Palliating hematologic oncologic emergencies with radiation in the age of targeted systemic therapies: three illustrative cases
Introduction
In radiation oncology, oncologic emergencies with an indication for urgent radiation are a routine part of practice. Hematologic malignancies make up a potentially large subset of these emergencies. These patients are admitted often, and imaged often. Hematologic cancers may involve nodal conglomerates or extranodal sites, and due to their rapid growth can quickly compromise bone integrity, cause cord compression, or exert symptomatic mass effect. Medical oncologists who appreciate the exquisite radiosensitivity of hematologic malignancies will consult radiation oncologists urgently and frequently.
These cases require a nuanced approach with consideration of the present emergent condition and overarching trajectory of the disease course. There have been significant recent advances in hematologic malignancy treatments, with multiple new lines of targeted therapy now available. Patients are living longer as they have multiple treatment options for their relapsed or refractory diseases (1-3). While essential to treat the emergency at hand, care should be taken to ensure treatment is tailored, allowing for future therapies. In addition to consulting with disease site experts, treatment decisions can be aided by various cancer treatment guidelines, such as the National Comprehensive Cancer Network (NCCN), European Society for Medical Oncology (ESMO), or the Children’s Oncology Group (COG). Dedicated radiation oncology and hematologic-specific guidelines are also available, notably in the form of guidelines papers from the International Lymphoma Radiation Oncology Group (ILROG) and the American Radium Society (ARS) Appropriate Use Criteria. Understanding whether a patient is purely palliative versus potentially curative is difficult to know in this era. Patients who had poor prognoses in the past can now be turned around if they can make it to systemic therapies that offer the potential for durable remission. Here, we present three cases that underline specific issues faced by a radiation oncologist in a hematologic oncologic emergency, and discuss approaches and references to aid in treatment.
Case description
A case of plasmacytoma causing cord compression
We highlight a case of cord compression leading to a new cancer diagnosis, with question of solitary plasmacytoma versus multiple myeloma at initial workup. A 56-year-old otherwise healthy gentleman presented to the emergency department with several months of thoracic back pain, and 1 week of diminished sensation below his umbilicus and gait ataxia. Spine magnetic resonance imaging (MRI) showed a contrast enhancing lesion concerning for malignancy at T4 involving the bilateral posterior elements with anterior extension into the vertebral body causing spinal cord effacement (Figure 1). Additional imaging revealed no other sites of disease. The on-call radiation oncology and neurosurgery teams were consulted and favored initial surgical intervention to obtain a tissue diagnosis and for decompression. High-dose steroids were administered promptly after obtaining tissue, which returned as a cyclin-D1 positive plasmacytoma with kappa restriction. Complete workup was ordered including serum and urine electrophoresis and bone marrow biopsy, and post-operative radiation was planned.
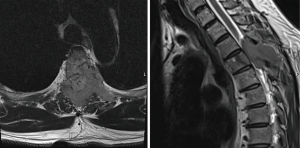
Diagnosis of plasmacytoma versus multiple myeloma was considered. As the full body positron emission tomography/computed tomography (PET/CT) scan showed no definite evidence of hypermetabolic neoplasm elsewhere, radiation was initially planned to 40 Gy in 20 fractions with a volumetric modified arc therapy plan, giving him the benefit of the doubt that this could be solitary bone plasmacytoma. The planning target volume (PTV) included the involved 4th thoracic vertebrae, the surgically manipulated spine (T2–T6) and all associated hardware. During radiation planning, bone marrow biopsy returned with 10–15% plasma cell neoplasm, meeting criteria for multiple myeloma. The prescription was adjusted to 30 Gy in 15 fractions (Figure 2). After radiation, Dara-VRd (velcade, decadron, lenalidomide) was initiated, with discussion of future consolidation of remission with melphalan, autologous stem cell transplant, and lenalidomide with or without daratumumab maintenance therapy.
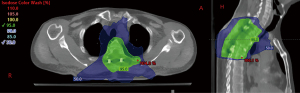
Plasma cell neoplasms are characterized by abnormal clonal plasma cells in bone marrow. Ten percent of patients with plasma cell neoplasms present with solitary plasmacytoma whereas 90% present with multiple myeloma. Treatment paradigms differ between the two disease states, as does radiation dose. For solitary plasmacytoma, radiation alone is recommended, with systemic therapy reserved for progression to multiple myeloma (4-6). Similar to NCCN, the ILROG guidelines recommend doses of 35–40 Gy for bone lesions ≤5 cm, 40–50 Gy for bone lesions >5 cm, and 40–50 Gy for solitary extramedullary plasmacytomas (4,5,7,8). While radiation alone for solitary plasmacytoma offers chance for cure, the rate of progression to multiple myeloma is 65–84% with bone disease and 10–30% with extranodal disease at ten years (4,9-12).
Multiple myeloma is considered a systemic disease where systemic therapy is the crux of treatment—radiation plays a purely palliative role. Like many hematologic malignancies, multiple myeloma has seen advances in treatment options. Patients often experience periods of disease control with eventual progression, proceeding from one line of treatment to the next. Standard first-line induction therapy is velcade, lenalidomide, and dexamethasone. Maintenance therapy with velcade or lenalidomide can be considered, as well as autologous hematopoietic stem cell transplant in eligible patients. Many therapies may be considered upon relapse depending on patient and disease specific factors: chemotherapy, immunomodulatory agents, proteasome inhibitors, and CAR-T cell therapy, among others (3,13-17). When radiation is utilized (often for local control, analgesia, and/or bone stability), drug-radiation interplay must be considered. Recent retrospective data suggest concurrent therapy is generally safe. Resende Salgado et al. noted no significant difference in hematologic or nonhematologic toxicities in patients treated concurrently with radiation and biologic agents (bortezomib, carfilzomib, daratumumab) compared to radiation alone, and reported no high grade toxicities in either group (18). Oertel et al. described a broader range of systemic agents and noted a higher rate of high grade toxicities (specifically leukocytopenia) in the concurrent treatment group post-radiation (19). However, on analysis by substance class, only concurrent proteasome inhibitors had increased high grade hematologic toxicity post-radiation (notable for thrombocytopenia). There was no difference in non-hematologic toxicity between groups. Additionally, timing of therapies should be discussed, as trial eligibility can be impacted by timing of radiation: many studies require a radiation ‘washout’ time (20-23).
Bone marrow is compromised at the outset for myeloma patients, and risk of infection is elevated. Autologous stem cell transplant and other next line therapies require adequate bone marrow function—marrow function thresholds are typically incorporated into clinical trial eligibility criteria (20,24-27). Effort should be made to reduce radiation dose and field size to spare bone marrow. In the previously cited study, Oertel et al. reported that radiation to 5 or more bone lesions led to increased thrombocytopenia and leukocytopenia during radiotherapy (RT) (19). We opt to restrict doses for multiple myeloma to <30 Gy, as Casamassima et al. showed marrow recovery at doses <30 Gy, and rare recovery with doses >36 Gy (28). More recent evidence suggests marrow suppression <20 Gy (29). It is valuable for radiation oncologists to have a sense of how much functional marrow resides in different bony targets. Campbell et al. published a useful atlas estimating relative bone marrow function using [18F]fluorothymidine (FLT)-PET, with the highest functional marrow in the ‘pelvis’, followed by ‘ribs, clavicles and scapulae’, ‘thoracic spine’, and ‘lumbar spine’, respectively, according to FLT tracer uptake (30). Twenty gray in 10 fractions is reasonable for patients with sufficient life expectancy, and 25–30 Gy/10–15 fractions for bulky disease or cord compression necessitating durable local control (4,31,32). Eight gray in 1 fraction is reasonable for patients with poor prognoses (4,33). MD Anderson Cancer Center (MDACC) published a large, single institution series of 772 myeloma patients, and 1,513 irradiated lesions with the most common regimens as follows: 2 Gy × 10 [27.3%; biologically effective dose (BED) =24 Gy10], 2 Gy × 12 (13.8%; BED =28.8 Gy10), 2.5 Gy × 8 (8.9%; BED =25 Gy10), and 2.5 Gy × 10 (18.3%; BED =31.25 Gy10) (31). Nearly all patients had durable pain control. Only 2.6% required reirradiation, and all reirradiated patients had successful palliation of symptoms. BED <28 Gy was associated with increased risk of reirradiation. In a smaller series of 205 lesions, Meredith et al. reported 11 lesions requiring reirradiation, with a trend toward better pain control and less risk of retreatment with BED ≥24 Gy (34). In an effort to decrease dose and spare marrow suppression further, an active ILROG trial is investigating 2 Gy × 2 fractions (amended to also include 4 Gy × 1 fraction) for pain palliation (35). Taken together, there remains a long-term benefit in dose reduction to spare marrow, however, there may be a threshold BED required for durable and effective palliation of symptoms. Depending on the clinical scenario, image guidance with smaller PTV margins may be justified. Thus, consideration at consult is given to both diagnosis and treatment plan to ensure the patient is receiving appropriate definitive vs palliative treatment, as well as to ensure the radiation plan does not compromise eligibility for and tolerability of future systemic treatments.
Specific scenarios occurring frequently in the myeloma population warrant additional discussion. Frank cord compression, particularly with acute neurologic deficits, is treated with surgical intervention and post-operative radiation (36). In their phase II randomized study, Gillespie et al. showed that prophylactic radiation to asymptomatic bone metastases compared to observation resulted in fewer skeletal-related events, fewer hospitalizations, and increased overall survival (OS), the effect of which was most notable in the spinal lesion cohort (37). As plasma cells are radiosensitive and bone marrow preservation is paramount, dose reduction to spinal lesions is tempting, though there is understandable hesitancy as local failure is potentially devastating. Sharma et al. published their retrospective experience on 95 patients with newly diagnosed multiple myeloma and spinal involvement who underwent radiation to their spinal disease (38). Sixty-eight percent had pathologic compression fractures, and 15% had cord compression. Common radiation regimens were 20 Gy/5 fractions, followed by 30 Gy/10–12 fractions, and 8 Gy/1 fraction. Six patients received re-irradiation (indication not given, initial radiation regimen not specified). In the aforementioned MDACC series, there were 82 spinal lesions receiving radiation (most common regimens 20 Gy/10 fractions, BED10 24, and 24 Gy/12 fractions, BED10 28.8) (31). There were 10 local failures. Bilsky Grade 3 cord compression and paraspinal extension >5 mm were associated with increased risk of local failure. In such cases of notable epidural or paraspinal extension, dose escalation may be warranted, as has generally been our practice.
Lytic osseous lesions requiring surgical fixation are frequently encountered in patients with myeloma. Post-operative radiation field size—whether to cover all surgical hardware—remains a topic of debate with limited data. Elhammali published a small series of 33 patients who underwent surgical fixation of long bone lesions followed by radiation (most common regimens: 20–25 Gy/8–12 fractions) (39). There were 5 local failures (2 in-field, 3 out-of-field), with no relapses resulting in hardware failure. In a larger series out of Memorial Sloan Kettering, 138 patients with 145 long bone lesions received post-operative radiation (median dose 30 Gy, median follow-up 30 months) (40). There were 36 local recurrences. On univariate analysis, whole hardware coverage and higher BED (≥39 Gy) were associated with lower risk of local recurrence; propensity matched score analysis confirmed statistical significance of hardware coverage. Our practice in myeloma and other histologies generally includes coverage of all hardware, as hardware failure from local recurrence (though a small risk) would have significant patient impact, likely resulting in another procedure. When additional stabilizing hardware is used that clearly did not traverse the cancerous lesion, we often do not further extend the field.
A case of life-threatening cutaneous T-cell lymphoma
Here we describe a case featuring the palliative role of total skin radiation. Our patient carried a diagnosis of mycosis fungoides with initial disease limited to large cutaneous plaques at initial diagnosis 6 years prior. He had been treated with topical and skin-directed therapies, including clobetasol ointment and narrowband ultraviolet B phototherapy (nbUVB), with relatively good disease control. Unfortunately, he was incarcerated for 6 months without access to specialized medical care. During that time disease progressed to widespread ulcerated tumors, many bleeding and purulent (Figure 3). He was sleeping on vinyl covers and wrapping himself in plastic wrap to prevent clothing from adhering to his skin. Pain and pruritus were uncontrolled. He struggled to work, sleep, and complete activities of daily living secondary to symptoms. He had lost weight and endorsed significant bilateral lower extremity edema.
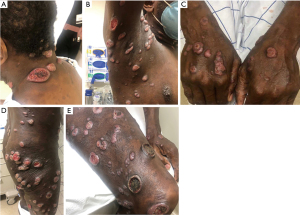
When he returned to dermatology, skin biopsy was performed along with PET/CT given his disease progression (Figure 4). Imaging showed widespread lymph node uptake and abnormal splenic uptake, and biopsy showed CD30-positive mycosis fungoides with large cell transformation (a histopathologic finding defined by ≥25% of large cells in the dermal infiltrate or the presence of microscopic nodules of large cells, overall associated with a more aggressive disease course and poorer prognosis) (41). Wound care was arranged. Skin and blood cultures grew Staphylococcus aureus, and antibiotics along with gabapentin for symptom control were initiated. He was referred to radiation and medical oncology.
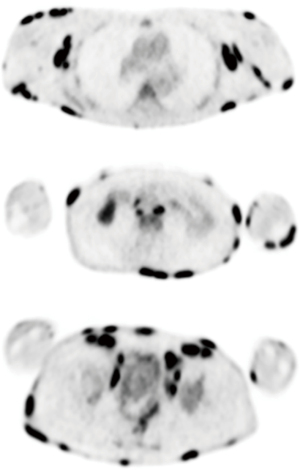
He presented to our outpatient clinic with continued severe pain, pruritus, fevers, and a pungent odor associated with the wounds. He had self-discontinued antibiotics due to an adverse reaction. We advised emergency department presentation for symptom management and infection control, however, he declined. We initiated urgent total skin irradiation, with goal of cutaneous disease regression thus palliating his pain, pruritus, and continued risk of infection. Concurrent systemic therapy was recommended given likely systemic involvement and widespread, severe cutaneous disease. Medical oncology opted to initiate single-agent brentuximab, as there was concern that multi-agent chemotherapy would pose further infection risk. He received total skin electron beam therapy (TSEBT) to 12 Gy, 1 Gy/fraction over the course of 3 weeks with boosts to the perineum (7 Gy) and soles (5 Gy). He experienced marked regression of his cutaneous tumors at the end of radiation, and symptoms slowly improved with treatment. One month post TSEBT, he noted sustained cutaneous improvement, lack of significant pain or itching, weight stabilization, and resolution of edema. No sites required focal boosts. He continued on brentuximab monotherapy every 3 weeks.
Many patients, for various reasons, have gaps in care. This was underscored during the COVID pandemic. Patients with cutaneous T-cell lymphoma may re-present with fulminant disease that has progressed unchecked without therapy. Progression ranges from widespread symptomatic skin plaques, to cutaneous tumors with open wounds posing risk of infection, to lymph node and blood involvement. TSEBT is a highly effective palliative treatment for widespread, symptomatic cutaneous disease. There has been a shift toward lower dose regimens (10–15 Gy in 1–2 Gy fractions compared to historical 36 Gy) that result in good response rates (~90%) with fewer side effects. These low dose regimens allow for additional skin radiation if needed and can be supplemented by topical and skin directed therapies (psoralen with ultraviolet light therapy—PUVA, nbUVB) (42,43). TSEBT is niche treatment that benefits from an experienced radiation oncology team—physicians, therapists, physicists, and dosimetrists included –to ensure optimal dose distribution and appropriate shielding of sensitive structures. Input from a well-practiced provider has benefits as the tolerance of the skin to TSEBT is dose-limited, and focal electron therapy is better suited for symptomatic lesions when disease is otherwise well-controlled.
Mycosis fungoides follows a long, waxing and waning course, necessitating careful timing of therapeutic agents to balance toxicity, skin tolerance, co-morbidities, and quality of life. Treatments range from topical therapies (steroids, retinoids, nitrogen mustard), to skin directed therapy, interferon, oral retinoids, systemic chemotherapies, immunotherapy, radiation (total skin and focal), and other investigative therapies (44). Involvement with a multidisciplinary team, including dermatology and medical oncology, optimizes treatment. Palliative radiation can be integrated into the treatment course. Focal radiation is highly effective, and can be repeated many times. Moderately low doses (4 Gy × 2, 8 Gy × 1) result in 77–92% response rates (45). We typically will treat up to 4 lesions at a time, generally limited by patients’ cooperation and tolerance of extended sessions as well as machine scheduling. Depending on the number and spatial relationship of lesions, several lesions can be treated serially. At some point, it becomes impractical to use focal radiation, and TSEBT may be a more optimal technique. With low dose TSEBT, particularly thick lesions or shadowed regions may require boosting. However, we generally find that with time (up to 3 months), even thick lesions can respond obviating the need for boost. Re-irradiation for CTCL is common, and we combine courses of focal radiation before and after TSEBT routinely, as is typically required over the years.
Recognizing that many centers do not have TSEBT, systemic treatment may be initiated with focal RT concurrently or sequentially for severe disease. In our experience, disease is slow to respond to systemic therapy and TSEBT achieves relatively fast control (on the order of several weeks) of diffuse, severe skin disease. TSEBT may be a tool worth developing in more centers. If unable to perform TSEBT, typical systemic agents used at our institution include brentuximab, pembrolizumab, romidepsin, pralatrexate, and mogamulizumab. PUVA and nbUVB are alternative skin-directed therapies (46,47). Both therapies show good overall response rates (ORRs) (>85%) with higher complete response rates utilizing PUVA (48,49). PUVA is considered more efficacious than nbUVB for thicker lesions, folliculotropic mycosis fungoides, and darker skin color (49). In our experience, both nbUVB and PUVA therapy take longer to achieve their full effect than radiation and are not as effective treating thick lesions. Furthermore, availability of PUVA in the outside community is sparse and has decreased in recent years, contributed to by the advent of effective biologics for more prevalent cutaneous diseases for which PUVA had been used (e.g., psoriasis and eczema), and poor financial reimbursement.
As with other hematologic cancers in which systemic therapy factors into treatment, timing of RT and its concurrent use warrants consideration. Methotrexate has long been documented as a radiation sensitizer, with known added neurotoxicity when given with RT to the brain (50). Pertinent to CTCL, there are case reports of radiation recall dermatitis (51). As pralatrexate shares a similar mechanism of action, we typically avoid concurrent administration with radiation. Bhangoo et al. reported the first documented case of radiation recall dermatitis after pralatrexate initiation (52). Among topical therapies, we ask patients to hold alkylating agents (e.g., nitrogen mustard) given the potential of additive skin toxicity, and radiation alone is highly effective at targeting the cutaneous lesions in question.
Another consideration when pursuing palliative radiation for CTCL is allogeneic stem cell transplant candidacy. In potential candidates, TSEBT and/or total body irradiation may be used as part of transplant conditioning (53). When TSEBT is used in transplant conditioning, doses are generally higher than the aforementioned palliative regimen, necessitating advanced planning. Close communication with dermatology and medical oncology is required for optimal treatment.
A case of bridging palliative radiation combined with CAR T-cell therapy
Our third case features the emerging role of radiation in the relapsed/refractory setting as a bridge to CAR-T therapy. Our patient carried a diagnosis of stage IV non-germinal center (GC) diffuse large B-cell lymphoma (DLBCL) with over-expression of BCL2 and C-MYC, International Prognostic Index (IPI) score 4, and central nervous system (CNS) IPI score 4 [age, stage, lactate dehydrogenase (LDH), extranodal sites]. He underwent six cycles rituximab, cyclophosphamide, doxorubicin, vincristine, prednisone (R-CHOP) (with methotrexate added in cycles 2–4 for CNS prophylaxis). End of treatment PET 8 weeks post treatment showed overall response except one area of progressive disease with increase in size and FDG avidity involving the right axilla (Deauville score 5). Axillary biopsy confirmed aggressive B-cell lymphoma. He underwent two cycles cisplatin-dexamethasone-gemcitabine-rituximab, and post treatment PET/CT showed persistent disease in the axilla. Anti-CD19 CAR-T therapy with tisagenlecleucel was planned, and apheresis completed. One week later, the patient felt rapid growth of the axillary mass, and radiation oncology was asked to palliate this mass prior to CAR-T cell infusion. On exam, the mass was easily palpable and painless, but the patient had pain and numbness in the right shoulder due to compression of the brachial plexus. The mass measured 5.8 cm × 6.0 cm × 11 cm on the planning CT (Figure 5). He underwent urgent radiation (42 Gy in 14 fractions) prior to CAR-T infusion. The gross tumor volume (GTV) was contoured and included the visualized mass on CT. Clinical target volume encompassing areas at high risk for disease involvement was the same as the GTV in this case. PTV was a 5 mm expansion from the GTV. 3D dynamic arc therapy was used, though technique varies by case and institutional preference. Factoring in the larger size of the mass and solitary site of macroscopic active disease, a higher dose was used. He had no radiation or CAR-T related toxicities including cytokine release syndrome, and was progression-free 1 year later.
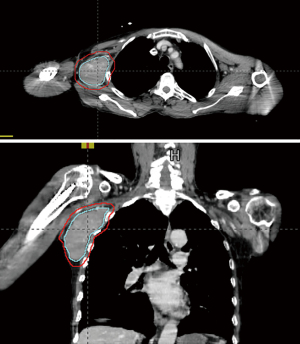
While many patients with DLBCL remain disease-free after standard R-CHOP with or without consolidative RT, 10–15% will have primary refractory disease, and 20–25% relapse (54). For these patients, salvage treatment with second-line chemotherapy followed by autologous stem-cell transplant has been the typical standard of care if performance status permits, however, progression-free survival (PFS) is less than 50% at three years (55-57). CAR-T therapy is an emerging treatment for relapsed/refractory disease. CAR-T is an adoptive therapy where autologous T-cells are collected (leukapheresis) and engineered to express receptor proteins that bind to target proteins on cancer cells—the CD-19 antigen in DLBCL. The engineered T-cells are then infused following lymphodepleting conditioning chemotherapy. Often, patients have rapid disease progression heading into or after leukapheresis. Radiation therapy “bridging” is an effective way to control disease progression and palliate symptoms.
For patients eligible for their next line of therapy (whether CAR-T or one of many next line options) with disease requiring palliative radiation, it is important to discuss with medical oncology so that radiation does not compromise the overarching treatment plan. Depending on the agent, systemic therapy may be held during radiation to prevent increased risk of toxicity (58-62). There are limited data on the safety of novel agents used concurrently with radiation. At our institution, we have noted increased high grade neutropenia with concurrent rather than sequential ibrutinib (63). The radiation prescription may affect the time a patient is not receiving systemic therapy. For patients with relapsed/refractory disease who are not transplant candidates and have limited life expectancy, an ILROG guideline recommends doses of 8–30 Gy in a hypofractionated regimen, though higher doses in standard fractionation may be used for bulky disease or when intent is potentially curative (64). Based on single institution retrospective data, conventional versus hypo-fractionation in palliation for relapsed/refractory DLBCL resulted in no difference in ORRs, time to local failure (TTLF) or acute toxicity (stratified by biological equivalent dose). Importantly, an EQD2 ≥35 Gy was associated with a higher ORR and TTLF (65).
At present, there is no consensus on the best dose, fractionation, and volume for CAR-T bridging patients. For those referred for palliation of symptoms, improving CAR-T response with bridging radiation is an added possibility. Factors associated with CAR-T failure include increased tumor burden, advanced stage disease, poor performance status, high IPI score, and 2+ sites of extranodal disease (66). Bridging radiation has been shown to significantly improve risk factors predictive of progression—namely metabolic tumor volume (MTV), maximum standardized uptake value (SUV), diameter, and LDH (67). Further, patients with low MTV pre-radiation compared to those who only achieved a low MTV post-radiation had similar PFS and OS (67). Utilizing ablative doses aims for durable local control, reduction in MTV, as well as palliation. Sim et al. reported 100% local control in lesions treated to an EQD2 >39 Gy (68). When higher doses are not feasible (such as with extensive fields, organ at risk (OAR) constraints, or patient specific factors), preclinical data suggest that very low doses of ’sensitizing’ radiation (1–3 Gy) to a comprehensive volume may increase CAR-T efficacy (69). The volume to encompass remains an area of study—whether to comprehensively treat all sites of disease or just treat bulky or symptomatic sites. While data are limited, a retrospective series out of MDACC showed a trend toward improved PFS and OS in patients treated comprehensively (70). Our approach has been to treat comprehensively when disease is reasonably limited within a radiation field.
The element of time comes into play as the manufacturing period and planned infusion can set a “clock” for when the bridging radiation is completed—about 3–4 weeks from apheresis to infusion. The sequence preferred by our group as well as other experts is to administer radiation after apheresis so as not to kill lymphocytes needed for harvesting, and prior to infusion to avoid radiating the CAR-T product and blunting response (66,71). This limited window combined with late referrals, necessitates flexibility in fractionation schemes, and often leads to a hypofractionated approach. This does raise concern regarding late effects of hypofractionation in a population that can achieve durable remissions (72-74). Our approach is to pursue standard fractionation when time and clinical context permit (e.g., good performance status, limited disease, patient willingness), but will adapt a hypofractionated scheme with adequate biological equivalent dose when needed (65,68).
Importantly, special “respect” should be given to the lymphocytes of patients with non-Hodgkin lymphomas as their role in various therapies grows, including CAR-T therapy and bi-specific antibodies. Depending on the dose, volume, and site treated, lymphocyte count can decrease secondary to radiation, which affects eligibility for particular systemic therapies, clinical trials and ability to collect or mobilize T-cells (75-80).
Several recent expert reviews delve into greater nuance and are excellent resources in the current role of bridging radiation in CAR-T therapy (66,71). The growing complexity of multiple lines of therapy for patients with non-Hodgkin lymphoma again speaks to the importance of multidisciplinary decision making and consulting updated guidelines or expert reviews, even in the “palliative” setting.
Conclusions
We are in an era of cancer medicine where multiple next line therapies exist for various hematologic malignancies and cancer is handled akin to a chronic disease. New treatments are constantly being developed. Patient care requires a multidisciplinary approach with good communication between all treating teams. It becomes essential that our palliative interventions do not burn bridges for future therapy eligibility. As cancer therapies have become so tailored within disease sites, it can be difficult for radiation oncologists who have not specialized in hematologic malignancies to be well versed in the latest practice recommendations.
The three distinct cases presented represent a snapshot of the varied hematologic palliative emergencies we encounter. In these cases, our patients had additional systemic therapies that could be considered, offering potential remission. Intervention for the hematologic emergency in a presenting patient is of course of prime importance. However, tailored treatment has long-term benefit and the potential to prolong survival by additional months to years, as patients continue on next line therapies, palliated as needed—or potentially cured.
Acknowledgments
Funding: None.
Footnote
Provenance and Peer Review: This article was commissioned by the Guest Editors (Isabella Choi, Stephanie Schaub, Simon S. Lo and Charles Simone) for the series “Radiotherapy for Oncologic Emergencies” published in Annals of Palliative Medicine. The article has undergone external peer review.
Peer Review File: Available at https://apm.amegroups.com/article/view/10.21037/apm-23-238/prf
Conflicts of Interest: All authors have completed the ICMJE uniform disclosure form (available at https://apm.amegroups.com/article/view/10.21037/apm-23-238/coif). The series “Radiotherapy for Oncologic Emergencies” was commissioned by the editorial office without any funding or sponsorship. The authors have no other conflicts of interest to declare.
Ethical Statement: The authors are accountable for all aspects of the work in ensuring that questions related to the accuracy or integrity of any part of the work are appropriately investigated and resolved. All procedures performed in this study were in accordance with the ethical standards of the institutional and national research committee(s) and with the Helsinki Declaration (as revised in 2013). Publication of this study was waived from patient consent according to the University of Pennsylvania institutional review board.
Open Access Statement: This is an Open Access article distributed in accordance with the Creative Commons Attribution-NonCommercial-NoDerivs 4.0 International License (CC BY-NC-ND 4.0), which permits the non-commercial replication and distribution of the article with the strict proviso that no changes or edits are made and the original work is properly cited (including links to both the formal publication through the relevant DOI and the license). See: https://creativecommons.org/licenses/by-nc-nd/4.0/.
References
- Wang L, Li LR, Young KH. New agents and regimens for diffuse large B cell lymphoma. J Hematol Oncol 2020;13:175. [Crossref] [PubMed]
- Kaufman AE, Patel K, Goyal K, et al. Mycosis fungoides: developments in incidence, treatment and survival. J Eur Acad Dermatol Venereol 2020;34:2288-94. [Crossref] [PubMed]
- Rajkumar SV. Multiple myeloma: Every year a new standard? Hematol Oncol 2019;37:62-5. [Crossref] [PubMed]
- Tsang RW, Campbell BA, Goda JS, et al. Radiation Therapy for Solitary Plasmacytoma and Multiple Myeloma: Guidelines From the International Lymphoma Radiation Oncology Group. Int J Radiat Oncol Biol Phys 2018;101:794-808. [Crossref] [PubMed]
- National Comprehensive Cancer Network. Multiple Myeloma (Version 5.2022). Accessed September 2, 2022. Available online: https://www.nccn.org/login?ReturnURL=https://www.nccn.org/professionals/physician_gls/pdf/myeloma.pdf
- International Lymphoma Radiation Oncology Group. Guidelines. 2023 [cited 2023 Jan 16]. Available online: https://www.ilrog.org/guidelines
- Tsang RW, Gospodarowicz MK, Pintilie M, et al. Solitary plasmacytoma treated with radiotherapy: impact of tumor size on outcome. Int J Radiat Oncol Biol Phys 2001;50:113-20. [Crossref] [PubMed]
- Zhu Q, Zou X, You R, et al. Establishment of an innovative staging system for extramedullary plasmacytoma. BMC Cancer 2016;16:777. [Crossref] [PubMed]
- Ozsahin M, Tsang RW, Poortmans P, et al. Outcomes and patterns of failure in solitary plasmacytoma: a multicenter Rare Cancer Network study of 258 patients. Int J Radiat Oncol Biol Phys 2006;64:210-7. [Crossref] [PubMed]
- Reed V, Shah J, Medeiros LJ, et al. Solitary plasmacytomas: outcome and prognostic factors after definitive radiation therapy. Cancer 2011;117:4468-74. [Crossref] [PubMed]
- Alexiou C, Kau RJ, Dietzfelbinger H, et al. Extramedullary plasmacytoma: tumor occurrence and therapeutic concepts. Cancer 1999;85:2305-14. [Crossref] [PubMed]
- Sasaki R, Yasuda K, Abe E, et al. Multi-institutional analysis of solitary extramedullary plasmacytoma of the head and neck treated with curative radiotherapy. Int J Radiat Oncol Biol Phys 2012;82:626-34. [Crossref] [PubMed]
- Weisel K, Hopkins TG, Fecteau D, et al. Dreamm-3: a phase 3, open-label, randomized study to evaluate the efficacy and safety of belantamab mafodotin (GSK2857916) monotherapy compared with pomalidomide plus low-dose dexamethasone (Pom/Dex) in participants with relapsed/refractory multiple myeloma (RRMM). Blood 2019;134:1900. [Crossref]
- Munshi NC, Anderson LD Jr, Shah N, et al. Idecabtagene vicleucel (ide-cel; bb2121), a BCMA-targeted CAR T-cell therapy, in patients with relapsed and refractory multiple myeloma (RRMM): Initial KarMMa results. J Clin Oncol 2020;38:8503. [Crossref]
- Berdeja JG, Madduri D, Usmani SZ, et al. Update of CARTITUDE-1: A phase Ib/II study of JNJ-4528, a B-cell maturation antigen (BCMA)-directed CAR-T-cell therapy, in relapsed/refractory multiple myeloma. J Clin Oncol 2020;38:8505. [Crossref]
- Mailankody S, Jakubowiak AJ, Htut M, et al. Orvacabtagene autoleucel (orva-cel), a B-cell maturation antigen (BCMA)-directed CAR T cell therapy for patients (pts) with relapsed/refractory multiple myeloma (RRMM): update of the phase 1/2 EVOLVE study (NCT03430011). J Clin Oncol 2020;38:8504. [Crossref]
- Lin Y, Raje NS, Berdeja JG, et al. Idecabtagene vicleucel (ide-cel, bb2121), a BCMA-directed CAR T cell therapy, in patients with relapsed and refractory multiple myeloma: updated results from phase 1 CRB-401 study. Blood 2020;136:26-7. [Crossref]
- Resende Salgado L, Wang S, Adler A, et al. The Safety Profile of Concurrent Therapy for Multiple Myeloma in the Modern Era. Adv Radiat Oncol 2018;4:112-7. [Crossref] [PubMed]
- Oertel M, Schlusemann T, Shumilov E, et al. Radiotherapy in Combination with Systemic Therapy for Multiple Myeloma-A Critical Toxicity Evaluation in the Modern Treatment Era. Cancers (Basel) 2023;15:2909. [Crossref] [PubMed]
- Atrash S. Selinexor, Daratumumab, Carfilzomib and Dexamethasone for the Treatment of High-Risk, Recurrent or Refractory Multiple Myeloma. ClinicalTrials.gov identifier: NCT04756401. Updated May 25, 2023. Accessed July 5, 2023. Available online: https://classic.clinicaltrials.gov/ct2/show/NCT04756401
- Qazilbash MH. Daratumumab in Treating Participants With Relapsed Multiple Myeloma After Stem Cell Transplant. ClinicalTrials.gov identifier: NCT03622775. Updated September 30, 2022. Accessed July 5, 2023. Available online: https://classic.clinicaltrials.gov/ct2/show/NCT03622775
- Lin Y. Pembrolizumab, Ixazomib Citrate, and Dexamethasone in Treating Patients With Relapsed Multiple Myeloma. ClinicalTrials.gov identifier: NCT03506360. Updated May 6, 2023. Accessed July 5, 2023. Available online: https://classic.clinicaltrials.gov/ct2/show/NCT03506360
- Krishnan A. Ixazomib Plus Pomalidomide and Dexamethasone in Treating Patients With Relapsed or Relapsed/Refractory Multiple Myeloma. ClinicalTrials.gov identifier: NCT02119468. Updated June 15, 2023. Accessed July 5, 2023. Available online: https://classic.clinicaltrials.gov/ct2/show/NCT02119468
- Al Hamed R, Bazarbachi AH, Malard F, et al. Current status of autologous stem cell transplantation for multiple myeloma. Blood Cancer J 2019;9:44. [Crossref] [PubMed]
- BeiGene. A Phase 1b/2 Study of BGB-11417in Monotherapy and in Various Combinations With Dexamethasone and Carfilzomib in Multiple Myeloma. ClinicalTrials.gov identifier: NCT04973605. Updated July 3, 2023. Accessed July 5, 2023. Available online: https://classic.clinicaltrials.gov/ct2/show/NCT04973605
- Sanofi. Isatuximab in Combination With Cemiplimab in Relapsed/Refractory Multiple Myeloma (RRMM) Patients. ClinicalTrials.gov identifier: NCT03194867. Updated April 19, 2023. Accessed July 5, 2023. Available online: https://classic.clinicaltrials.gov/ct2/show/NCT03194867
- Janssen Research & Development, LLC. A Study Comparing JNJ-68284528, a CAR-T Therapy Directed Against B-cell Maturation Antigen (BCMA), Versus Pomalidomide, Bortezomib and Dexamethasone (PVd) or Daratumumab, Pomalidomide and Dexamethasone (DPd) in Participants With Relapsed and Lenalidomide-Refractory Multiple Myeloma (CARTITUDE-4). ClinicalTrials.gov identifier: NCT04181827. Updated June 29, 2023. Accessed July 5, 2023. Available online: https://classic.clinicaltrials.gov/ct2/show/NCT04181827
- Casamassima F, Ruggiero C, Caramella D, et al. Hematopoietic bone marrow recovery after radiation therapy: MRI evaluation. Blood 1989;73:1677-81. [Crossref] [PubMed]
- Campbell BA, Hofman MS, Prince HM. A Novel Application of [18F]Fluorothymidine-PET ([18F]FLT-PET) in Clinical Practice to Quantify Regional Bone Marrow Function in a Patient With Treatment-Induced Cytopenias and to Guide "Marrow-Sparing" Radiotherapy Clin Nucl Med 2019;44:e624-6. [Crossref] [PubMed]
- Campbell BA, Callahan J, Bressel M, et al. Distribution Atlas of Proliferating Bone Marrow in Non-Small Cell Lung Cancer Patients Measured by FLT-PET/CT Imaging, With Potential Applicability in Radiation Therapy Planning. Int J Radiat Oncol Biol Phys 2015;92:1035-43. [Crossref] [PubMed]
- Elhammali A, Amini B, Ludmir EB, et al. New paradigm for radiation in multiple myeloma: lower yet effective dose to avoid radiation toxicity. Haematologica 2020;105:e355-7. [Crossref] [PubMed]
- Rades D, Hoskin PJ, Stalpers LJ, et al. Short-course radiotherapy is not optimal for spinal cord compression due to myeloma. Int J Radiat Oncol Biol Phys 2006;64:1452-7. [Crossref] [PubMed]
- Rudzianskiene M, Inciura A, Gerbutavicius R, et al. Single vs. multiple fraction regimens for palliative radiotherapy treatment of multiple myeloma : A prospective randomised study. Einzelne Fraktion vs. multiple Fraktionen in der palliativen Strahlentherapie des multiplen Myeloms : Eine prospektive randomisierte Studie. Strahlenther Onkol 2017;193:742-9. [Crossref] [PubMed]
- Meredith RF, Bassler JR, McDonald AM, et al. Biological Effective Radiation Dose for Multiple Myeloma Palliation. Adv Radiat Oncol 2023;8:101214. [Crossref] [PubMed]
- Ballas L. Phase II Multi-Institutional Study of Low-Dose (2Gy) Palliative Radiotherapy in the Treatment of Symptomatic Bone Metastases From Multiple Myeloma. ClinicalTrials.gov identifier: NCT03858205. Updated March 2, 2022. Accessed September 2, 2022. Available online: https://clinicaltrials.gov/ct2/show/NCT03858205
- Patchell RA, Tibbs PA, Regine WF, et al. Direct decompressive surgical resection in the treatment of spinal cord compression caused by metastatic cancer: a randomised trial. Lancet 2005;366:643-8. [Crossref] [PubMed]
- Gillespie EF, Mathis NJ, Marine C, Zhang Z, Barker CA, Guttmann DM, Kotecha R, McIntosh AF, Vaynrub M, Bartelstein M, Mitchell A. Prophylactic Radiation Therapy vs. Standard-of-Care for Patients with High-Risk, Asymptomatic Bone Metastases: A Multicenter, Randomized Phase II Trial. Int J Radiat Oncol Biol Phys 2022;114:1059. [Crossref]
- Sharma AM, Sackett M, Bueddefeld D, et al. Incidence of spinal disease and role of spinal radiotherapy in multiple myeloma. Curr Oncol 2018;25:e539-44. [Crossref] [PubMed]
- Elhammali A, Milgrom SA, Amini B, et al. Postoperative Radiotherapy for Multiple Myeloma of Long Bones: Should the Entire Rod Be Treated? Clin Lymphoma Myeloma Leuk 2019;19:e465-9. [Crossref] [PubMed]
- Rosen DB, Haseltine JM, Bartelstein M, et al. Should Postoperative Radiation for Long Bone Metastases Cover Part or All of the Orthopedic Hardware? Results of a Large Retrospective Analysis. Adv Radiat Oncol 2021;6:100756. [Crossref] [PubMed]
- Miyashiro D, Sanches JA. Mycosis fungoides and Sézary syndrome: clinical presentation, diagnosis, staging, and therapeutic management. Front Oncol 2023;13:1141108. [Crossref] [PubMed]
- Whittaker S, Hoppe R, Prince HM. How I treat mycosis fungoides and Sézary syndrome. Blood 2016;127:3142-53. [Crossref] [PubMed]
- Nguyen CV, Bohjanen KA. Skin-Directed Therapies in Cutaneous T-Cell Lymphoma. Dermatol Clin 2015;33:683-96. [Crossref] [PubMed]
- Wilcox RA. Cutaneous T-cell lymphoma: 2017 update on diagnosis, risk-stratification, and management. Am J Hematol 2017;92:1085-102. [Crossref] [PubMed]
- Patel AM, West L, Atluri PS, et al. Optimizing Palliative Focal Radiation Therapy Dose in Cutaneous T-Cell Lymphoma: How Low Can You Go? Pract Radiat Oncol 2023;13:e192-9. [Crossref] [PubMed]
- National Comprehensive Cancer Network. Primary Cutaneous Lymphomas (Version 1.2023). Accessed July 1, 2023. Available online: https://www.nccn.org/professionals/physician_gls/pdf/primary_cutaneous.pdf
- Carter J, Zug KA. Phototherapy for cutaneous T-cell lymphoma: online survey and literature review. J Am Acad Dermatol 2009;60:39-50. [Crossref] [PubMed]
- Phan K, Ramachandran V, Fassihi H, et al. Comparison of Narrowband UV-B With Psoralen-UV-A Phototherapy for Patients With Early-Stage Mycosis Fungoides: A Systematic Review and Meta-analysis. JAMA Dermatol 2019;155:335-41. [Crossref] [PubMed]
- Marka A, Carter JB. Phototherapy for Cutaneous T-Cell Lymphoma. Dermatol Clin 2020;38:127-35. [Crossref] [PubMed]
- Bleyer WA. Neurologic sequelae of methotrexate and ionizing radiation: a new classification. Cancer Treat Rep 1981;65:89-98. [PubMed]
- Kharfan Dabaja MA, Morgensztern D, Markoe AM, Bartlett-Pandite L. Radiation recall dermatitis induced by methotrexate in a patient with Hodgkin's disease. Am J Clin Oncol 2000;23:531-3. [Crossref] [PubMed]
- Bhangoo RS, Bhangoo MS, Mangold AR, et al. Radiation Recall Dermatitis After the Use of Pralatrexate for Peripheral T-cell Lymphoma. Adv Radiat Oncol 2018;4:31-4. [Crossref] [PubMed]
- Duvic M, Donato M, Dabaja B, et al. Total skin electron beam and non-myeloablative allogeneic hematopoietic stem-cell transplantation in advanced mycosis fungoides and Sezary syndrome. J Clin Oncol 2010;28:2365-72. [Crossref] [PubMed]
- Sehn LH, Gascoyne RD. Diffuse large B-cell lymphoma: optimizing outcome in the context of clinical and biologic heterogeneity. Blood 2015;125:22-32. [Crossref] [PubMed]
- Gisselbrecht C, Schmitz N, Mounier N, et al. Rituximab maintenance therapy after autologous stem-cell transplantation in patients with relapsed CD20(+) diffuse large B-cell lymphoma: final analysis of the collaborative trial in relapsed aggressive lymphoma. J Clin Oncol 2012;30:4462-9. [Crossref] [PubMed]
- Crump M, Neelapu SS, Farooq U, et al. Outcomes in refractory diffuse large B-cell lymphoma: results from the international SCHOLAR-1 study. Blood 2017;130:1800-8. Erratum in: Blood 2018;131:587-8. [Crossref] [PubMed]
- Van Den Neste E, Schmitz N, Mounier N, et al. Outcome of patients with relapsed diffuse large B-cell lymphoma who fail second-line salvage regimens in the International CORAL study. Bone Marrow Transplant 2016;51:51-7. [Crossref] [PubMed]
- Koukourakis MI, Giatromanolaki A, Schiza S, et al. Concurrent twice-a-week docetaxel and radiotherapy: a dose escalation trial with immunological toxicity evaluation. Int J Radiat Oncol Biol Phys 1999;43:107-14. [Crossref] [PubMed]
- Koukourakis MI, Koukouraki S, Giatromanolaki A, et al. Liposomal doxorubicin and conventionally fractionated radiotherapy in the treatment of locally advanced non-small-cell lung cancer and head and neck cancer. J Clin Oncol 1999;17:3512-21. [Crossref] [PubMed]
- Eisbruch A, Shewach DS, Bradford CR, et al. Radiation concurrent with gemcitabine for locally advanced head and neck cancer: a phase I trial and intracellular drug incorporation study. J Clin Oncol 2001;19:792-9. [Crossref] [PubMed]
- Graham MV, Jahanzeb M, Dresler CM, et al. Results of a trial with topotecan dose escalation and concurrent thoracic radiation therapy for locally advanced, inoperable nonsmall cell lung cancer. Int J Radiat Oncol Biol Phys 1996;36:1215-20. [Crossref] [PubMed]
- Verma V, Cushman TR, Tang C, et al. Toxicity of radiation and immunotherapy combinations. Adv Radiat Oncol 2018;3:506-11. [Crossref] [PubMed]
- Ho CI, Ballard HJ, Arscott WT, et al. Concurrent Use of Novel Agents and Radiation Is Tolerated in Lymphoma Patients. Blood 2019;134:2905. [Crossref]
- Ng AK, Yahalom J, Goda JS, et al. Role of Radiation Therapy in Patients With Relapsed/Refractory Diffuse Large B-Cell Lymphoma: Guidelines from the International Lymphoma Radiation Oncology Group. Int J Radiat Oncol Biol Phys 2018;100:652-69. [Crossref] [PubMed]
- Wright CM, Dreyfuss AD, Baron JA, et al. Radiation Therapy for Relapsed or Refractory Diffuse Large B-Cell Lymphoma: What Is the Right Regimen for Palliation? Adv Radiat Oncol 2022;7:101016. [Crossref] [PubMed]
- Figura NB, Sim AJ, Jain MD, et al. Radiation therapy prior to CAR T-cell therapy in lymphoma: impact on patient outcomes. Expert Rev Hematol 2022;15:1023-30. [Crossref] [PubMed]
- Hubbeling H, Silverman EA, Michaud L, et al. Bridging Radiation Rapidly and Effectively Cytoreduces High-Risk Relapsed/Refractory Aggressive B Cell Lymphomas Prior to Chimeric Antigen Receptor T Cell Therapy. Transplant Cell Ther 2023;29:259.e1-259.e10. [Crossref] [PubMed]
- Sim AJ, Figura NB, Dohm AE, et al. In-field failures in patients undergoing bridging radiotherapy for CD19-directed chimeric antigen receptor (CAR) T-cell therapy for recurrent/refractory large B-cell lymphomas. Int J Radiat Oncol Biol Phys 2021;111:S131. [Crossref]
- DeSelm C, Palomba ML, Yahalom J, et al. Low-Dose Radiation Conditioning Enables CAR T Cells to Mitigate Antigen Escape. Mol Ther 2018;26:2542-52. [Crossref] [PubMed]
- Pinnix CC, Gunther JR, Dabaja BS, et al. Bridging therapy prior to axicabtagene ciloleucel for relapsed/refractory large B-cell lymphoma. Blood Adv 2020;4:2871-83. [Crossref] [PubMed]
- Fang PQ, Gunther JR, Wu SY, et al. Radiation and CAR T-cell Therapy in Lymphoma: Future Frontiers and Potential Opportunities for Synergy. Front Oncol 2021;11:648655. [Crossref] [PubMed]
- Locke FL, Ghobadi A, Jacobson CA, et al. Long-term safety and activity of axicabtagene ciloleucel in refractory large B-cell lymphoma (ZUMA-1): a single-arm, multicentre, phase 1-2 trial. Lancet Oncol 2019;20:31-42. [Crossref] [PubMed]
- Schuster SJ, Bishop MR, Tam CS, et al. Tisagenlecleucel in Adult Relapsed or Refractory Diffuse Large B-Cell Lymphoma. N Engl J Med 2019;380:45-56. [Crossref] [PubMed]
- Abramson JS, Palomba ML, Gordon LI, et al. Lisocabtagene maraleucel for patients with relapsed or refractory large B-cell lymphomas (TRANSCEND NHL 001): a multicentre seamless design study. Lancet 2020;396:839-52. [Crossref] [PubMed]
- Terrones-Campos C, Ledergerber B, Vogelius IR, et al. Hematological toxicity in patients with solid malignant tumors treated with radiation - Temporal analysis, dose response and impact on survival. Radiother Oncol 2021;158:175-83. [Crossref] [PubMed]
- Goyal G. Testing the Combination of Nivolumab and ASTX727 for Relapsed or Refractory B-Cell Lymphoma. ClinicalTrials.gov identifier: NCT05272384. Updated June 22, 2023. Accessed July 1, 2023. Available online: https://clinicaltrials.gov/ct2/show/NCT05272384
- Novartis Pharmaceuticals. Tisagenlecleucel in Adult Patients With Aggressive B-cell Non-Hodgkin Lymphoma (BELINDA). ClinicalTrials.gov identifier: NCT03570892. Updated March 5, 2023. Accessed July 1, 2023. Available online: https://classic.clinicaltrials.gov/ct2/show/NCT03570892
- Novartis Pharmaceuticals. Study of Efficacy and Safety of CTL019 in Adult DLBCL Patients (JULIET). ClinicalTrials.gov identifier: NCT02445248. Updated May 11, 2023. Accessed July 1, 2023. Available online: https://classic.clinicaltrials.gov/ct2/show/NCT02445248
- Autolus Limited. CD19/22 CAR T Cells (AUTO3) for the Treatment of Diffuse Large B Cell Lymphoma (ALEXANDER). ClinicalTrials.gov identifier: NCT03287817. Updated October 20, 2022. Accessed July 1, 2023. Available online: https://classic.clinicaltrials.gov/ct2/show/NCT03287817
- Kite, A Gilead Company. Study of Effectiveness of Axicabtagene Ciloleucel Compared to Standard of Care Therapy in Patients With Relapsed/Refractory Diffuse Large B Cell Lymphoma (ZUMA-7). ClinicalTrials.gov identifier: NCT03391466. Updated June 22, 2023. Accessed July 1, 2023. Available online: https://clinicaltrials.gov/study/NCT03391466