Superior target delineation for stereotactic body radiotherapy of bone metastases from renal cell carcinoma on MRI compared to CT
Introduction
Ninety percent of all tumors originating from the kidney are renal cell carcinomas (RCC) (1). The incidence of RCC is on the rise partially due to the increased use of diagnostic imaging (2). Of all patients with RCC, 20–35% will develop bone metastases during the course of their disease (3). In metastatic RCC (mRCC) there has been a treatment shift towards targeted therapy which has resulted in a 50% increase in overall survival (4). Due to the increasing overall survival in mRCC, there is a need for better local control of the tumor and its metastases. When used for curative intentions, conventional radiotherapy techniques have proven to be unable to manage kidney motion resulting in large radiation fields including large volumes of healthy kidney and surrounding abdominal organs (5). As a result, lower radiation doses compared to other tumor sites were applied and conventional radiotherapy was thought to lead to suboptimal tumor control rates. RCC was thus assumed to be radioresistant, however this misunderstanding was due to the low dose given in historic series (5).
In recent literature, RCC has been shown sensitive to high dose radiotherapy applied with stereotactic body radiotherapy (SBRT) techniques (6-8). SBRT assures a substantial higher biological effective dose to the tumor compared to conventional fractionation schemes. In RCC, a larger dose per fraction, in both curative and palliative setting (6), can lead to local control rates up to 90% (7). Recommendations have been made for fractionation schemes for optimal local control in primary tumors: 24 Gy in 1 fraction, 32 Gy in 2 fractions, 36 Gy in 3 fractions or 35 Gy in 5 fractions in the primary setting (6).
The radiosensitivity of RCC for treatment of localized RCC might be translated towards mRCC. Image-guided SBRT provides good symptom palliation and local control (7,9). Treatment with SBRT for RCC induced an increase in local control rates from 50% to up to 85–100% (10-13). Currently the treatment planning of SBRT for mRCC is mainly CT-guided, including CT-based delineations (14-16). Furthermore in the delineation of spinal metastases, co-registration with MRI is recommended for the delineation of the spinal cord (16). However, SBRT is an emerging treatment option for non-spinal lesions, and in those treatment plans MRI is less frequently used (16). Recently, spine radiosurgery consortium consensus guidelines for target volume definition in spinal SBRT have been proposed. These recommendations included the use of MRI in target and organ at risk (OAR) delineation. The recommendations were assumed to be applicable to all types of spinal metastases, in which there was no special focus for bone metastases from RCC (17). However, we assume mRCC is a different subgroup within the bone metastases population, due to the different biological behavior of RCC tumor cells (6). In the non-metastatic setting of RCC there are recommendations to adjust the use of MRI in the diagnostic process, because the visibility of RCC in improved on MRI (18). We hypothesize that MRI also plays an important role in the visibility of mRCC in the context of stereotactic treatment planning. In this paper we will emphasize the importance of the use of MRI in stereotactic treatment planning for RCC bone metastases.
Methods
From June 2013 to August 2016, all consecutive patients who underwent SBRT for RCC bone metastases were included. All patients are participating in a bone metastases cohort and signed informed consent for their clinical data to be used for research purposes (https://clinicaltrials.gov/ct2/show/NCT02356497). Within this cohort, patients are treated with standard radiotherapy of single fraction external beam radiotherapy of 8 Gy. The dose distribution usually consist of a single field in posteroanterior direction with the 100% isodose line at 6 cm for a 6 MV photon beam and at 6 or 7 cm at a 10 MV photon beam.
Image acquisition and patient positioning
Prior to SBRT, patients CT and MRI images were acquired in radiotherapy position according to our clinical protocol. In plane resolution CT images were performed at 1 mm slice thickness on a large bore CT scanner (Philips Medical Systems, Best, the Netherlands). In addition, all patients underwent a 1.5 Tesla MRI scan (Achieva; Philips Medical System, Best, The Netherlands) with a 1.1–4 mm slice thickness. For every patient, T1-weighted images were acquired in transversal and sagittal direction, including a transversal multi echo DIXON scan, as well as T2-weighted images in transversal and sagittal direction, and diffusion weighted images (DWI). More details on MR sequences are listed in Table 1. During CT and MR imaging, patients were positioned in treatment position on a vacuum cushion (BlueBAG™, Elekta, Stockholm, Sweden), according to recommendation made by Dahele et al. (16).
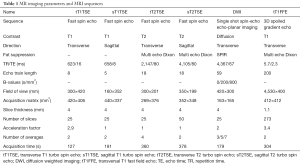
Full table
Target volume delineation
The transversal T1 TSE sequence was registered with the planning CT and all other MRI sequences were matched to this T1 sequence. Contouring was performed by a radiation oncologist specialized in bone metastases and verified by a radiologist on CT. Separately, the clinical contouring for MRI-based delineation was used, delineated by the responsible radiation oncologist, in adherence to local consensus contouring guidelines. Delineation was performed in an in house developed software system (19). The MR to CT registration was also executed in this software system according to clinical practice, using the mutual information registration algorithm within a rectangular box, containing the gross tumor volume (GTV).
In CT delineation, the focus was on delineation of tumor versus normal bone marrow and adjacent cortex. All scans were reviewed applying the same settings with window level at +300 Hounsfield units (HU) and window width at 1,000 HU. The standard setting of a window level at +40 HU and window width of 400 HU was also used. In MRI delineation, T1 images were used for target delineation aided by the information derived from the T2 and DWI sequences.
Data analysis
A comparison was made of the GTV as delineated on both CT and MRI, using the GTV volumes, the conformity index (CI) and distance between the centers of mass (dCOM). The CI is measured using the following formula: . A perfect agreement between the delineation on CT and MRI is indicated by a CI of 1. A CI of 0 means there is no overlap. The dCOM was defined as the dCOM, which implies that the centers of the two delineations are the same if this value was 0.
Statistical differences in volumes between CT and MR were tested with Wilcoxon signed-rank test, with a significance level of α =0.05. SPSS version 23.0 was used for the statistical analysis.
Results
Patient characteristics
Nine patients with 11 bone metastases from RCC, treated with SBRT were included (Table 2), consisting of eight patients with a single lesion, and one patient with three lesions. The median age was 65 years (range, 49–80 years). Most of the lesions were located in the thoracic or lumbar spine, and all lesions were osteolytic. All malignancies were pathologically proven RCC; 8 lesions (72.7%) had a clear cell subtype, and 3 lesions (27.3%) a chromophobe subtype.

Full table
Tumor volume on CT and MRI
The volumes of the lesions on MRI were larger in seven out of 11 cases compared to the CT, for the other four cases the volumes were comparable (Table 3). The mean size on MRI was 50.5 mL and the median 33.4 mL (range, 0.2–247.6 mL), compared to a mean and median volume of 35.6 and 18.1 mL respectively on CT (range, 0.1–195.9 mL). The difference in mean volume as delineated on CT and MRI was statistically significant (P=0.013). The CI in the different lesions varied between 0.09 and 0.82. The dCOM varied between 0.7 and 13.2 mm. A visual example of the difference in delineation in a representative case is shown in Figure 1.
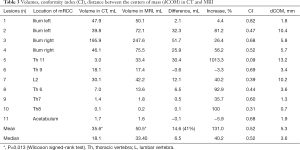
Full table
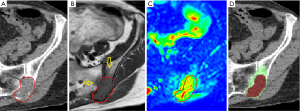
The seven lesions showing a larger volume on MRI do all have soft tissue involvement as well as bone marrow invasion. In all cases this was supported by the DWI sequence. In these lesions soft tissue involvement was not fully seen on the CT-scan. The median largest distance measured between the CT and MRI delineation was 26.7 mm (range, 15.1–31.3 mm).
In the four lesions showing no difference in volume on MRI compared to CT, there was no bone marrow, nor soft tissue invasion. These findings were supported by the DWI image.
Discussion
In this study, the use of CT and MRI for target volume delineation was prospectively compared in the stereotactic treatment of patients with bone metastases from RCC. The mean volume of the GTV on MRI was 41% larger (equaling almost 15 mL) compared to the mean volume of the GTV on CT, which was both clinically relevant and statistically significant. In seven out of 11 lesions the volumes of the lesions were larger on MRI comparing to the CT volume. This is probably due to superior visualization of soft tissue and bone marrow infiltration when using MR imaging compared with CT imaging (18). In 4 out of 11 lesions the volumes were comparable in size and, importantly, none of these lesions were smaller on MRI. Remarkably, three of those four lesions were chromophobe subtypes of RCC. However, it is more likely to conclude the lack of difference in those lesions is due to the absence of soft tissue or bone marrow infiltration, rather than a due to a different subtype of RCC.
The assumed radioresistance of RCC is questionable, as this was based on historic series prescribing radiation doses to primary RCC lesions which were too low to be effective (6). RCC may be more resistant than other tumors, but given high enough doses this may be overcome. However, results of this study suggest that part of the seemingly radioresistance of metastases from RCC might not be explained by inadequate dose regimen only, but also by suboptimal delineation due to CT-based contouring. It might be that part of the tumors received an underdosage if tumor delineation was based on CT information only. This would be the case especially in the peripheral parts of the bone lesions. In order to achieve optimal local control, an adequate dose on the entire GTV is relevant, which stresses the importance of accurate tumor delineation and an adequate biological effective dose. In this study, we have shown that the use of MRI results in a mean increase of the GTV volume, which supports the hypothesis that parts of the GTV would not have been contoured in a CT-only approach.
Although it seems that visualization of mRCC lesions on MRI is superior to CT, in this study we did not perform a pathological validation of the size of the lesions. To the best of our knowledge, there is no pathological validation of MRI imaging of RCC bone metastases performed up to date. This leaves the possibility that for example edema instead of macroscopic tumor extension contributes to the difference in tumor volumes. Another limitation is the small sample size of the present study, which might influence the result. To our knowledge, a comparison in delineation between CT and MRI in mRCC has not been performed to date. Next step is to perform a study with a larger sample size to test our preliminary conclusions.
There is no specific literature available on the added value of the use of MRI in the planning of planning in mRCC bone metastases. In diagnostic and functional imaging of RCC, MRI is more frequently used. MRI is described as a feasible alternative to CT for imaging RCC (18). Recent evidence also shows that MRI might be better to differentiate between renal lesions, which remained inconclusive at first on CT imaging, leading to further utility of renal MRI (20). Diffusion-weighted and perfusion-weighted imaging is also more often used for the evaluation to diagnose renal masses (21). Metastases of RCC can occur anywhere in the body, but are most frequently found in lung, bone, brain and liver (14). Imaging of these metastases is mostly done by CT-imaging, although in the detection of small cerebral and bone lesions, MRI is more sensitive and therefore the image modality of preference for those localizations (22).
In a critical review, Sahgal et al. reported on SBRT in spinal metastases originating from different primary tumors, describing that the imaging technique for treatment planning varies between institutions using CT only, or a fusion technique with MRI (23). Nguyen et al. use MRI for delineation of the GTV, and discovered in certain occasions that extension of the size of the lesion in oligometastatic disease was revealed on MRI scan during work-up to SBRT treatment (15), so this also confirmed the adjusted value of MRI. Sikka et al. reported on the added value of MRI in pancreatic metastases from RCC. These are described as typically T1 hypointense and T2 hyperintense, and the authors suggest that DWI can be useful in the diagnosis of RCC metastases (24). Metastases of RCC show restricted diffusion on DWI and can increase the visibility, especially in small lesions. Furthermore, DWI may be useful when it is not possible to administer for example gadolinium contrast in certain patients.
Our results also show the usability of DWI in delineation. All lesions, in which invasions in bone marrow or soft tissue were shown, the DWI supports the suggestion that there is extension which is seen on the T1 MRI images.
In the primary setting, it may be safer to irradiate in a hypofractionated setting, as is used in most clinical studies to primary treatment of localized RCC (12,13) and which is also advised by De Meerleer et al. (6). However, when more advanced image guided radiotherapy strategies show benefit of more precise targeting of the tumor, for example by MRI-guided linear accelerator radiotherapy with the MR-linac, it might be possible to increase the dose per fraction and treat patients with lesser fractions (25). Single fraction dose escalation up to 24 Gy in mRCC has been correlated with improved local control and progression free survival (14,26). In single fraction high dosages on spinal metastases the risk of vertebral compression fractures however increases (27). The cumulative incidence of compression fracture has been reported to be as high as 39% after single fraction spinal SBRT of 24 Gy. Considering the fact that the radioresistance of RCC is questionable, one could doubt whether it would be absolutely necessary to enhance this dosage to 24 Gy in a single fraction, instead of a slightly lower dose which is standard for bone metastases SBRT with different histology.
For mRCC, single fraction SBRT is associated with better local control than multiple hypofractionated fractions (28), although the OAR constraints are leading in the decision to opt for a single or multiple fraction SBRT approach in individual cases.
An alternative approach, assuring a high dose within the GTV, would be to deliver SBRT using a simultaneous integrated boost (SIB)-SBRT strategy. In SIB-SBRT treatment plans, the GTV is boosted while the dose to the surrounding clinical tumor volume (CTV) possibly containing microscopic tumor extension, is reduced. By limiting the dose to the relatively healthy surrounding bone, the risk of vertebral compression fractures might be decreased. In order to be able to boost the GTV, it is necessary to be certain about the true extent of the GTV. It is therefore of critical importance to use accurate and precise imaging techniques for GTV delineation.
Conclusions
Contouring of RCC bone metastases on MRI resulted in both clinically relevant and statistically significant larger volumes compared with contouring the same lesions with CT. It seems MRI represents the extent of the GTV in RCC bone metastases more accurately, possibly due to improved visualization of bone marrow infiltration and soft tissue expansion. Contouring based on CT-only could result in an underestimation of the actual tumor volume, which may cause an under dosage of the GTV in SBRT treatment plans, leading to lower local control rates.
Acknowledgements
None.
Footnote
Conflicts of Interest: Dr. Kerkmeijer reports a grant from AB Elekta to the UMC Utrecht (for MR-linac related research activities), outside of the submitted work.
Ethical Statement: This study was approved by the institutional board within the PRESENT cohort by the ethical committee of the University Medical Center Utrecht (number 13-261). The PRESENT cohort is published under NCT02356497 on ClinicalTrials.gov. All patients signed informed consent for their clinical data to be used for research purposes.
References
- Ljungberg B, Bensalah K, Bex A, et al. European Association of Urology Guidelines on Renal Cell Carcinoma 2015. Update 2015.
- Ferlay J, Steliarova-Foucher E, Lortet-Tieulent J, et al. Cancer incidence and mortality patterns in Europe: estimates for 40 countries in 2012. Eur J Cancer 2013;49:1374-403. [Crossref] [PubMed]
- Woodward E, Jagdev S, McParland L, et al. Skeletal complications and survival in renal cancer patients with bone metastases. Bone 2011;48:160-6. [Crossref] [PubMed]
- Soerensen AV, Donskov F, Hermann GG, et al. Improved overall survival after implementation of targeted therapy for patients with metastatic renal cell carcinoma: results from the Danish Renal Cancer Group (DARENCA) study-2. Eur J Cancer 2014;50:553-62. [Crossref] [PubMed]
- Ning S, Trisler K, Wessels BW, et al. Radiobiologic studies of radioimmunotherapy and external beam radiotherapy in vitro and in vivo in human renal cell carcinoma xenografts. Cancer 1997;80:2519-28. [Crossref] [PubMed]
- De Meerleer G, Khoo V, Escudier B, et al. Radiotherapy for renal-cell carcinoma. Lancet Oncol 2014;15:e170-7. [Crossref] [PubMed]
- Teh B, Bloch C, Galli-Guevara M, et al. The treatment of primary and metastatic renal cell carcinoma (RCC) with image-guided stereotactic body radiation therapy (SBRT). Biomed Imaging Interv J 2007;3:e6. [PubMed]
- Svedman C, Karlsson K, Rutkowska E, et al. Stereotactic body radiotherapy of primary and metastatic renal lesions for patients with only one functioning kidney. Acta Oncol 2008;47:1578-83. [Crossref] [PubMed]
- Tree AC, Khoo VS, Eeles RA, et al. Stereotactic body radiotherapy for oligometastases. Lancet Oncol 2013;14:e28-37. [Crossref] [PubMed]
- Staehler M, Bader M, Schlenker B, et al. Single fraction radiosurgery for the treatment of renal tumors. J Urol 2015;193:771-5. [Crossref] [PubMed]
- Ponsky L, Lo SS, Zhang Y, et al. Phase I dose-escalation study of stereotactic body radiotherapy (SBRT) for poor surgical candidates with localized renal cell carcinoma. Radiother Oncol 2015;117:183-7. [Crossref] [PubMed]
- Sun MR, Brook A, Powell MF, et al. Effect of Stereotactic Body Radiotherapy on the Growth Kinetics and Enhancement Pattern of Primary Renal Tumors. AJR Am J Roentgenol 2016;206:544-53. [Crossref] [PubMed]
- Chang JH, Cheung P, Erler D, et al. Stereotactic Ablative Body Radiotherapy for Primary Renal Cell Carcinoma in Non-surgical Candidates: Initial Clinical Experience. Clin Oncol (R Coll Radiol) 2016;28:e109-14. [Crossref] [PubMed]
- Zelefsky MJ, Greco C, Motzer R, et al. Tumor control outcomes after hypofractionated and single-dose stereotactic image-guided intensity-modulated radiotherapy for extracranial metastases from renal cell carcinoma. Int J Radiat Oncol Biol Phys 2012;82:1744-8. [Crossref] [PubMed]
- Nguyen QN, Shiu AS, Rhines LD, et al. Management of spinal metastases from renal cell carcinoma using stereotactic body radiotherapy. Int J Radiat Oncol Biol Phys 2010;76:1185-92. [Crossref] [PubMed]
- Dahele M, Zindler JD, Sanchez E, et al. Imaging for stereotactic spine radiotherapy: clinical considerations. Int J Radiat Oncol Biol Phys 2011;81:321-30. [Crossref] [PubMed]
- Cox BW, Spratt DE, Lovelock M, et al. International Spine Radiosurgery Consortium consensus guidelines for target volume definition in spinal stereotactic radiosurgery. Int J Radiat Oncol Biol Phys 2012;83:e597-605. [Crossref] [PubMed]
- Sankineni S, Brown A, Cieciera M, et al. Imaging of renal cell carcinoma. Urol Oncol 2016;34:147-55. [Crossref] [PubMed]
- Bol GH, Kotte AN, van der Heide UA, et al. Simultaneous multi-modality ROI delineation in clinical practice. Comput Methods Programs Biomed 2009;96:133-40. [Crossref] [PubMed]
- Willatt JM, Hussain HK, Chong S, et al. MR imaging in the characterization of small renal masses. Abdom Imaging 2014;39:761-9. [Crossref] [PubMed]
- Capitanio U, Montorsi F. Renal cancer. Lancet 2016;387:894-906. [Crossref] [PubMed]
- Mueller-Lisse UG, Mueller-Lisse UL. Imaging of advanced renal cell carcinoma. World J Urol 2010;28:253-61. [Crossref] [PubMed]
- Sahgal A, Larson DA, Chang EL. Stereotactic body radiosurgery for spinal metastases: a critical review. Int J Radiat Oncol Biol Phys 2008;71:652-65. [Crossref] [PubMed]
- Sikka A, Adam SZ, Wood C, et al. Magnetic resonance imaging of pancreatic metastases from renal cell carcinoma. Clin Imaging 2015;39:945-53. [Crossref] [PubMed]
- Raaymakers BW, Lagendijk JJ, Overweg J, et al. Integrating a 1.5 T MRI scanner with a 6 MV accelerator: proof of concept. Phys Med Biol 2009;54:N229-37.
- Yamada Y, Bilsky MH, Lovelock DM, et al. High-dose, single-fraction image-guided intensity-modulated radiotherapy for metastatic spinal lesions. Int J Radiat Oncol Biol Phys 2008;71:484-90. [Crossref] [PubMed]
- Sahgal A, Atenafu EG, Chao S, et al. Vertebral compression fracture after spine stereotactic body radiotherapy: a multi-institutional analysis with a focus on radiation dose and the spinal instability neoplastic score. J Clin Oncol 2013;31:3426-31. [Crossref] [PubMed]
- Ghia AJ, Chang EL, Bishop AJ, et al. Single-fraction versus multifraction spinal stereotactic radiosurgery for spinal metastases from renal cell carcinoma: secondary analysis of Phase I/II trials. J Neurosurg Spine 2016;24:829-36. [Crossref] [PubMed]