts) |
Best supportive care |
Poor life expectancy |
Rapid development of deficits with loss of ambulation |
Not a candidate for surgery or chemotherapy |
*, chemosensitive/chemoresponsive tumors are often also radiosensitive/radioresponsive. MESCC, malignant epidural spinal cord compression.
Acknowledgments
Funding: None.
Footnote
Provenance and Peer Review: This article was commissioned by the editorial office, Annals of Palliative Medicine, for the series “Radiotherapy for Oncologic Emergencies”. The article has undergone external peer review.
Reporting Checklist: The authors have completed the Narrative Review reporting checklist. Available at https://apm.amegroups.com/article/view/10.21037/apm-23-342/rc
Peer Review File: Available at https://apm.amegroups.com/article/view/10.21037/apm-23-342/prf
Conflicts of Interest: All authors have completed the ICMJE uniform disclosure form (available at https://apm.amegroups.com/article/view/10.21037/apm-23-342/coif). The series “Radiotherapy for Oncologic Emergencies” was commissioned by the editorial office without any funding or sponsorship. S.S.L. and S.K.S. served as unpaid Guest Editors of the series. S.S.L. serves as an unpaid Associate Editor-in-Chief of Annals of Palliative Medicine from February 2022 to January 2024. Unrelated to this work, A.B. reports stock holdings in Pfizer and GSK plc; Y.D.T. reports leadership role as Chair of the ASTRO scientific committee Palliative Care track and honoraria for ASTRO refresher course (2020–2021). A.G.A. reports travel support from ICOTEC; B.V. reports honoraria from Elekta AB and travel expenses from Elekta AB and Brainlab AG; J.D.P. reports honoraria from Varian, ICOTEC, and Novocure, travel support from Varian and ICOTEC, and advisory board role for Novocure. S.T.C. reports honoraria from Varian, and honoraria and travel support from Blue Earth Diagnostics. M.F. reports consultancy fees and honoraria from Elekta and Varian, travel support from Elekta, and Ex Officio Board Member role for ISRS. K.R. reports research funding to her institution from Accuray, Canon, and Elekta, consulting fees from ICOTEC; honoraria from Accuray, travel support from Brainlab, Accuray, and Elekta, payments from BioMimetix, a patent under development for radiogenomics in collaboration with Canon, and leadership role as CNS track chair for ASTRO education committee; A.S. reports research grants from Elekta AB, Varian, Seagen, and BrainLab, consulting fees from Elekta AB, Varian, Merck, Abbvie, Roche, and BrainLab, honoraria from AstraZeneca, Elekta AB, Varian, Seagen, Accuray, and BrainLab, and leadership roles as Vice President of ISRS, Co-Chair of the AO Spine Knowledge Forum, and Member of the Elekta MR Linac Research Consortium. S.S.L. reports research funding from the NCI and Kuni foundation, travel support from JASTRO, and leadership roles as Assistant Councilor and Chair of CARROS Nominating Committee for the ACR and Member of the Board of Directors and Medical Director of Distinction in Practice in Stereotactic Radiotherapy Program for the Radiosurgery Society. The authors have no other conflicts of interest to declare.
Ethical Statement: The authors are accountable for all aspects of the work in ensuring that questions related to the accuracy or integrity of any part of the work are appropriately investigated and resolved.
Open Access Statement: This is an Open Access article distributed in accordance with the Creative Commons Attribution-NonCommercial-NoDerivs 4.0 International License (CC BY-NC-ND 4.0), which permits the non-commercial replication and distribution of the article with the strict proviso that no changes or edits are made and the original work is properly cited (including links to both the formal publication through the relevant DOI and the license). See: https://creativecommons.org/licenses/by-nc-nd/4.0/.
※Special series on Radiotherapy for Oncologic Emergencies.
References
- Loblaw DA, Laperriere NJ, Mackillop WJ. A population-based study of malignant spinal cord compression in Ontario. Clin Oncol (R Coll Radiol) 2003;15:211-7. [Crossref] [PubMed]
- U.S. Cancer Statistics Working Group. U.S. Cancer Statistics Data Visualizations Tool, based on 2021 submission data (1999-2019): U.S. Department of Health and Human Services, Centers for Disease Control and Prevention and National Cancer Institute. 2022. Available online: www.cdc.gov/cancer/dataviz
- Barron KD, Hirano A, Araki S, et al. Experiences with metastatic neoplasms involving the spinal cord. Neurology 1959;9:91-106. [Crossref] [PubMed]
- Gilbert RW, Kim JH, Posner JB. Epidural spinal cord compression from metastatic tumor: diagnosis and treatment. Ann Neurol 1978;3:40-51. [Crossref] [PubMed]
- Constans JP, de Divitiis E, Donzelli R, et al. Spinal metastases with neurological manifestations. Review of 600 cases. J Neurosurg 1983;59:111-8. [Crossref] [PubMed]
- Helweg-Larsen S, Sørensen PS, Kreiner S. Prognostic factors in metastatic spinal cord compression: a prospective study using multivariate analysis of variables influencing survival and gait function in 153 patients. Int J Radiat Oncol Biol Phys 2000;46:1163-9. [Crossref] [PubMed]
- Maranzano E, Latini P, Checcaglini F, et al. Radiation therapy in metastatic spinal cord compression. A prospective analysis of 105 consecutive patients. Cancer 1991;67:1311-7. [Crossref] [PubMed]
- Schiff D, O'Neill BP, Wang CH, et al. Neuroimaging and treatment implications of patients with multiple epidural spinal metastases. Cancer 1998;83:1593-601. [Crossref] [PubMed]
- van der Sande JJ, Kröger R, Boogerd W. Multiple spinal epidural metastases; an unexpectedly frequent finding. J Neurol Neurosurg Psychiatry 1990;53:1001-3. [Crossref] [PubMed]
- Young RF, Post EM, King GA. Treatment of spinal epidural metastases. Randomized prospective comparison of laminectomy and radiotherapy. J Neurosurg 1980;53:741-8. [Crossref] [PubMed]
- Patchell RA, Tibbs PA, Regine WF, et al. Direct decompressive surgical resection in the treatment of spinal cord compression caused by metastatic cancer: a randomised trial. Lancet 2005;366:643-8. [Crossref] [PubMed]
- Thirion PG, Dunne MT, Kelly PJ, et al. Non-inferiority randomised phase 3 trial comparing two radiation schedules (single vs. five fractions) in malignant spinal cord compression. Br J Cancer 2020;122:1315-23. [Crossref] [PubMed]
- Lee KA, Dunne M, Small C, et al. (ICORG 05-03): prospective randomized non-inferiority phase III trial comparing two radiation schedules in malignant spinal cord compression (not proceeding with surgical decompression); the quality of life analysis. Acta Oncol 2018;57:965-72. [Crossref] [PubMed]
- Rades D, Lange M, Veninga T, et al. Preliminary results of spinal cord compression recurrence evaluation (score-1) study comparing short-course versus long-course radiotherapy for local control of malignant epidural spinal cord compression. Int J Radiat Oncol Biol Phys 2009;73:228-34. [Crossref] [PubMed]
- Rades D, Šegedin B, Conde-Moreno AJ, et al. Radiotherapy With 4 Gy × 5 Versus 3 Gy × 10 for Metastatic Epidural Spinal Cord Compression: Final Results of the SCORE-2 Trial (ARO 2009/01). J Clin Oncol 2016;34:597-602. [Crossref] [PubMed]
- Rades D, Šegedin B, Conde-Moreno AJ, et al. Patient-Reported Outcomes-Secondary Analysis of the SCORE-2 Trial Comparing 4 Gy × 5 to 3 Gy × 10 for Metastatic Epidural Spinal Cord Compression. Int J Radiat Oncol Biol Phys 2019;105:760-4. [Crossref] [PubMed]
- Rades D, Cacicedo J, Conde-Moreno AJ, et al. Precision Radiation Therapy for Metastatic Spinal Cord Compression: Final Results of the PRE-MODE Trial. Int J Radiat Oncol Biol Phys 2020;106:780-9. [Crossref] [PubMed]
- Maranzano E, Trippa F, Casale M, et al. 8Gy single-dose radiotherapy is effective in metastatic spinal cord compression: results of a phase III randomized multicentre Italian trial. Radiother Oncol 2009;93:174-9. [Crossref] [PubMed]
- McClelland S 3rd, Kim E, Passias PG, et al. Spinal stereotactic body radiotherapy in the United States: A decade-long nationwide analysis of patient demographics, practice patterns, and trends over time. J Clin Neurosci 2017;46:109-12. [Crossref] [PubMed]
- Bilsky MH, Laufer I, Fourney DR, et al. Reliability analysis of the epidural spinal cord compression scale. J Neurosurg Spine 2010;13:324-8. [Crossref] [PubMed]
- Sørensen S, Helweg-Larsen S, Mouridsen H, et al. Effect of high-dose dexamethasone in carcinomatous metastatic spinal cord compression treated with radiotherapy: a randomised trial. Eur J Cancer 1994;30A:22-7. [Crossref] [PubMed]
- Maranzano E, Latini P, Beneventi S, et al. Radiotherapy without steroids in selected metastatic spinal cord compression patients. A phase II trial. Am J Clin Oncol 1996;19:179-83. [Crossref] [PubMed]
- Vecht CJ, Haaxma-Reiche H, van Putten WL, et al. Initial bolus of conventional versus high-dose dexamethasone in metastatic spinal cord compression. Neurology 1989;39:1255-7. [Crossref] [PubMed]
- Heimdal K, Hirschberg H, Slettebø H, et al. High incidence of serious side effects of high-dose dexamethasone treatment in patients with epidural spinal cord compression. J Neurooncol 1992;12:141-4. [Crossref] [PubMed]
- Graham PH, Capp A, Delaney G, et al. A pilot randomised comparison of dexamethasone 96 mg vs 16 mg per day for malignant spinal-cord compression treated by radiotherapy: TROG 01.05 Superdex study. Clin Oncol (R Coll Radiol) 2006;18:70-6. [Crossref] [PubMed]
- Kumar A, Weber MH, Gokaslan Z, et al. Metastatic Spinal Cord Compression and Steroid Treatment: A Systematic Review. Clin Spine Surg 2017;30:156-63. [Crossref] [PubMed]
- Zacharia BE, Kahn S, Bander ED, et al. Incidence and risk factors for preoperative deep venous thrombosis in 314 consecutive patients undergoing surgery for spinal metastasis. J Neurosurg Spine 2017;27:189-97. [Crossref] [PubMed]
- Laufer I, Rubin DG, Lis E, et al. The NOMS framework: approach to the treatment of spinal metastatic tumors. Oncologist 2013;18:744-51. [Crossref] [PubMed]
- Raj VS, Lofton L. Rehabilitation and treatment of spinal cord tumors. J Spinal Cord Med 2013;36:4-11. [Crossref] [PubMed]
- Spratt DE, Beeler WH, de Moraes FY, et al. An integrated multidisciplinary algorithm for the management of spinal metastases: an International Spine Oncology Consortium report. Lancet Oncol 2017;18:e720-30. [Crossref] [PubMed]
- Morgen SS, Engelholm SA, Larsen CF, et al. Health-related Quality of Life in Patients with Metastatic Spinal Cord Compression. Orthop Surg 2016;8:309-15. [Crossref] [PubMed]
- Rades D, Fehlauer F, Stalpers LJ, et al. A prospective evaluation of two radiotherapy schedules with 10 versus 20 fractions for the treatment of metastatic spinal cord compression: final results of a multicenter study. Cancer 2004;101:2687-92. [Crossref] [PubMed]
- Abu-Hegazy M, Wahba HA. Single-versus multi-fraction radiation treatment for met-astatic spinal cord compression: functional outcome study. The Chinese-German Journal of Clinical Oncology 2011;10:535-40. [Crossref]
- Hoskin PJ, Hopkins K, Misra V, et al. Effect of Single-Fraction vs Multifraction Radiotherapy on Ambulatory Status Among Patients With Spinal Canal Compression From Metastatic Cancer: The SCORAD Randomized Clinical Trial. JAMA 2019;322:2084-94. [Crossref] [PubMed]
- Giraldo A, Benavente S, Ramos M, et al. Effectiveness of radiotherapy for metastatic spinal cord compression in patients with short life expectancy. Rep Pract Oncol Radiother 2017;22:58-63. [Crossref] [PubMed]
- Rades D, Küchler J, Graumüller L, et al. Radiotherapy with or without Decompressive Surgery for Metastatic Spinal Cord Compression: A Retrospective Matched-Pair Study Including Data from Prospectively Evaluated Patients. Cancers (Basel) 2022;14:1260. [Crossref] [PubMed]
- Tokuhashi Y, Matsuzaki H, Oda H, et al. A revised scoring system for preoperative evaluation of metastatic spine tumor prognosis. Spine (Phila Pa 1976) 2005;30:2186-91. [Crossref] [PubMed]
- Morgen SS, Fruergaard S, Gehrchen M, et al. A revision of the Tokuhashi revised score improves the prognostic ability in patients with metastatic spinal cord compression. J Cancer Res Clin Oncol 2018;144:33-8. [Crossref] [PubMed]
- Karhade AV, Thio QCBS, Ogink PT, et al. Predicting 90-Day and 1-Year Mortality in Spinal Metastatic Disease: Development and Internal Validation. Neurosurgery 2019;85:E671-81. [Crossref] [PubMed]
- Shah AA, Karhade AV, Park HY, et al. Updated external validation of the SORG machine learning algorithms for prediction of ninety-day and one-year mortality after surgery for spinal metastasis. Spine J 2021;21:1679-86. [Crossref] [PubMed]
- Yang JJ, Chen CW, Fourman MS, et al. International external validation of the SORG machine learning algorithms for predicting 90-day and one-year survival of patients with spine metastases using a Taiwanese cohort. Spine J 2021;21:1670-8. [Crossref] [PubMed]
- Zegarek G, Tessitore E, Chaboudez E, et al. SORG algorithm to predict 3- and 12-month survival in metastatic spinal disease: a cross-sectional population-based retrospective study. Acta Neurochir (Wien) 2022;164:2627-35. [Crossref] [PubMed]
- Chi JH, Gokaslan Z, McCormick P, et al. Selecting treatment for patients with malignant epidural spinal cord compression-does age matter?: results from a randomized clinical trial. Spine (Phila Pa 1976) 2009;34:431-5. [Crossref] [PubMed]
- Fisher CG, DiPaola CP, Ryken TC, et al. A novel classification system for spinal instability in neoplastic disease: an evidence-based approach and expert consensus from the Spine Oncology Study Group. Spine (Phila Pa 1976) 2010;35:E1221-9. [Crossref] [PubMed]
- Loblaw DA, Laperriere NJ. Emergency treatment of malignant extradural spinal cord compression: an evidence-based guideline. J Clin Oncol 1998;16:1613-24. [Crossref] [PubMed]
- Rades D, Heidenreich F, Karstens JH. Final results of a prospective study of the prognostic value of the time to develop motor deficits before irradiation in metastatic spinal cord compression. Int J Radiat Oncol Biol Phys 2002;53:975-9. [Crossref] [PubMed]
- Rades D, Blach M, Bremer M, et al. Prognostic significance of the time of developing motor deficits before radiation therapy in metastatic spinal cord compression: one-year results of a prospective trial. Int J Radiat Oncol Biol Phys 2000;48:1403-8. [Crossref] [PubMed]
- Chaichana KL, Woodworth GF, Sciubba DM, et al. Predictors of ambulatory function after decompressive surgery for metastatic epidural spinal cord compression. Neurosurgery 2008;62:683-92; discussion 683-92. [Crossref] [PubMed]
- Kim RY, Smith JW, Spencer SA, et al. Malignant epidural spinal cord compression associated with a paravertebral mass: its radiotherapeutic outcome on radiosensitivity. Int J Radiat Oncol Biol Phys 1993;27:1079-83. [Crossref] [PubMed]
- Bolm L, Janssen S, Bartscht T, et al. Radiotherapy Alone for Malignant Spinal Cord Compression in Young Men with Seminoma. Anticancer Res 2016;36:2033-4. [PubMed]
- Rubin P, Mayer E, Poulter C. Extradural spinal cord compression by tumor. II. High daily dose experience without laminectomy. Radiology 1969;93:1248-60. [Crossref] [PubMed]
- Wallington M, Mendis S, Premawardhana U, et al. Local control and survival in spinal cord compression from lymphoma and myeloma. Radiother Oncol 1997;42:43-7. [Crossref] [PubMed]
- Grommes C, Bosl GJ, DeAngelis LM. Treatment of epidural spinal cord involvement from germ cell tumors with chemotherapy. Cancer 2011;117:1911-6. [Crossref] [PubMed]
- Katagiri H, Takahashi M, Inagaki J, et al. Clinical results of nonsurgical treatment for spinal metastases. Int J Radiat Oncol Biol Phys 1998;42:1127-32. [Crossref] [PubMed]
- Kataoka A, Shimizu K, Matsumoto T, et al. Epidural spinal cord compression as an initial symptom in childhood acute lymphoblastic leukemia: rapid decompression by local irradiation and systemic chemotherapy. Pediatr Hematol Oncol 1995;12:179-84. [Crossref] [PubMed]
- Hayes FA, Thompson EI, Hvizdala E, et al. Chemotherapy as an alternative to laminectomy and radiation in the management of epidural tumor. J Pediatr 1984;104:221-4. [Crossref] [PubMed]
- Avilés A, Fernández R, González JL, et al. Spinal cord compression as a primary manifestation of aggressive malignant lymphomas: long-term analysis of treatments with radiotherapy, chemotherapy or combined therapy. Leuk Lymphoma 2002;43:355-9. [Crossref] [PubMed]
- Zelefsky MJ, Scher HI, Krol G, et al. Spinal epidural tumor in patients with prostate cancer. Clinical and radiographic predictors of response to radiation therapy. Cancer 1992;70:2319-25. [Crossref] [PubMed]
- Cowap J, Hardy JR, A'Hern R. Outcome of malignant spinal cord compression at a cancer center: implications for palliative care services. J Pain Symptom Manage 2000;19:257-64. [Crossref] [PubMed]
- Rades D, Stalpers LJ, Veninga T, et al. Evaluation of five radiation schedules and prognostic factors for metastatic spinal cord compression. J Clin Oncol 2005;23:3366-75. [Crossref] [PubMed]
- Haddad P, Thaell JF, Kiely JM, et al. Lymphoma of the spinal extradural space. Cancer 1976;38:1862-6. [Crossref] [PubMed]
- Rades D, Rudat V, Veninga T, et al. Prognostic factors for functional outcome and survival after reirradiation for in-field recurrences of metastatic spinal cord compression. Cancer 2008;113:1090-6. [Crossref] [PubMed]
- Hoskin PJ, Hopkins K, Misra V, et al. Prognostic factors for survival and ambulatory status at 8 weeks with metastatic spinal cord compression in the SCORAD randomised trial. Radiother Oncol 2022;173:77-83. [Crossref] [PubMed]
- Rades D, Lange M, Veninga T, et al. Final results of a prospective study comparing the local control of short-course and long-course radiotherapy for metastatic spinal cord compression. Int J Radiat Oncol Biol Phys 2011;79:524-30. [Crossref] [PubMed]
- Kim RY, Spencer SA, Meredith RF, et al. Extradural spinal cord compression: analysis of factors determining functional prognosis--prospective study. Radiology 1990;176:279-82. [Crossref] [PubMed]
- Greenberg HS, Kim JH, Posner JB. Epidural spinal cord compression from metastatic tumor: results with a new treatment protocol. Ann Neurol 1980;8:361-6. [Crossref] [PubMed]
- Capuccini J, Macchia G, Farina E, et al. Short-course regimen of palliative radiotherapy in complicated bone metastases: a phase i-ii study (SHARON Project). Clin Exp Metastasis 2018;35:605-11. [Crossref] [PubMed]
- Silva MF, Marta GN, Lisboa FPC, et al. Hypofractionated radiotherapy for complicated bone metastases in patients with poor performance status: a phase II international trial. Tumori 2019;105:181-7. [Crossref] [PubMed]
- Rades D, Cacicedo J, Conde-Moreno AJ, et al. Comparison of 5 × 5 Gy and 10 × 3 Gy for metastatic spinal cord compression using data from three prospective trials. Radiat Oncol 2021;16:7. [Crossref] [PubMed]
- Emami B, Lyman J, Brown A, et al. Tolerance of normal tissue to therapeutic irradiation. Int J Radiat Oncol Biol Phys 1991;21:109-22. [Crossref] [PubMed]
- Koswig S, Budach V. Remineralization and pain relief in bone metastases after after different radiotherapy fractions (10 times 3 Gy vs. 1 time 8 Gy). A prospective study. Strahlenther Onkol 1999;175:500-8. [Crossref] [PubMed]
- Maranzano E, Trippa F, Casale M, et al. Reirradiation of metastatic spinal cord compression: definitive results of two randomized trials. Radiother Oncol 2011;98:234-7. [Crossref] [PubMed]
- Nieder C, Grosu AL, Andratschke NH, et al. Update of human spinal cord reirradiation tolerance based on additional data from 38 patients. Int J Radiat Oncol Biol Phys 2006;66:1446-9. [Crossref] [PubMed]
- Sahgal A, Chang JH, Ma L, et al. Spinal Cord Dose Tolerance to Stereotactic Body Radiation Therapy. Int J Radiat Oncol Biol Phys 2021;110:124-36. [Crossref] [PubMed]
- Wallace ND, Dunne MT, McArdle O, et al. Efficacy and toxicity of primary re-irradiation for malignant spinal cord compression based on radiobiological modelling: a phase II clinical trial. Br J Cancer 2023;128:576-85. [Crossref] [PubMed]
- Mahadevan A, Floyd S, Wong E, et al. Stereotactic body radiotherapy reirradiation for recurrent epidural spinal metastases. Int J Radiat Oncol Biol Phys 2011;81:1500-5. [Crossref] [PubMed]
- Hashmi A, Guckenberger M, Kersh R, et al. Re-irradiation stereotactic body radiotherapy for spinal metastases: a multi-institutional outcome analysis. J Neurosurg Spine 2016;25:646-53. [Crossref] [PubMed]
- Milker-Zabel S, Zabel A, Thilmann C, et al. Clinical results of retreatment of vertebral bone metastases by stereotactic conformal radiotherapy and intensity-modulated radiotherapy. Int J Radiat Oncol Biol Phys 2003;55:162-7. [Crossref] [PubMed]
- Hamilton AJ, Lulu BA, Fosmire H, et al. Preliminary clinical experience with linear accelerator-based spinal stereotactic radiosurgery. Neurosurgery 1995;36:311-9. [Crossref] [PubMed]
- Yamada Y, Bilsky MH, Lovelock DM, et al. High-dose, single-fraction image-guided intensity-modulated radiotherapy for metastatic spinal lesions. Int J Radiat Oncol Biol Phys 2008;71:484-90. [Crossref] [PubMed]
- Ryu S, Rock J, Jain R, et al. Radiosurgical decompression of metastatic epidural compression. Cancer 2010;116:2250-7. [Crossref] [PubMed]
- Gerszten PC, Burton SA, Ozhasoglu C, et al. Radiosurgery for Spinal Metastases Clinical Experience in 500 Cases From a Single Institution. Spine 2007;32:193-9. [Crossref] [PubMed]
- Sahgal A, Myrehaug SD, Siva S, et al. Stereotactic body radiotherapy versus conventional external beam radiotherapy in patients with painful spinal metastases: an open-label, multicentre, randomised, controlled, phase 2/3 trial. Lancet Oncol 2021;22:1023-33. [Crossref] [PubMed]
- Abugharib A, Zeng KL, Tseng CL, et al. Spine Stereotactic Body Radiotherapy for Prostate Cancer Metastases and the Impact of Hormone Sensitivity Status on Local Control. Neurosurgery 2022;90:743-9. [Crossref] [PubMed]
- Solberg TD, Balter JM, Benedict SH, et al. Quality and safety considerations in stereotactic radiosurgery and stereotactic body radiation therapy: Executive summary. Pract Radiat Oncol 2012;2:2-9. [Crossref] [PubMed]
- Weksberg DC, Palmer MB, Vu KN, et al. Generalizable class solutions for treatment planning of spinal stereotactic body radiation therapy. Int J Radiat Oncol Biol Phys 2012;84:847-53. [Crossref] [PubMed]
- Ghia AJ, Guha-Thakurta N, Hess K, et al. Phase 1 Study of Spinal Cord Constraint Relaxation With Single Session Spine Stereotactic Radiosurgery in the Primary Management of Patients With Inoperable, Previously Unirradiated Metastatic Epidural Spinal Cord Compression. Int J Radiat Oncol Biol Phys 2018;102:1481-8. [Crossref] [PubMed]
- Ghia AJ, Guha-Thakurta N, Song J, et al. Phase 1 study of spinal cord constraint relaxation with single session spine stereotactic radiosurgery in the primary management of patients with inoperable, previously irradiated metastatic epidural spinal cord compression. N Am Spine Soc J 2021;6:100066. [Crossref] [PubMed]
- Jabbari S, Gerszten PC, Ruschin M, et al. Stereotactic Body Radiotherapy for Spinal Metastases: Practice Guidelines, Outcomes, and Risks. Cancer J 2016;22:280-9. [Crossref] [PubMed]
- Rothrock RJ, Li Y, Lis E, et al. Hypofractionated spinal stereotactic body radiation therapy for high-grade epidural disease. J Neurosurg Spine 2020; Epub ahead of print. [Crossref] [PubMed]
- Chang EL, Shiu AS, Mendel E, et al. Phase I/II study of stereotactic body radiotherapy for spinal metastasis and its pattern of failure. J Neurosurg Spine 2007;7:151-60. [Crossref] [PubMed]
- Laufer I, Iorgulescu JB, Chapman T, et al. Local disease control for spinal metastases following "separation surgery" and adjuvant hypofractionated or high-dose single-fraction stereotactic radiosurgery: outcome analysis in 186 patients. J Neurosurg Spine 2013;18:207-14. [Crossref] [PubMed]
- Redmond KJ, Sciubba D, Khan M, et al. A Phase 2 Study of Post-Operative Stereotactic Body Radiation Therapy (SBRT) for Solid Tumor Spine Metastases. Int J Radiat Oncol Biol Phys 2020;106:261-8. [Crossref] [PubMed]
- Ito K, Sugita S, Nakajima Y, et al. Phase 2 Clinical Trial of Separation Surgery Followed by Stereotactic Body Radiation Therapy for Metastatic Epidural Spinal Cord Compression. Int J Radiat Oncol Biol Phys 2022;112:106-13. [Crossref] [PubMed]
- Redmond KJ, Robertson S, Lo SS, et al. Consensus Contouring Guidelines for Postoperative Stereotactic Body Radiation Therapy for Metastatic Solid Tumor Malignancies to the Spine. Int J Radiat Oncol Biol Phys 2017;97:64-74. [Crossref] [PubMed]
- Cox BW, Spratt DE, Lovelock M, et al. International Spine Radiosurgery Consortium consensus guidelines for target volume definition in spinal stereotactic radiosurgery. Int J Radiat Oncol Biol Phys 2012;83:e597-605. [Crossref] [PubMed]
- Dunne EM, Sahgal A, Lo SS, et al. International consensus recommendations for target volume delineation specific to sacral metastases and spinal stereotactic body radiation therapy (SBRT). Radiother Oncol 2020;145:21-9. [Crossref] [PubMed]
- Chen X, LeCompte MC, Gui C, et al. Deviation from consensus contouring guidelines predicts inferior local control after spine stereotactic body radiotherapy. Radiother Oncol 2022;173:215-22. [Crossref] [PubMed]
- Sahgal A, Whyne CM, Ma L, et al. Vertebral compression fracture after stereotactic body radiotherapy for spinal metastases. Lancet Oncol 2013;14:e310-20. [Crossref] [PubMed]
- Sahgal A, Ma L, Weinberg V, et al. Reirradiation human spinal cord tolerance for stereotactic body radiotherapy. Int J Radiat Oncol Biol Phys 2012;82:107-16. [Crossref] [PubMed]
- Ryu S, Jin JY, Jin R, et al. Partial volume tolerance of the spinal cord and complications of single-dose radiosurgery. Cancer 2007;109:628-36. [Crossref] [PubMed]
- Laufer I, Lo SS, Chang EL, et al. Population description and clinical response assessment for spinal metastases: part 2 of the SPIne response assessment in Neuro-Oncology (SPINO) group report. Neuro Oncol 2018;20:1215-24. [Crossref] [PubMed]
- Thibault I, Chang EL, Sheehan J, et al. Response assessment after stereotactic body radiotherapy for spinal metastasis: a report from the SPIne response assessment in Neuro-Oncology (SPINO) group. Lancet Oncol 2015;16:e595-603. [Crossref] [PubMed]