Latest clinical research in leptomeningeal disease (LMD)—a narrative review
Introduction
Leptomeningeal disease (LMD), also known as leptomeningeal carcinomatosis or metastasis, is a devastating neurological condition typically developing late in the disease course of cancer patients. Lung cancer, breast cancer and malignant melanoma are the most common primary cancers that spread to the leptomeninges (1). Generally speaking, LMD occurs in 10–15% of patients with metastatic solid cancer. However, the incidence is rising due to modern imaging techniques, more screening brain imaging and longer survival brought by advances in cancer treatment (1,2). LMD is usually diagnosed by clinical symptoms, cerebro-spinal magnetic resonance imaging (MRI) and/or cerebrospinal fluid (CSF) cytology, although in some cases the diagnosis remains challenging (1).
Due to the anatomy of leptomeninges, patients with LMD can have various combinations of symptoms attributable to tumour involvement in the cerebral hemisphere, cranial nerves and spinal cord or nerve roots. The wide range of symptoms can include headache, seizures, mental changes, diplopia, visual field defects, facial weakness or numbness, hearing loss, dysphagia, limbs weakness, radicular pain and paraesthesia over trunk or limbs (3,4). These symptoms may negatively impact the patient’s functioning and well-being, which are often measured with health-related quality of life (HRQoL) instruments. The disease occurs often in the context of advanced disease. Patients with LMD may also present with neurological symptoms or signs related to adverse events of previous treatments.
Current treatments of LMD include focal central nervous system (CNS) radiotherapy, intrathecal pharmacotherapy, and systemic pharmacotherapy. Whole brain radiotherapy has only modest benefits in this population (5).
The median survival time of patients of LMD in solid malignancy is usually very poor and limited to 2–6 months despite anti-tumour treatment (1). While there have been several review articles published on the established treatments in LMD (5-7), there is a need for more research in this area to improve patient outcome. The goals of treatment in LMD have been suggested to be prolongation of survival and preserving the quality of life, e.g., by delaying neurological deterioration (5). To achieve these goals, clinical trials in LMD should ideally have both the length of survival and clinical status and HRQoL as endpoints.
Many of the recent diagnostic and therapeutic studies for LMD are biomarker-driven. Biomarker-driven diagnostic studies typically focused on the detection of specific genetic alteration, such as the epidermal growth factor receptor (EGFR) mutation in non-small cell lung cancer (NSCLC). Biomarker-driven therapeutic studies often explored the intracranial efficacy, including in LMD, of systemic target therapy, such as osimertinib for EGFR mutated NSCLC or anti-HER2 antibodies for HER2 overexpressed breast cancer. These biomarker specific diagnostic or therapeutic studies have been extensively covered in published review articles (8-10). Therefore, the use of biomarker-driven systemic treatment in LMD will not be the focus of our review. This narrative review aims to highlight diagnostic or treatment approaches who are not solely dependent on the presence of specific biomarkers. These are often early-stage studies which help determine the feasibility of research along a certain direction, and may therefore have significant impact on the management of LMD arising from various primary sites. We present this article in accordance with the Narrative Review reporting checklist (available at https://apm.amegroups.com/article/view/10.21037/apm-23-390/rc).
Methods
The database of clinicaltrials.gov was searched on 5 Nov 2022 using the keyword “leptomeningeal” under “condition or disease”. Inclusion criteria for our review were (I) clinical trials which are “not yet recruiting”, “recruiting”, “enrolling by invitation” or “active, not recruiting”; and (II) targeted at patient with LMD arising from a solid malignancy. Clinical trials of all phases and observation studies were included. Studies unrelated to diagnostic or therapeutic intervention were excluded. The MEDLINE (via PubMed) and EMBASE (via Elsevier) database were searched to identify any published results of the clinical trials included in this review. The summary of search strategy is provided in Table 1.
Table 1
Items | Specification |
---|---|
Date of search | 5 Nov 2022 |
Databases and other sources searched | Clinicaltrials.gov to identify the clinical research. The MEDLINE and EMBASE database were searched to identify any published results of the clinical trials included in this review |
Search terms used | “Leptomeningeal” under “condition or disease” |
Timeframe | From inception to 5 Nov 2022 |
Inclusion and exclusion criteria | Inclusion criteria: (I) clinical trials which are “not yet recruiting”, “recruiting”, “enrolling by invitation” or “active, not recruiting”, and (II) targeted at patient with LMD arising from a solid malignancy. Clinical trials of all phases and observation studies were included |
Studies unrelated to diagnostic or therapeutic intervention were excluded | |
Selection process | Search done by two independent reviewers (Chan AW and Wong HCY), who resolved any dispute by discussion and consensus |
LMD, leptomeningeal disease.
Clinical trials that were deemed to have (I) potentially promising efficacy, based on the efficacy endpoints in the published data; or (II) significant impact on the research directions of LMD by two independent reviewers (Chan AW and Wong HCY) were discussed in this review. These reviewers screened the results and resolved any disputes by discussion and consensus. When evaluating the impact of clinical trials on the future research directions of LMD, the reviewers assessed whether the clinical trials: (I) employed novel interventions that are distinct from those currently in use; (II) were built upon recent advances in translational research in LMD; or (III) could potentially overcome some of the bottlenecks in the management of LMD.
The general framework and methodology of this narrative review followed the recommendation by Ferrari (11).
Identification of trials
A summary of the search strategy and the reasons for the selection of clinical trials for discussion in this narrative review is described in Figure 1. The initial search yielded 44 clinical trials. Five of these 44 clinical trials did not meet our inclusion criteria because they were targeted at solid malignancies without LMD (n=3) or were targeted at non-malignant conditions of the leptomeninges (n=2). A total of 39 clinical trials met our inclusion criteria. One clinical trial was excluded as it was a survey related to telemedicine and deemed unrelated to diagnostic or therapeutic intervetion. A summary of the remaining 38 clinical trials is shown in Table 2.
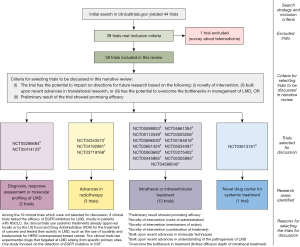
Table 2
NCT number | Title | Tumour histology | Phase# | Intervention |
---|---|---|---|---|
NCT05598853* | Intrathecal Double Checkpoint Inhibition (IT-IO) | NSCLC, melanoma | Phase I | IT nivolumab and IT ipilimumab |
NCT05414123* | A Therapy Treatment Response Trial in Patients With Leptomeningeal Metastases (LM) Using CNSide (FORESEE) | NSCLC, breast cancer | Observational | Not applicable |
NCT05385185 | Clinical Observation of ICI Combined With Recombinant Human Endostatin on Leptomeningeal Metastasis of Lung Cancer | Lung cancer | Phase II | IV camrelizumab or envafolimab and IV recombinant human vascular endostatin |
NCT05305885* | Intra-pemetrexed Alone or Combined With Concurrent Radiotherapy for Leptomeningeal Metastasis | Any solid tumour | Phase not reported | IT or ICV pemetrexed and radiotherapy |
NCT05289908 | Intrathecal Pemetrexed for Leptomeningeal Metastasis | Any solid tumour | Phase I/II | IT pemetrexed |
NCT05286684* | Feasibility of Exosome Analysis in Cerebrospinal Fluid During the Diagnostic Workup of Metastatic Meningitis (Exo-LCR) | Breast cancer | Phase not reported | Not applicable |
NCT05257967 | CSF Analysis in EGFR Mutant Non-Small Cell Lung Cancer With Leptomeningeal Disease | EGFR mutant NSCLC | Phase not reported | Not applicable |
NCT05184816* | A Study of Deferoxamine (DFO) in People With Leptomeningeal Metastasis | Any solid tumour/NSCLC | Phase I | IT deferoxamine |
NCT05146219 | Study of TY-9591 in Patients With a Lung Cancer With Brain or Leptomeningeal Metastases With EGFR Mutation | EGFR mutant NSCLC | Phase II | Oral TY-9591 (EGFR inhibitor) |
NCT05112549* | Intrathecal Application of PD1 Antibody in Metastatic Solid Tumors With Leptomeningeal Disease (IT-PD1/NOA 26) | Any solid tumour | Phase I | IT nivolumab |
NCT05063682* | The Efficacy and Safety of Brain-targeting Immune Cells (EGFRvIII-CAR T Cells) in Treating Patients With Leptomeningeal Disease From Glioblastoma. Administering Patients EGFRvIII-CAR T Cells May Help to Recognize and Destroy Brain Tumor Cells in Patients (CARTREMENDOUS) | EGFRvII+ glioblastoma multiforme | Phase I | ICV EGFRvIII-CAR T Cells |
NCT05034497* | Intraventricular Administration of Rhenium-186 NanoLiposome for Leptomeningeal Metastases (ReSPECT-LM) | Any solid tumour | Phase I | ICV Rhenium-186 |
NCT04944069 | Almonertinib With Bevacizumab for EGFR-Mutant NSCLC Patients With Leptomeningeal Metastasis | EGFR mutant NSCLC | Phase not reported | Oral almonertinib with IV bevacizumab |
NCT04833205 | Clinical Efficacy and Safety of EGFR-TKI Combined With Nimotuzumab in the Treatment of Leptomeningeal Metastases From Lung Cancer | EGFR mutant NSCLC | Phase II | IV nimotuzumab and oral third generation TKI |
NCT04778800 | A Dose Exploration Study of Almonertinib for EGFRm NSCLC Patients With Brain/Leptomeningeal Metastasis (ARTISTRY) | EGFR mutant NSCLC | Phase not reported | Oral almonertinib |
NCT04729348 | Pembrolizumab And Lenvatinib In Leptomeningeal Metastases | Any solid tumour | Phase II | IV pembrolizumab and oral lenvatinib |
NCT04661384* | Brain Tumor-Specific Immune Cells (IL13Ralpha2-CAR T Cells) for the Treatment of Leptomeningeal Glioblastoma, Ependymoma, or Medulloblastoma | Primary brain tumour | Phase I | ICV IL13Ralpha2-CAR T Cells |
NCT04588545* | Radiation Therapy Followed by Intrathecal Trastuzumab/Pertuzumab in HER2+ Breast Leptomeningeal Disease | HER2 positive breast cancer | Phase I/II | Radiotherapy and IT trastuzumab/pertuzumab |
NCT04563871 | Efficacy and Safety of 80mg Osimertinib in Patients With Non-small Cell Lung Cancer (NSCLC) | EGFR mutant NSCLC | Phase II | Oral osimertinib |
NCT04356222 | Efficacy and Safety of Durvalumab in Non-Small Cell Lung Cancer With Leptomeningeal Metastasis | NSCLC | Phase IV | IV durvalumab and IT methotrexate |
NCT04356118 | Efficacy and Safety of Recombinant Human Endostatin in Non-Small Cell Lung Cancer With Leptomeningeal Metastasis | NSCLC | Phase IV | Oral targeted drugs for NSCLC, IV recombinant human endostatin and IT methotrexate |
NCT04343573* | Proton Craniospinal Radiation Therapy vs. Partial Photon Radiation Therapy for Leptomeningeal Metastasis From Solid Tumors | NSCLC, breast cancer | Phase II | Proton craniospinal radiation |
NCT04233021 | Study of Osimertinib in Patients With a Lung Cancer With Brain or Leptomeningeal Metastases With EGFR Mutation | EGFR mutant NSCLC | Phase II | Oral osimertinib |
NCT04197934 | WSD0922-FU for the Treatment of Glioblastoma, Anaplastic Astrocytoma, or Non-small Cell Lung Cancer With Central Nervous System Metastases | IDH wild type primary brain tumour and NSCLC | Phase I | Oral EGFR/EGFRvIII Inhibitor WSD0922-FU |
NCT04192981* | GDC-0084 With Radiation Therapy for People With PIK3CA-Mutated Solid Tumor Brain Metastases or Leptomeningeal Metastases | Solid tumour harbouring PIK3CA mutations | Phase I | Oral GDC-0084 (PI3KCA inhibitor) and whole brain radiotherapy |
NCT03719768* | Avelumab With Radiotherapy in Patients With Leptomeningeal Disease | Any solid tumour | Phase I | IT avelumab and whole brain radiotherapy |
NCT03696030* | HER2-CAR T Cells in Treating Patients With Recurrent Brain or Leptomeningeal Metastases | HER2 positive breast cancer | Phase I | ICV HER2-CAR T Cells |
NCT03661424* | BATs in Patients With Breast Cancer and Leptomeningeal Metastases | Breast cancer | Phase I | ICV HER2 bi-specific antibody (HER2Bi) armed activated T-cells |
NCT03613181* | ANG1005 in Leptomeningeal Disease From Breast Cancer | HER2 negative breast cancer | Phase III | IV ANG 1005 (paclitaxel trevatide) |
NCT03520504 | Study of Proton Radiation to the Brain and Spinal Cord for Patients With Leptomeningeal Metastases | Any solid tumour | Phase IB | Proton craniospinal irradiation |
NCT03501979 | Tucatinib, Trastuzumab, and Capecitabine for the Treatment of HER2+ LMD | HER2 positive breast cancer | Phase II | Oral tucatinib, IV trastuzumab, and oral capecitabine |
NCT03423628 | A Study to Assess the Safety and Tolerability of AZD1390 Given With Radiation Therapy in Patients With Brain Cancer | High grade glioma | Phase I | Radiotherapy and oral AZD1390 (ATM kinase inhibitor) |
NCT03275402* | 131I-omburtamab Radioimmunotherapy for Neuroblastoma Central Nervous System/Leptomeningeal Metastases | Neuroblastoma | Phase II/III | ICV Iodine 131 omburtamab |
NCT03257124 | Study of AZD9291 in NSCLC Patients Harboring T790M Mutation Who Failed EGFR TKI and With Brain and/or LMS | EGFR mutant NSCLC | Phase II | Oral AZD9291 (osimertinib) |
NCT03025256* | Intravenous and Intrathecal Nivolumab in Treating Patients With Leptomeningeal Disease | NSCLC, melanoma | Phase I | IT and IV nivolumab |
NCT02422641 | Prospective Evaluation Of High-Dose Systemic Methotrexate In Patients With Breast Cancer And Leptomeningeal Metastasis | Breast cancer | Phase II | IT methotrexate |
NCT00445965* | Iodine I 131 Monoclonal Antibody 3F8 in Treating Patients With Central Nervous System Cancer or Leptomeningeal Cancer | Malignancy known to express GD2 | Phase II | IT Iodine 131 Monoclonal Antibody 3F8 |
NCT00089245 | Radiolabeled Monoclonal Antibody Therapy in Treating Patients With Refractory, Recurrent, or Advanced CNS or Leptomeningeal Cancer | Malignancy known to be 8H9 reactive | Phase I | IT Iodine 131 Omburtamab |
*, discussed in the narrative review. #, the clinical trials are interventional studies unless stated otherwise. NSCLC, non-small cell lung cancer; IT, intrathecal; CNS, central nervous system; ICI, immune checkpoint inhibitors; ICV, intracerebroventricular; CSF, cerebrospinal fluid; EGFR, epidermal growth factor receptor; IV, intravenous; TKI, tyrosine kinase inhibitor; HER2, human epidermal growth receptor 2; IDH, isocitrate dehydrogenase; LMD, leptomeningeal disease.
Nineteen clinical trials were selected by 2 co-authors reviewers to be discussed below. Four key areas of research were identified. They included: (I) diagnosis, response assessment or molecular profiling of LMD (n=2); (II) advances in radiotherapy (n=3); (III) intrathecal treatment (n=13); and (IV) novel drug carrier for systemic treatment (n=1). The two independent reviewers agreed on the selection of studies for discussion 90% of the time, and reached consensus in the remaining 10% after discussion. The phases of clinical trials, primary endpoints and enrolment number of the clinical trials selected for discussion were listed in Table 3. Of note, HRQoL was included as an endpoint in only two of the 19 studies selected for discussion, and in four of the 38 studies included in this review. The remaining 19 studies were not selected for discussion and the reasons for this are described in Figure 1.
Table 3
Number of trials | |
---|---|
Clinical trial phase | |
Phase 1 | 11 (57.9) |
Phase 2 or phase 1/2 | 3 (15.8) |
Phase 3 or phase 2/3 | 2 (10.5) |
Not reported | 3 (15.8) |
Primary endpoints* | |
Adverse events, maximum tolerated dose or dose for phase 2 | 13 (68.4) |
Overall survival | 7 (36.8) |
Response rate | 2 (10.5) |
Actual or estimated enrolment number# | 39 [28–50] |
Data are shown as n (%) or median [25th percentile–75th percentile]. *, some clinical trials included more than one primary endpoints. All of the primary endpoints were counted in this table. #, only three studies reported actual enrolment number, and the remaining reported estimated enrolment number.
Some of the studies included were still enrolling, whereas others had their preliminary results published. Our review discussed the methodology of these studies, as well as the early results whenever they were available.
Key findings
Diagnosis, response assessment or molecular profiling of LMD
Circulating tumour cells
The cytology examination of CSF is one of the cornerstones for the diagnosis of LMD. The detection of malignant cells in CSF allows for molecular testing, which in turn can be used to guide the management. However, even repeated CSF sampling by lumbar puncture for cytology has a sensitivity of up to 75% only (12).
Against this background, the FORESEE study (NCT05414123) aims to determine whether CNSide, a laboratory-developed test for circulating tumour cells detection, is more sensitive than cytology in detecting tumour cells in CSF (13). In this multi-centre, prospective observational study enrolling patients with breast cancer or NSCLC who have suspicious or confirmed LMD diagnosed by CSF cytology will be enrolled. The outcomes measured include the sensitivity of CNSide relative to cytology examination, the correlation of CNSide with clinical evaluation and MRI, and the impact of CNSide on clinical management, as measured by the proportion of decision points in which the physician indicated that CNSside aided in their decision-making. The detection of circulating tumour cells in CSF, which is based on the unique physical or biological properties of tumour cells, could be clinically significant for three reasons (14). First, its higher sensitivity than cytology may lead to an earlier diagnosis of confirmed LMD (15). Second, quantification of circulating tumour cells before and after treatment can aid in the response assessment (16). Third, isolation of circulating tumour cells may allow molecular testing which detects tumour heterogeneity. For instance, in metastatic HER2-amplified breast cancer, one of the standard first-line treatment for these patients is dual anti-HER2 antibodies in combination with chemotherapy (17). It has been shown that discordance between HER2 status in primary breast tumour and brain metastasis occurs in 14% of patients (18). Further research is needed to look at whether circulating tumour cells in CSF could detect discordant HER2 status between blood and CSF, and how it might influence the management of LMD in breast cancer.
Despite the potential advantages discussed above, there are some hurdles to overcome before the detection of circulating tumour cells in CSF could change clinical practice. For instance, there currently is wide variation in the techniques used to detect circulating tumour cells or cell free DNA (14). More research on the cut-off value and the standardization of the molecular techniques is needed, before they can be used to establish a diagnosis or monitor treatment response, especially when the measurements are done at different centres (19). Tumour cells can also persist in CSF of patients with stable or improved clinical or radiological features (1).
Exosome analysis
Other more innovative research using CSF in patients with LMD includes exosome analysis. One of these single-arm interventional trials (NCT05286684) aims to assess the correlation between proteomic profile of CSF by exosome analysis and cytology in patients with breast cancer and LMD (20). Exosomes are vesicles containing DNA, RNA and proteins, released by both normal and tumour cells into the surrounding biofluids, for intercellular communication (21). When combined with cell free DNA, exosome analysis has been shown to enhance the sensitivity of detection of EGFR mutation in blood (22). The potential utility of exosome in CSF for patients with LMD is less clear than those of circulating tumour cells or cell-free DNA at present.
Radiotherapy
Proton craniospinal irradiation
Radiotherapy is one of the mainstays of treatment for LMD. Because of the propensity of LMD to spread along the entire neuroaxis, a phase II randomized trial (NCT04343573) by the Memorial Sloan Kattering Cancer Center has compared craniospinal irradiation of the entire neuraxis 30 Gy in 10 fractions delivered with proton therapy to focal radiotherapy with photons in the treatment of LMD from solid malignancy (23). The recently published results showed that proton craniospinal irradiation improved CNS progression free survival (7.5 vs. 2.3 months, hazard ratio 0.15, P<0.001) and overall survival (9.9 vs. 6 months, hazard ratio 0.43, P=0.025) compared to local photon radiotherapy. No significant increase in grade 3 or above treatment-related adverse events were observed (P=0.19, 31 events in the craniospinal irradiation with proton group and 17 events in the focal radiotherapy with photon group) (24). HRQoL was not reported as one of the endpoints, but the assessment of patient reported outcome using the MD Anderson Symptom Inventory for Brain Tumor (MDASI-BT) and MD Anderson Symptom Inventory for Spinal Tumor (MDASI-SP) were the secondary objectives in the protocol of this phase 2 trial, and they have been reported in the result of the phase 1b trial by Yang et al. (25).
The outcomes with proton craniospinal irradiation in LMD is very promising, and its low toxicity is consistent with a previous report of proton craniospinal irradiation for adult patients with medulloblastoma (26). Nevertheless, there are several questions that remain to be answered. First, what is the best timing of craniospinal irradiation, especially when there could be drug treatments that are highly effective for CNS metastasis. The trial compared two techniques of irradiation with different irradiation fields and does not answer the question of the role or timing of radiotherapy in the management of LMD. For instance, lorlatinib, a third-generation ALK inhibitor, has been reported to have intracranial response rate of 71% in ALK positive NSCLC (27). In patients who are eligible for both proton craniospinal radiotherapy and targeted therapy with good CNS penetration, the optimal treatment sequence or potential benefit of concomitant use will need to be evaluated by further research. Second, focal stereotactic radiosurgery or radiotherapy has been suggested to be an effective treatment for bulky spinal disease or nodular LMD, which could arise after surgical resection of brain metastasis (12,28). How should focal stereotactic radiosurgery or radiotherapy be integrated with proton craniospinal irradiation in the treatment of nodular LMD or bulky spinal disease? Alternatively, can proton craniospinal irradiation supplant the need for stereotactic radiosurgery or radiotherapy through either its superior efficacy as it was delivered in the Memorial Sloan Kettering Cancer Center phase II trial (24) or by delivering an integrated or sequential boost to bulky disease beyond 30 Gy (cobalt Gray equivalent)? As the subgroup analysis of nodular and linear LMD in this craniospinal irradiation study are not reported, it remains unclear whether the benefit of proton craniospinal irradiation would differ according to subtype. Third, there are other indications for proton radiotherapy such as skull base tumours, low-grade glioma and paediatric tumours, while the capacity of proton treatment facility varies across the globe (29-32). Which group of patients can benefit the most from proton treatment? Fourth, how does craniospinal irradiation with photons and the volumetric modulated arc therapy (VMAT) technique compare with craniospinal irradiation with protons? These are the questions that remain to be answered.
Conventional radiotherapy in combination with pharmaceutical treatment
Two studies are looking at the combination of radiotherapy with drug treatments including oral dual phosphatidylinositol 3 kinase (PI3K)/mammalian target of rapamycin (mTOR) inhibitor (NCT04192981; phase I single-arm interventional study) (33) and intravenous programmed death-ligand 1 (PD-L1) inhibitor (NCT03719768; phase IB single-arm interventional study) (34). The former trial (NCT04192981) recruits patients with either brain metastasis or LMD, while the latter trial (NCT03719768) recruits only patients with LMD.
Reasons provided to support the role of radiotherapy include the following. First, the combination of radiotherapy with systemic treatment had been suggested to increase the blood-brain barrier permeability, which enhances the delivery of drugs to the site of LMD (35). Second, drugs administered systemically such as PI3K/mTOR inhibitors have been shown to be radiosensitizing (36). Third, the combined use of radiotherapy and immunotherapy could induce an abscopal effect, which is defined as regression of tumour outside the radiation field (37) radiotherapy can relieve sites of obstruction, restore CSF flow and facilitate distribution of the intrathecal drug when CSF flow blocks have been observed and when an intrathecal treatment is planned in a patient presenting with CSF blocks (38). As the combination of radiotherapy with pharmaceutical treatment does not require advanced radiation techniques or novel molecular diagnostic methods, it has the potential to be made widely available even in resource-limited settings.
Whether these potential advantages translate into clinically significant benefits for patients with LMD remains uncertain. A small study showed that in patients given intravenous trastuzumab and whole brain irradiation, the concentration of trastuzumab in CSF was only less than 2% of that in the serum, in patients with LMD, vs. <1% in patients with CNS metastases not treated by whole brain irradiation (39). In a recent systemic review of the effect of radiotherapy on the permeability of blood-brain barrier, as measured by techniques including MRI and liquid chromatography-mass spectrometry, only 35% of the included clinical studies reported disruption of blood-brain barrier following radiotherapy (40). More studies are needed to examine the impact of radiotherapy on the blood-brain barrier and whether it could influence the pharmacokinetics of systemic treatment.
Some LMD show contrast enhancement in MRI. It has been suggested that the contrast enhancement or extravasation in LMD in MRI already represents a breakdown of the blood-brain barrier, and systemic drugs should be able to reach the site of LMD just as well as the intravenously administered contrast (5). This could diminish the potential gain brought by the combination with radiotherapy if the blood-brain barrier is already impaired before radiotherapy. Regarding radiosensitizing agents, one of the challenges is to identify an agent that could be selectively taken up by tumour cells with relatively sparing of normal brain tissue (41). As for abscopal effect, the median time of onset of abscopal effect from concurrent use of radiotherapy and immunotherapy in one study was 5 months, which is longer than the historically expected median survival of 2–4 months in patients with melanoma or breast cancer with LMD (5,42). The combination of systemic treatment or intrathecal treatment and radiotherapy, especially with a large radiation field, may also increase the risk of toxicity.
There are two possible directions for future research with respect to the sequential use or combination of radiotherapy and drugs. First, the exact timing of radiotherapy may be important. A study using dynamic-contrast MRI brain showed that the blood-brain barrier permeability would increase at 2–4 weeks following radiotherapy, though data on the permeability beyond one month was not captured in this study (43). It should be recognized that treatment toxicities may be influenced by the timing of radiotherapy and drugs as well. For instance, leukoencephalopathy is much more likely to develop when whole brain irradiation is given before intravenous methotrexate, presumably because of the increased permeability of the brain to methotrexate induced by radiotherapy (44). Studies that explore the timing of systemic treatment relative to the completion of radiotherapy for LMD may provide insight into how to maximize the efficacy and minimize the toxicities of treatment. Second, the optimal radiotherapy dose to trigger abscopal effect continues to be an area of active research, and may help inform the focal radiation dose for LMD (45).
Intrathecal treatment
The goal is here to increase the drug concentration in the CSF with a minimal systemic toxicity. There are many clinical studies regarding the use of intrathecal treatment in LMD. They include agents targeting the microenvironment of CSF, cell-based immunotherapy, radiopharmaceutical and drugs already approved for systemic use.
Agent targeting the microenvironment of CSF
A phase 1A/B single-arm interventional trial (NCT05184816) is evaluating the use of intrathecal deferoxamine, an iron chelator, for the treatment of LMD (46). Research showed that CSF is relatively devoid of oxygen and micronutrients. Cancer cells in the CSF of patients with LMD were found to have upregulation of iron transport gene including lipocalin-2, in order to collect sparse extracellular iron from CSF (47). In the same study, it was found that lipocalin-2 could support tumour cell growth in the leptomeninges of mice, suggesting that uptake of iron could be important to the survival of tumour cells in LMD. Mice who had intra-cistern injection of iron chelator showed longer survival and suppressed growth of the tumour cells in leptomeninges. This forms the biological rationale for the use of deferoxamine in patients with LMD.
Other potential targets related to the specific microenvironment of CSF include the Complement 3, which is upregulated in LMD. Complement 3 is implicated in the disruption of blood-brain barrier, passage of tumour cells into the CSF space and alteration of CSF composition to promote cell growth (48). Recently published research in the metabolism of tumour cells in CSF, such as the role of gamma-aminobutyric acid (GABA) transaminase in medulloblastoma, may also identify new targets for intrathecal drug in LMD (49).
Radiopharmaceuticals
Several ongoing clinical studies [NCT05034497 (phase I interventional study), NCT03275402 (phase 2/3 single-arm interventional study), NCT00445965 (phase II single-arm interventional study)] aim to assess the safety and efficacy of intrathecal radiopharmaceutical therapy (RPT) for the treatment of LMD (50-52). Conventional chemotherapy or antibodies administrated by the intrathecal route have limited efficacy, partly because they can only penetrate the most superficial 2–3 mm of LMD (12). The beta radiation emitted by RPT, such as Iodine-131, could overcome this limitation and penetrate up to 8 mm of tumour tissue (53). At the same time, the depth of penetration of beta radiation also means that most radiopharmaecuticals can cause haematological toxicities, as a result of irradiation of the bone marrow, with the nadir cell count at 4–6 weeks post-administration (54).
It is worth noting that the intrathecal use of Iodine-131 was reported more than 30 years ago (55). However, the development of intrathecal or intraventricular RPT since then has been relatively stagnant, and there is currently no intrathecal or intraventricular RPT widely used for LMD. The slow progress of RPT in cancer treatment has been attributed to the often increased complexity of delivering and managing patients receiving RPT, concerns of radioactivity and the need for multidisciplinary effort for its successful implementation (53). The short shelf-life of RPT due to radioactive decay and its cost can also be barriers to its wider implementation (56). While some of these barriers could be difficult to overcome, a well-trained multidisciplinary team including physicians who are familiar with radionuclide handling, radiation safety and RPT administration is important for the wider use of RPT (54).
Cell-based immunotherapy
Three phase I single-arm interventional studies (NCT03696030, NCT05063682, NCT04661384 ) explored the intraventricular use of chimeric antigen receptor (CAR)-T cells, one of which targeted the HER2 receptor as the antigen (57-59). A CAR is a recombinant receptor designed to redirect T-cells to target tumour-specific antigen and mediates tumour cell killing. Another phase I single-arm interventional study (NCT03661424) used intraventricular bi-specific antibody armed activated T-cell for LMD (60). The endpoints included adverse events, objective response rate and HRQoL. The bi-specific antibody in this study was against CD-3 and HER2. Activated T-cell armed with this bi-specific antibody has been shown to exhibit high level of cytotoxicity against HER2 expressing breast cancer cells (61).
The use of cell-based immunotherapy in solid malignancies is not as well-established as in haematological malignancy, likely because of issues in tumour-target selection, T-cell migration and the tumour microenvironment (62). Almost all B-cell neoplasms express CD-19, which could be a target for CAR-T. In solid malignancy, selection of an antigen exclusively present in all tumour cells is much more difficult (63). Although the HER2 receptor in breast cancer cells may appear to be an attractive option, conversion of HER2 from positive to negative upon disease progression could occur in around 20% of cases, possibly reflecting the selective pressure exerted by anti-HER2 treatment (64). Loss of HER2 receptor in tumour cells might be associated with antigen-negative tumour relapse (65). This could diminish the efficacy of HER2-targeted cell-based immunotherapy for LMD and necessitate the checking of HER2 status of tumour cells in CSF if available.
Another issue of cell-based immunotherapy in solid malignancies is the requirement for T-cell migration. As the target cells of haematological malignancy reside in the blood, the T-cells can come to direct contact with the target cells once given intravenously. In most solid malignancies, the T-cell has to migrate to the tumour to become effective. Fortunately, the intraventricular route of administration in the three clinical trials discussed above could bypass this problem, as both the T-cell and tumour cells are located in the CSF space.
Lastly, the immunosuppressive microenvironment in most solid tumours can be hostile to T-cell (62). More research is needed to evaluate the microenvironment of CSF and explore whether concurrent intraventricular injection of other immunotherapy such as PD-L1 inhibitors can help overcome the immunosuppression (66).
Drugs already approved for systemic use
Studies in LMD have explored the intrathecal use of drugs whose intravenous use for advanced-stage cancer had already been approved, such as intrathecal nivolumab alone (NCT05112549; phase I single-arm interventional study), combined intrathecal and intravenous nivolumab (NCT03025256; phase I/IIb single-arm interventional study) and intrathecal nivolumab with ipilimumab (NCT05598853; phase I single-arm interventional study) (67-69). It has been suggested that intravenously administered checkpoint inhibitors may not be able to cross the blood-brain barrier because of its size (>140,00 Da) (70). These studies will address the questions of whether the intrathecal use could lead to better CNS efficacy and possibly to a lower incidence of systemic side effects. As the use of intravenous checkpoint inhibitors is already part of the standard treatment in many types of cancer (71), studies on the combined use of intravenous and intrathecal checkpoint inhibitors might be more clinically relevant than those which used intrathecal checkpoint inhibitors alone.
Other studies have used intrathecal pemetrexed (NCT05305885; open label randomized interventional study) (72) and intrathecal HER2 antibody (NCT04588545; phase I/II non-randomized interventional study) (73). The same research group that leads the NCT05305885-trial has published a phase I/II trial using intrathecal pemetrexed concurrent with focal radiotherapy for LMD (74). Sixty-two percent of the patients in this phase I/II study had NSCLC, and prior intravenous pemetrexed use was associated with a trend towards poorer response to intrathecal pemetrexed, as determined by the Response Assessment in Neuro-Oncology criteria. This could be explained by drug resistance induced by prior systemic pemetrexed, which is a standard first-line treatment for non-squamous NSCLC (75). Future studies may explore the efficacy of intrathecal agents that are not part of the standard systemic treatments for the cancer type in order to avoid the compromise of efficacy caused by prior systemic exposure.
Novel drug carrier in systemic treatment
Many ongoing clinical studies utilizing systemic treatment in LMD aim to assess the efficacy of drugs already approved for the treatment of cancer, such as tucatinib, osimertinib and durvalumab (Table 2). One phase III randomised trial (NCT03613181) is comparing ANG1005, an investigational chemotherapy-peptide conjugate, to physician’s best choice in patients with LMD or brain metastasis (76). The primary outcome is overall survival. ANG1005 is a compound consisting of three paclitaxel molecules linked to Angiopep-2, which was designed to help cross the blood-brain barrier through interaction with the lipoprotein receptor-related protein 1 (LRP1) on the surface of endothelial cells of the blood-brain barrier (77)
In the published ANG1005 phase II trial, the intracranial response rate in patients with breast cancer and LMD, diagnosed on imaging criteria only, was 29% (77). The fact that 68% of patients with LMD in this study had Karnofsky Performance Status score of 80 or above may cast some doubt upon the generalizability of these findings. Nevertheless, the encouraging early results have already led to studies investigating the linkage of Angiopep-2 and other drugs such as lapatinib (78). This direction of combining drugs with novel carriers that can cross the blood-brain barrier will likely be an area of active research in the years to come (79).
Limitations
Our narrative review has several limitations. First, in order to balance the depth of analysis and breadth of coverage, we deliberately chose not to discuss all of the clinical studies in LMD that fulfilled our inclusion criteria. Second, the findings of most of the clinical studies included in this review have not been published, in neither abstract nor full paper. Our understanding of their research questions and methodology were based on the brief record in clinicaltrials.gov, which may not be a complete representation of the study design or the investigator’s idea of the research question. Third, as our review focused on ongoing clinical research, studies that have recently been registered as “completed” in clinicaltrials.gov are intentionally not included in our review. We acknowledge that some of these studies might also have promising results and significant impact on future research direction. Nevertheless, these typically have already been discussed in other recently published review articles regarding the current state of management in LMD. While the published review articles in the advances in LMD mostly focused on primary site specific treatment approaches (8-10), our review discusses diagnostic approach or treatment principle which can be applied to LMD regardless of the primary site or presence of a particular biomarker.
Knowledge gap
Four broad areas of knowledge gap were identified by this narrative review. First, more research is needed in the risk stratification or prognostic tools for the survival in LMD. On one hand, as discussed above, the emergence of novel molecular techniques may diagnosis LMD at an earlier stage, leading to longer survival times either because of earlier treatment or lead time bias. On the other hand, the prognosis of some patients with LMD may be so poor that they would not benefit from intensive treatment. Le Rhun et al. have shown that the European Association of Neuro-Oncology (EANO) and European Society of Medical Oncology (ESMO) classification of LMD types and MRI findings are highly prognostic, in that patients with positive CSF cytology or nodular pattern on MRI had poorer survival than patients with negative CSF cytology and linear pattern on MRI (80). As noted by the authors of this prognostic validation study, their cohorts of patients were not treated in more recent years where novel targeted therapy has been available. It was shown in the same prognostic validation study that there was a significant difference in the survival time of patients with lung cancer, breast cancer and melanoma as well. A prognostic tool in patients with LMD that takes into account the cancer type, functional status as measured by the Karnofsky Performance Status Scale or other methods, neurocognitive functioning, molecular markers and novel diagnostic technology such as circulating tumour cells in CSF may help us select the appropriate clinical trial or treatment for this group of patients. Clinical trials whose eligibility criteria are based on the factors that included in such prognostic tool could answer questions such as whether whole brain irradiation is beneficial for patients with poor-risk LMD. The importance of careful patient selection for whole brain irradiation was illustrated by a recent retrospective review of patients with LMD who received whole brain irradiation (81). In this study, more than half of the patients with headache, dizziness or nausea caused by LMD showed improvement after whole brain irradiation, while those with depressed level of consciousness or seizures did not. Among the 22 patients with LMD, only one developed grade 3 dizziness after whole brain irradiation. There were no other grade 3 or worse acute adverse events.
Second, more research in the cost-effectiveness analysis of proton therapy and other costly treatments in LMD is needed (82). The possible outcomes of interest can include overall survival and outcomes related to the patients’ functioning and wellbeing. Such research would allow clinicians to provide the best possible care for patients with LMD in spite of resource constraints.
Third, when considering the effectiveness of treatment, the impact on both survival and the patients functioning and wellbeing needs to be taken into account. Functioning and wellbeing is typically measured with HRQoL questionnaires. There are few ongoing randomized trials in LMD and most of the early phase studies in LMD do not use HRQoL as an endpoint. The incorporation of HRQoL as the endpoint in future phase 2 studies in LMD will provide insight into the impact of new treatments, their side effects and the burden of any diagnostic investigations on the HRQoL of patients with LMD. Besides, there are currently no HRQoL assessment tools that have been validated for use in patients with LMD. Some studies have used HRQoL instruments designed for primary brain tumours, such as the EORTC-QLQ Brain Neoplasm (BN20) (83) and the MDASI-BT (25,84). Although symptoms of brain tumours and LMD may overlap to some degree, there are substantial differences as well. For instance, symptoms attributable to lower cranial nerve palsy and spinal cord or nerve root involvement by tumour are common in LMD but rare for primary brain tumours (85,86). A HRQoL instrument validated in patients with LMD is needed to assess the impact of treatment. This is especially important for patients whose symptoms may be out of proportion with their radiological findings. In these patients, assessing the changes in functioning and wellbeing over time could aid response assessment in clinical trials as well. Until a dedicated tool to measure HRQoL outcomes in LMD has been developed, investigators should be encouraged to utilize tools that cover as much relevant domains for LMD patients as possible, such as the MDASI-BT, MDASI-SP, EORTC QLQ-BN20, or other tools.
Fourth, the optimal sequence of treatment for LMD remains unknown. Multi-disciplinary collaboration is critical to manage patients with LMD. Most of the studies included in this review focused on specific interventions, such as radiation therapy, systemic therapy or intrathecal therapy. There is limited evidence to date on how to sequence these treatment modalities to achieve the best outcome.
Conclusions
Ongoing clinical research in the diagnosis, response assessment or molecular profiling of LMD, radiotherapy, intrathecal treatment and novel drug carrier for systemic treatment have the potential to significantly improve the historically poor prognosis of patients with LMD. Future development may focus on integration of novel molecular techniques into the diagnosis of LMD, combination of radiotherapy and pharmaceutical treatment, better understanding of the tumour microenvironment in LMD, implementation of intrathecal or intraventricular RPT with a multi-disciplinary approach and cell-based immunotherapy. The knowledge gaps that need to be addressed include prognostic tools for overall survival in LMD, cost-effectiveness studies of new treatments, the development of tools to reliably measure the functioning and wellbeing of patients with LMD and sequencing of the treatment modalities
Acknowledgments
Funding: None.
Footnote
Reporting Checklist: The authors have completed the Narrative Review reporting checklist. Available at https://apm.amegroups.com/article/view/10.21037/apm-23-390/rc
Peer Review File: Available at https://apm.amegroups.com/article/view/10.21037/apm-23-390/prf
Conflicts of Interest: All authors have completed the ICMJE uniform disclosure form (available at https://apm.amegroups.com/article/view/10.21037/apm-23-390/coif). E.O. serves as an unpaid editorial board member of Annals of Palliative Medicine from December 2022 to November 2024. E.C. serves as an unpaid editorial board member of Annals of Palliative Medicine from February 2022 to January 2024. C.B.S. II is Editor-in-Chief of Annals of Palliative Medicine. MT is a member of the Scientific Advisory Board Dutch Cancer Society (KWF Kankerbestrijding) and chair of the Grant Review Committee EORTC Quality of Life Group, RANO (response assessment in neuro-oncology)-PRO (patient reported outcomes) committee and EANO Publishing Committee. E.L.R reports personal financial interests as an advisory board member for Astra Zeneca, Bayer, Janssen, Leo Pharma, Pierre Fabre, Seattle Genetics and Servier; institutional funding from Bristol Meyers Squibb (BMS), and is currently the EORTC BTG secretary. The other authors have no conflicts of interest to declare.
Ethical Statement: The authors are accountable for all aspects of the work in ensuring that questions related to the accuracy or integrity of any part of the work are appropriately investigated and resolved.
Open Access Statement: This is an Open Access article distributed in accordance with the Creative Commons Attribution-NonCommercial-NoDerivs 4.0 International License (CC BY-NC-ND 4.0), which permits the non-commercial replication and distribution of the article with the strict proviso that no changes or edits are made and the original work is properly cited (including links to both the formal publication through the relevant DOI and the license). See: https://creativecommons.org/licenses/by-nc-nd/4.0/.
References
- Le Rhun E, Weller M, Brandsma D, et al. EANO-ESMO Clinical Practice Guidelines for diagnosis, treatment and follow-up of patients with leptomeningeal metastasis from solid tumours. Ann Oncol 2017;28:iv84-99. [Crossref] [PubMed]
- Nayar G, Ejikeme T, Chongsathidkiet P, et al. Leptomeningeal disease: current diagnostic and therapeutic strategies. Oncotarget 2017;8:73312-28. [Crossref] [PubMed]
- Hyun JW, Jeong IH, Joung A, et al. Leptomeningeal metastasis: Clinical experience of 519 cases. Eur J Cancer 2016;56:107-14. [Crossref] [PubMed]
- Le Rhun E, Taillibert S, Chamberlain MC. Carcinomatous meningitis: Leptomeningeal metastases in solid tumors. Surg Neurol Int 2013;4:S265-88. [Crossref] [PubMed]
- Le Rhun E, Preusser M, van den Bent M, et al. How we treat patients with leptomeningeal metastases. ESMO Open 2019;4:e000507. [Crossref] [PubMed]
- Lukas RV. Leptomeningeal metastases-What outcomes should we measure and how? Neuro Oncol 2022;24:1736-7. [Crossref] [PubMed]
- Hofer S, Le Rhun E. Leptomeningeal metastases from solid tumours. memo - Magazine of European Medical Oncology 2021;14:192-7. [Crossref]
- Sharma A, Low JT, Kumthekar P. Advances in the Diagnosis and Treatment of Leptomeningeal Disease. Curr Neurol Neurosci Rep 2022;22:413-25. [Crossref] [PubMed]
- Wilcox JA, Li MJ, Boire AA. Leptomeningeal Metastases: New Opportunities in the Modern Era. Neurotherapeutics 2022;19:1782-98. [Crossref] [PubMed]
- Lukas RV, Thakkar JP, Cristofanilli M, et al. Leptomeningeal metastases: the future is now. J Neurooncol 2022;156:443-52. [Crossref] [PubMed]
- Ferrari R. Writing narrative style literature reviews. Med Writ 2015;24:230-5. [Crossref]
- Nguyen TK, Nguyen EK, Soliman H. An overview of leptomeningeal disease. Ann Palliat Med 2021;10:909-22. [Crossref] [PubMed]
- Biocept, Inc. A Longitudinal Therapy Response Monitoring Study in Subjects With Leptomeningeal Metastases Using CNSide (CSF Tumor Cells) Compared to Standard of Care (CSF Cytology, Clinical Evaluation, and Imaging). clinicaltrials.gov; 2022. Accessed November 16, 2022. Available online: https://clinicaltrials.gov/ct2/show/NCT05414123
- Lin D, Shen L, Luo M, et al. Circulating tumor cells: biology and clinical significance. Signal Transduct Target Ther 2021;6:404. [Crossref] [PubMed]
- Lin X, Fleisher M, Rosenblum M, et al. Cerebrospinal fluid circulating tumor cells: a novel tool to diagnose leptomeningeal metastases from epithelial tumors. Neuro Oncol 2017;19:1248-54. [Crossref] [PubMed]
- Malani R, Fleisher M, Kumthekar P, et al. Cerebrospinal fluid circulating tumor cells as a quantifiable measurement of leptomeningeal metastases in patients with HER2 positive cancer. J Neurooncol 2020;148:599-606. [Crossref] [PubMed]
- Swain SM, Baselga J, Kim SB, et al. Pertuzumab, trastuzumab, and docetaxel in HER2-positive metastatic breast cancer. N Engl J Med 2015;372:724-34. [Crossref] [PubMed]
- Duchnowska R, Dziadziuszko R, Trojanowski T, et al. Conversion of epidermal growth factor receptor 2 and hormone receptor expression in breast cancer metastases to the brain. Breast Cancer Res 2012;14:R119. [Crossref] [PubMed]
- Thery L, Meddis A, Cabel L, et al. Circulating Tumor Cells in Early Breast Cancer. JNCI Cancer Spectr 2019;3:pkz026. [Crossref] [PubMed]
- Centre Oscar Lambret. Feasibility of Exosome Analysis in Cerebrospinal Fluid During the Diagnostic Workup of Metastatic Meningitis From Breast Cancer. clinicaltrials.gov; 2022. Accessed November 16, 2022. Available online: https://clinicaltrials.gov/ct2/show/NCT05286684
- Yu W, Hurley J, Roberts D, et al. Exosome-based liquid biopsies in cancer: opportunities and challenges. Ann Oncol 2021;32:466-77. [Crossref] [PubMed]
- Krug AK, Enderle D, Karlovich C, et al. Improved EGFR mutation detection using combined exosomal RNA and circulating tumor DNA in NSCLC patient plasma. Ann Oncol 2018;29:2143. [Crossref] [PubMed]
- Memorial Sloan Kettering Cancer Center. A Phase II Randomized Study Assessing the Efficacy of Proton Craniospinal Irradiation (CSI) vs Involved-Field Photon Radiation Therapy for Leptomeningeal Metastases From Solid Tumor Malignancies. clinicaltrials.gov; 2022. Accessed November 16, 2022. Available online: https://clinicaltrials.gov/ct2/show/NCT04343573
- Yang JT, Wijetunga NA, Pentsova E, et al. Randomized Phase II Trial of Proton Craniospinal Irradiation Versus Photon Involved-Field Radiotherapy for Patients With Solid Tumor Leptomeningeal Metastasis. J Clin Oncol 2022;40:3858-67. [Crossref] [PubMed]
- Yang TJ, Wijetunga NA, Yamada J, et al. Clinical trial of proton craniospinal irradiation for leptomeningeal metastases. Neuro Oncol 2021;23:134-43. [Crossref] [PubMed]
- Brown AP, Barney CL, Grosshans DR, et al. Proton beam craniospinal irradiation reduces acute toxicity for adults with medulloblastoma. Int J Radiat Oncol Biol Phys 2013;86:277-84. [Crossref] [PubMed]
- Solomon BJ, Bauer TM, Ignatius Ou SH, et al. Post Hoc Analysis of Lorlatinib Intracranial Efficacy and Safety in Patients With ALK-Positive Advanced Non-Small-Cell Lung Cancer From the Phase III CROWN Study. J Clin Oncol 2022;40:3593-602. [Crossref] [PubMed]
- Prabhu RS, Turner BE, Asher AL, et al. A multi-institutional analysis of presentation and outcomes for leptomeningeal disease recurrence after surgical resection and radiosurgery for brain metastases. Neuro Oncol 2019;21:1049-59. [Crossref] [PubMed]
- Journy N, Indelicato DJ, Withrow DR, et al. Patterns of proton therapy use in pediatric cancer management in 2016: An international survey. Radiother Oncol 2019;132:155-61. [Crossref] [PubMed]
- Mercado CE, Holtzman AL, Rotondo R, et al. Proton therapy for skull base tumors: A review of clinical outcomes for chordomas and chondrosarcomas. Head Neck 2019;41:536-41. [Crossref] [PubMed]
- Grau C, Durante M, Georg D, et al. Particle therapy in Europe. Mol Oncol 2020;14:1492-9. [Crossref] [PubMed]
- Maillie L, Lazarev S, Simone CB 2nd, et al. Geospatial Disparities in Access to Proton Therapy in the Continental United States. Cancer Invest 2021;39:582-8. [Crossref] [PubMed]
- Memorial Sloan Kettering Cancer Center. A Phase I Study With Expansion Cohort of Concurrent GDC-0084 With Radiation Therapy for Patients With Solid Tumor Brain Metastases or Leptomeningeal Metastases Harboring PI3K Pathway Mutations. clinicaltrials.gov; 2022. Accessed November 16, 2022. Available online: https://clinicaltrials.gov/ct2/show/NCT04192981
- H. Lee Moffitt Cancer Center and Research Institute. Phase IB Study of Avelumab With Radiotherapy in Patients With Leptomeningeal Disease. clinicaltrials.gov; 2022. Accessed November 16, 2022. Available online: https://clinicaltrials.gov/ct2/show/NCT03719768
- Mo F, Pellerino A, Soffietti R, et al. Blood-Brain Barrier in Brain Tumors: Biology and Clinical Relevance. Int J Mol Sci 2021;22:12654. [Crossref] [PubMed]
- Yu CC, Hung SK, Lin HY, et al. Targeting the PI3K/AKT/mTOR signaling pathway as an effectively radiosensitizing strategy for treating human oral squamous cell carcinoma in vitro and in vivo. Oncotarget 2017;8:68641-53. [Crossref] [PubMed]
- Wang J, Tawbi HA. Emergent immunotherapy approaches for brain metastases. Neurooncol Adv 2021;3:v43-51. [Crossref] [PubMed]
- Chamberlain MC. Radioisotope CSF flow studies in leptomeningeal metastases. J Neurooncol 1998;38:135-40. [Crossref] [PubMed]
- Stemmler HJ, Schmitt M, Harbeck N, et al. Application of intrathecal trastuzumab (Herceptintrade mark) for treatment of meningeal carcinomatosis in HER2-overexpressing metastatic breast cancer. Oncol Rep 2006;15:1373-7. [PubMed]
- Hart E, Odé Z, Derieppe MPP, et al. Blood-brain barrier permeability following conventional photon radiotherapy - A systematic review and meta-analysis of clinical and preclinical studies. Clin Transl Radiat Oncol 2022;35:44-55. [Crossref] [PubMed]
- Pepper NB, Stummer W, Eich HT. The use of radiosensitizing agents in the therapy of glioblastoma multiforme-a comprehensive review. Strahlenther Onkol 2022;198:507-26. [Crossref] [PubMed]
- Reynders K, Illidge T, Siva S, et al. The abscopal effect of local radiotherapy: using immunotherapy to make a rare event clinically relevant. Cancer Treat Rev 2015;41:503-10. [Crossref] [PubMed]
- Teng F, Tsien CI, Lawrence TS, et al. Blood-tumor barrier opening changes in brain metastases from pre to one-month post radiation therapy. Radiother Oncol 2017;125:89-93. [Crossref] [PubMed]
- Gowan GM, Herrington JD, Simonetta AB. Methotrexate-induced toxic leukoencephalopathy. Pharmacotherapy 2002;22:1183-7. [Crossref] [PubMed]
- Poleszczuk J, Enderling H. The Optimal Radiation Dose to Induce Robust Systemic Anti-Tumor Immunity. Int J Mol Sci 2018;19:3377. [Crossref] [PubMed]
- Memorial Sloan Kettering Cancer Center. A Phase 1a/1b Trial of Intrathecal Deferoxamine for Leptomeningeal Metastases. clinicaltrials.gov; 2022. Accessed November 17, 2022. Available online: https://clinicaltrials.gov/ct2/show/NCT05184816
- Chi Y, Remsik J, Kiseliovas V, et al. Cancer cells deploy lipocalin-2 to collect limiting iron in leptomeningeal metastasis. Science 2020;369:276-82. [Crossref] [PubMed]
- Boire A, Zou Y, Shieh J, et al. Complement Component 3 Adapts the Cerebrospinal Fluid for Leptomeningeal Metastasis. Cell 2017;168:1101-1113.e13. [Crossref] [PubMed]
- Martirosian V, Deshpande K, Zhou H, et al. Medulloblastoma uses GABA transaminase to survive in the cerebrospinal fluid microenvironment and promote leptomeningeal dissemination. Cell Rep 2021;35:109302. [Crossref] [PubMed]
- Y-mAbs Therapeutics. A Multicenter Phase 2/3 Trial of the Efficacy and Safety of Intracerebroventricular Radioimmunotherapy Using 131I-Omburtamab for Neuroblastoma Central Nervous System/Leptomeningeal Metastases. clinicaltrials.gov; 2021. Accessed November 17, 2022. Available online: https://clinicaltrials.gov/ct2/show/NCT03275402
- Plus Therapeutics. A Multicenter Phase 1 Clinical Study to Determine the Maximum Tolerated Dose/Maximum Feasible Dose, Safety,& Efficacy of Single Dose Rhenium-186 NanoLiposome (186RNL) Administered Via Intraventricular Catheter for Leptomeningeal Metastases. clinicaltrials.gov; 2022. Accessed November 17, 2022. Available online: https://clinicaltrials.gov/ct2/show/NCT05034497
- Memorial Sloan Kettering Cancer Center. Phase II Study of Intrathecal I-3F8 in Patients With GD2-Expressing Central Nervous System and Leptomeningeal Neoplasms. clinicaltrials.gov; 2022. Accessed November 17, 2022. Available online: https://clinicaltrials.gov/ct2/show/NCT00445965
- Sgouros G, Bodei L, McDevitt MR, et al. Radiopharmaceutical therapy in cancer: clinical advances and challenges. Nat Rev Drug Discov 2020;19:589-608. [Crossref] [PubMed]
- O'Brien SR, Pryma DA. Introduction to Radiopharmaceutical Therapy. Pract Radiat Oncol 2022;12:285-8. [Crossref] [PubMed]
- Lashford LS, Davies AG, Richardson RB, et al. A pilot study of 131I monoclonal antibodies in the therapy of leptomeningeal tumors. Cancer 1988;61:857-68. [Crossref] [PubMed]
- Damasceno A, Pijeira MSO, Ricci-Junior E, et al. Exploiting the extemporaneousness of radiopharmaceuticals: Radiolabeling stability under diverse conditions. J Pharm Biomed Anal 2022;221:115024. [Crossref] [PubMed]
- City of Hope Medical Center. A Phase 1 Study to Evaluate IL13Rα2-Targeted Chimeric Antigen Receptor (CAR) T Cells for Adult Patients With Leptomeningeal Glioblastoma, Ependymoma or Medulloblastoma. clinicaltrials.gov; 2022. Accessed November 17, 2022. Available online: https://clinicaltrials.gov/ct2/show/NCT04661384
- City of Hope Medical Center. A Phase 1 Cellular Immunotherapy Study of Intraventricularly Administered Autologous HER2-Targeted Chimeric Antigen Receptor (HER2-CAR) T Cells in Patients With Brain and/or Leptomeningeal Metastases From HER2 Positive Cancers. clinicaltrials.gov; 2022. Accessed November 17, 2022. Available online: https://clinicaltrials.gov/ct2/show/NCT03696030
- Chembrain LTD. A Phase 1 Study to Evaluate EGFRvIII -Targeted Chimeric Antigen Receptor (CAR) T Cells for Adult Patients With Leptomeningeal Glioblastoma. clinicaltrials.gov; 2021. Accessed November 17, 2022. Available online: https://clinicaltrials.gov/ct2/show/NCT05063682
- Fadul CE. A Phase I Study of Anti-CD3 x Anti-Her2/Neu (Her2Bi) Armed Activated T Cells (ATC) in Patients With Breast Cancer Leptomeningeal Metastases. clinicaltrials.gov; 2021. Accessed November 17, 2022. Available online: https://clinicaltrials.gov/ct2/show/NCT03661424
- Lum LG, Thakur A, Al-Kadhimi Z, et al. Targeted T-cell Therapy in Stage IV Breast Cancer: A Phase I Clinical Trial. Clin Cancer Res 2015;21:2305-14. [Crossref] [PubMed]
- Qian S, Villarejo-Campos P, Guijo I, et al. Update for Advance CAR-T Therapy in Solid Tumors, Clinical Application in Peritoneal Carcinomatosis From Colorectal Cancer and Future Prospects. Front Immunol 2022;13:841425. [Crossref] [PubMed]
- McGranahan N, Swanton C. Clonal Heterogeneity and Tumor Evolution: Past, Present, and the Future. Cell 2017;168:613-28. [Crossref] [PubMed]
- Schrijver WAME, Suijkerbuijk KPM, van Gils CH, et al. Receptor Conversion in Distant Breast Cancer Metastases: A Systematic Review and Meta-analysis. J Natl Cancer Inst 2018;110:568-80. [Crossref] [PubMed]
- Shah NN, Fry TJ. Mechanisms of resistance to CAR T cell therapy. Nat Rev Clin Oncol 2019;16:372-85. [Crossref] [PubMed]
- Grosser R, Cherkassky L, Chintala N, et al. Combination Immunotherapy with CAR T Cells and Checkpoint Blockade for the Treatment of Solid Tumors. Cancer Cell 2019;36:471-82. [Crossref] [PubMed]
- University of Zurich. Intrathecal Administration of Anti-PD1/Anti-CTLA-4 in Combination With Systemic Combination of Anti-PD1/Anti-CTLA-4 in Patients With NSCLC Without Oncogenic Driver Mutation or Melanoma and Newly Diagnosed Leptomeningeal Metastasis: A Multicentric Phase I Study. clinicaltrials.gov; 2022. Accessed November 17, 2022. Available online: https://clinicaltrials.gov/ct2/show/NCT05598853
- M.D. Anderson Cancer Center. Phase I/Ib Study of Concurrent Intravenous and Intrathecal Nivolumab for Melanoma and Lung Cancer Patients With Leptomeningeal Disease (LMD). clinicaltrials.gov; 2022. Accessed November 17, 2022. Available online: https://clinicaltrials.gov/ct2/show/NCT03025256
- University Hospital Tuebingen. Intrathecal Application of PD1 Antibody in Metastatic Solid Tumors With Leptomeningeal Disease (IT-PD1/ NOA 26). clinicaltrials.gov; 2022. Accessed November 9, 2022. Available online: https://clinicaltrials.gov/ct2/show/NCT05112549
- Huppert LA, Melisko ME, Glastonbury CM, et al. Treatment of Metastatic Melanoma With Leptomeningeal Disease Using Intrathecal Immunotherapy. JCO Oncol Pract 2020;16:757-9. [Crossref] [PubMed]
- Larroquette M, Domblides C, Lefort F, et al. Combining immune checkpoint inhibitors with chemotherapy in advanced solid tumours: A review. Eur J Cancer 2021; Epub ahead of print. [Crossref] [PubMed]
- Pan Z. An Open-Label, Randomized, Multicenter Trial of Intrathecal-Pemetrexed Combined With Concurrent Involved-Field Radiotherapy and Intrathecal-Pemetrexed Alone in Patients With Leptomeningeal Metastasis From Solid Tumors. clinicaltrials.gov; 2022. Accessed November 16, 2022. Available online: https://clinicaltrials.gov/ct2/show/NCT05305885
- H. Lee Moffitt Cancer Center and Research Institute. Phase I/II Study of Radiation Therapy Followed by Intrathecal Trastuzumab/Pertuzumab in the Management of HER2+ Breast Leptomeningeal Disease. clinicaltrials.gov; 2022. Accessed November 9, 2022. Available online: https://clinicaltrials.gov/ct2/show/NCT04588545
- Pan Z, Yang G, He H, et al. Intrathecal pemetrexed combined with involved-field radiotherapy as a first-line intra-CSF therapy for leptomeningeal metastases from solid tumors: a phase I/II study. Ther Adv Med Oncol 2020;12:1758835920937953. [Crossref] [PubMed]
- Gandhi L, Rodríguez-Abreu D, Gadgeel S, et al. Pembrolizumab plus Chemotherapy in Metastatic Non-Small-Cell Lung Cancer. N Engl J Med 2018;378:2078-92. [Crossref] [PubMed]
- Angiochem Inc. A Randomized Open-Label, Multi-Center Pivotal Study of ANG1005 Compared With Physician’s Best Choice in HER2-Negative Breast Cancer Patients With Newly Diagnosed Leptomeningeal Carcinomatosis and Previously Treated Brain Metastases (ANGLeD). clinicaltrials.gov; 2022. Accessed November 24, 2022. Available online: https://clinicaltrials.gov/ct2/show/NCT03613181
- Kumthekar P, Tang SC, Brenner AJ, et al. ANG1005, a Brain-Penetrating Peptide-Drug Conjugate, Shows Activity in Patients with Breast Cancer with Leptomeningeal Carcinomatosis and Recurrent Brain Metastases. Clin Cancer Res 2020;26:2789-99. [Crossref] [PubMed]
- Lu H, Chen T, Wang Y, et al. Dual targeting micelles loaded with paclitaxel and lapatinib for combinational therapy of brain metastases from breast cancer. Sci Rep 2022;12:2610. [Crossref] [PubMed]
- Habib S, Singh M. Angiopep-2-Modified Nanoparticles for Brain-Directed Delivery of Therapeutics: A Review. Polymers (Basel) 2022;14:712. [Crossref] [PubMed]
- Le Rhun E, Devos P, Weller J, et al. Prognostic validation and clinical implications of the EANO ESMO classification of leptomeningeal metastasis from solid tumors. Neuro Oncol 2021;23:1100-12. [Crossref] [PubMed]
- Hirano Y, Konishi K, Ejima Y. Utility of whole brain radiation therapy for leptomeningeal carcinomatosis. Int J Clin Oncol 2020;25:1432-9. [Crossref] [PubMed]
- Verma V, Shah C, Rwigema JC, et al. Cost-comparativeness of proton versus photon therapy. Chin Clin Oncol 2016;5:56. [Crossref] [PubMed]
- Taphoorn MJ, Claassens L, Aaronson NK, et al. An international validation study of the EORTC brain cancer module (EORTC QLQ-BN20) for assessing health-related quality of life and symptoms in brain cancer patients. Eur J Cancer 2010;46:1033-40. [Crossref] [PubMed]
- Armstrong TS, Mendoza T, Gning I, et al. Validation of the M.D. Anderson Symptom Inventory Brain Tumor Module (MDASI-BT). J Neurooncol 2006;80:27-35. [Crossref] [PubMed]
- Powell J. Symptomatic Spinal Metastases from Intracranial High-grade Glioma – Report of Four Cases and Review of the Literature. Eur Oncol Haematol 2011;7:179-82. [Crossref]
- Mabray MC, Glastonbury CM, Mamlouk MD, et al. Direct Cranial Nerve Involvement by Gliomas: Case Series and Review of the Literature. AJNR Am J Neuroradiol 2015;36:1349-54. [Crossref] [PubMed]