STAT RT: a prospective pilot clinical trial of Scan-Plan-QA-Treat stereotactic body radiation therapy for painful osseous metastases
Introduction
Most treatment regimens for the palliation of osseous metastases in the United States consist of 20–30 Gy delivered in 5–10 fractions (1). The 2014 American Society for Radiation Oncology Choosing Wisely guidelines recommend treatments with 8 Gy in 1 fraction, 20 Gy in 5 fractions, 24 Gy in 6 fractions, and 30 Gy in 10 fractions. Therefore, most current standard of care techniques extend over 1–3 weeks from time of treatment planning imaging to the end of treatment resulting in patient inconvenience and delayed palliation. In addition, palliative bone metastasis radiation treatments tend to utilize simple radiation therapy techniques such as opposed radiation beams which can irradiate significant amount of normal tissues with the full dose of radiation to the bone metastasis resulting in significant radiation-related toxicities (2). In place of opposed beams, highly conformal stereotactic body radiation therapy (SBRT) has been used to treat bone metastases with 1–5 fractions (14–40 Gy total dose) with local control rates exceeding 80% (3-10). SBRT has been shown in non-randomized studies to have longer durations of pain relief and shorter time to relief relative to traditional treatment modalities (2,10,11). Prior studies have shown that single 8 Gy treatments are as effective as multi-fraction regimens in relieving pain; however, re-treatment rates were higher among patients treated with single fraction, 20% vs. 8% respectively (12,13).
With a rising number of patients with painful bone metastases due to the aging population, there is a need for more effective palliative treatments that are more efficient to plan and deliver, require fewer total treatments, and minimize acute toxicities. Wong et al. attempted to streamline this process using a cone beam CT scan for planning purposes and single-fraction treatments delivered with simple one- or two-field techniques. This treatment strategy was effective in streamlining delivery (14); however, these treatments had poor conformality leading to high radiation doses to surrounding organs (15). In a recent publication, Mian et al. 2016 reported on a dosimetric analysis of a conformal Rapid Spine (RASp) planning method that meets RTOG 0631 objectives for spinal metastasis patients while limiting the treatment to 5 fields with a total of 15 segments to allow for rapid spine SBRT planning and delivery. These plans were compared to IMRT plans and were acceptable but not optimized as well as standard IMRT plans (16).
We have developed a highly coordinated, SBRT Scan-Plan-QA-Treat workflow in which CT simulation, treatment planning, pretreatment quality assurance (QA), and initial treatment delivery are all completed in a single day to maximize patient convenience and minimize time to treatment and pain relief.
This prospective phase II clinical trial was designed to evaluate the feasibility, safety, efficacy, and patient satisfaction of a multi-fraction Scan-Plan-QA-Treat workflow. Outcomes measured included pain, opioid use, response to treatment, adverse events (AEs), functional status, quality of life and patient satisfaction. We used the Brief Pain Inventory (BPI), Oral Morphine Equivalent Dose (OMED), pain response criteria as defined by the International Bone Metastases Consensus Working Party (IBMCWP), general and bone-pain specific quality of life scores, and a patient satisfaction survey to measure these outcomes (17-19).
Methods
Patient eligibility
Patients were prospectively enrolled on an IRB-approved pilot clinical trial and followed for one year. Patients were required to have biopsy-proven cancer with 1–3 painful osseous metastases, recent diagnostic imaging demonstrating metastatic disease, and persistent distinguishable pain at the target site(s) with an average pain score of 3 or greater. Patients could have other osseous metastases, but those sites had to have a pain score of 2 points less than target site(s). Target lesions could not have been previously irradiated. Long bone target lesions were required to have a Mirel’s fracture score of ≤7. Patients <18 years old or those with ≤12 weeks life expectancy as judged by the treating radiation oncologist were excluded. Patients with epidural compression of the spinal cord/cauda equina or greater than 25% spinal canal compromise were excluded.
Scan-Plan-QA-Treat planning and treatment
Patients were treated with radiation therapy according to the multi-fraction Scan-Plan-QA-Treat workflow, in which kV computed tomography (CT), inverse treatment planning, treatment plan QA via a phantom and a novel QA methodology called Monte Carlo Log QA (MCLogQA) (20), and delivery of first fraction are all performed in a single day. If available, previous diagnostic images were co-registered to the kVCT images to guide contouring of targets and organs at risk. Either IMRT or 3D planning was utilized with TomoHelical or TomoDirect beams (TomoTherapy®, Accuray Incorporated, Madison, WI). Accepted dose limits were used to protect nearby organs at risk (21,22).
All patients underwent non-contrast enhanced kVCT simulations with standard immobilization de-vices. Diagnostic contrast-enhanced CT scans, PET CT scans, or MRIs were co-registered to the kVCT simulation during treatment planning to define the gross tumor volume (GTV) and regional organs at risk. The GTV was expanded by 0–10 mm to create a PTV depending on critical adjacent structures such as the spinal cord which limited PTV expansion. Patients with PTVs that were within 5 mm of the spinal cord or cauda equina were excluded from the study since we felt that these patients should not be treated in a rapid investigational workflow which may not allow adequate time for treatment planning optimization. The minimal target coverage was 90% of the PTV receiving 95% of the prescribed dose.
Treatment courses consisted of 2–5 fractions delivered daily, with a dose of 5–10 Gy per fraction and a cumulative biological effective dose between 25 and 75 Gy. Prescribed dose and planning target volume were at the discretion of the treating radiation oncologist and were determined by tumor size, histology and proximity to critical structures.
QA: MCLogQA and Phantom-based QA comparison
Standard phantom-based QA was performed prior to the first fraction of every treatment, with treatments having to pass traditional 3 mm/3% gamma criteria for radiation oncology QA. In addition to the standard QA, the MCLogQA was also performed as part of the development of a more efficient QA process. The MCLogQA method is a two-step process comprised of a pre-treatment Monte Carlo secondary dose calculation and a post-treatment log file with exit detector data treatment delivery analysis (23-28).
Patient evaluation and assessment
Patients were assessed for pain, opioid usage, quality of life, performance status, and satisfaction. Data were collected prior to treatment and at 1 week, 4 weeks, 8 weeks, 12 weeks, 6 months and 12 months post-treatment. The BPI was used to measure patient’s subjective pain. Opioid usage was measured via patient report and converted into an OMED for the prior 72 hours at each time point. Patients’ functional status was assessed by the treating physician according to the Karnofsky Performance Scale.
Treatment response was determined using the worst pain score from the BPI and OMED according to guidelines set forth by the IBMCWP (17). Under the IBMCWP guidelines, complete response (CR) was defined as pain reduction to zero and OMED stable or reduced. Partial response (PR) was defined as pain reduction by two scores or more and OMED stable or reduced; or stable pain (SP) and OMED reduction by 25% or more. Pain progression (PP) was defined as pain increase by two scores or more and OMED stable or increased; or no change in pain and OMED increased by 25% or more. SP was defined as SP and stable OMED. We also classified patients with decreased pain but increased OMED as having SP, as this scenario was not described by the working group.
Functional Assessment of Cancer Therapy-Bone Pain (FACT-BP) and Functional Assessment of Cancer Therapy-General (FACT-G) surveys were used to assess changes in patient quality of life.
A study-specific survey was used to measure patient satisfaction. It consisted of 17 questions regarding patient perception of treatment and 3 questions related to patient satisfaction. A modified version of this satisfaction survey which assessed patient expectations about treatment was also given pre-treatment.
Development of acute radiation-related AEs was recorded at each follow-up visit and graded according to the Common Terminology Criteria for Adverse Events (CTCAE) v4.0 for the first 30 days after treatment. Late effects were also captured and graded according to CTCAE v4.0 after the first 30 days.
Statistical analysis
BPI measures of severity, worst pain, and interference with daily living were calculated and pre-treatment scores were compared with post-treatment values at each time point using Wilcoxon Signed Ranks tests. Karnofsky Performance Status, FACT-BP, and FACT-G scores were compared to pre-treatment levels at each time point using Wilcoxon Signed Ranks tests. OMED scores were compared to pre-treatment scores using paired two-sided t-tests. The P value was set to 0.05 for both Wilcoxon and t-tests for determining significance. Treatment response and satisfaction scores had no directly comparable pre-treatment values, so were reported descriptively without comparative statistical analysis.
Results
Patient and tumor characteristics
Table 1 presents a classification of the 28 patients accrued to the study. A mean of 1.3 metastases were treated per patient. At the time of analysis, surveys were available from 28, 16, 21, 13, 15, 13, and 9 patients at the pre-treatment, 1-, 4-, 8-, 12-, 26-, and 52-week time points, respectively. Median follow-up was 12 weeks as a result of loss to follow-up and deaths after treatment. Median overall survival was 9.6 months with a 6-month overall survival of 64%, and 12-month overall survival of 43%.
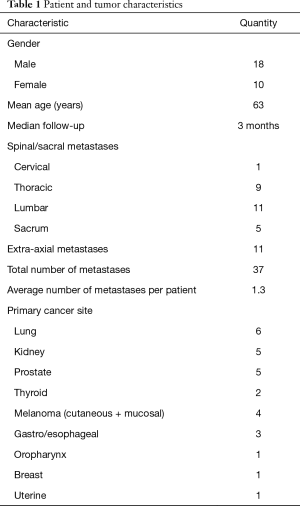
Full table
Scan-Plan-QA-Treat workflow feasibility
All patients except one were treated per the multi-fraction Scan-Plan-QA-Treat workflow, receiving their first treatment on the same day of CT simulation. The one exception was a patient who received his treatment the day after CT simulation because he needed additional analgesia and anxiolytics before he could tolerate treatment. For all patients treated per protocol, median time from CT to completion of the first treatment was 6.3 hours (range, 3.0–9.5 hours), and the median time from CT simulation to completion of the last treatment was 4.0 days (range, 1.0–9.0 days). All patients received their full radiation courses as prescribed with one exception. This exceptional case was prescribed 16 Gy in 2 fractions but only received 8 Gy in 1 fraction because the second fraction was delayed, during which time the patient had complete resolution of her pain and the second fraction was cancelled. Patients received a mean cumulative dose of 21.6 Gy in 3.1 fractions to the target lesions with ranges of 8–40 Gy in 1–5 fractions. The daily treatment times for all patients ranged from 2.5–30.1 minutes with an average of 11.3 minutes. The vast majority of patients (76%) were treated with two sub-fractions (for example if 8 Gy were delivered the patient received 2 sub-fractions of 4 Gy) including all patients treated to the spine at the level of the spinal cord (L1 vertebra and above). Daily CT IGRT was utilized prior to the first sub-fraction and the patient’s external marks were verified to be on the lasers before treating each sub-fraction.
AEs
There were no serious AEs were reported; all AEs were grade 2 or less. Radiation-related AEs included: fatigue (grade 2 in 2 patients and grade 1 in 2 patients), nausea (grade 2 in 1 patient and grade 1 in 2 patients), bone pain in the treated bone (grade1 in 4 patients), pneumonitis (grade 1 in 1 patient), rib fracture (grade 1 in 1 patient), numbness (grade1 in 1 patient), radiation dermatitis (grade 1 in 1 patient) and cough (grade 1 in 1 patient).
Pain
Pain at the target sites according to the BPI showed statistically significant improvements at all time points for worst pain, pain severity, and pain interference when compared to pre-treatment values with the exception that pain severity and interference scores were not significantly improved at the 1-year follow-up. Average pain scores are illustrated in Figure 1. Post-treatment average worst pain scores were significantly less than pre-treatment score at all time points including weeks 1, 4, 8, 12, 26, and 52 with P values of 0.001, 0.002, 0.001, 0.001, 0.003, and 0.042, respectively. Post-treatment average pain severity scores were significantly less than pre-treatment scores at all time points except at 52 weeks with P values of 0.001, 0.008, 0.001, 0.001, 0.002, and 0.086 for weeks 1, 4, 8, 12, 26, and 52, respectively. Post-treatment average pain interference scores were significantly less than pre-treatment scores at all time points except at 52 weeks with P values of 0.008, 0.002, 0.001, 0.005, 0.002, and 0.114 for weeks 1, 4, 8, 12, 26, and 52, respectively.
Based on the IBMCWP criteria, 69% of patients had at least a PR by one week after treatment. The 12-week time point had the highest percentage of pain response with 87% having at least a PR. By 12 months, 56% of patients surveyed still had at least a PR. Response to treatment is summarized in Figure 2.
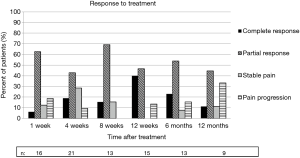
Opioid use, measured using OMED, was not found to be statistically different from baseline at any time post-treatment. This data was somewhat skewed by two patients using significantly higher opioid doses for the first three post-treatment time points due to sub-optimal medical analgesia at the time of enrollment. Changes in OMED were not found to be statistically significant regardless of inclusion or exclusion of outliers in the analysis. Figure 3 demonstrates average and median opioid use over time. Of note, no patients underwent vertebroplasty and only 1 of 28 patients (3.6%) underwent bisphosphonate therapy.
Functional status
Mean pre-treatment KPS score was 75. Mean KPS was increased at all time points after treatment with KPS scores of 83, 80, 87, 86, 84, and 80 at weeks 1, 4, 8, 12, 26, and 52, respectively; however, only the 12 week time point was found to be statistically significant (P=0.012).
Quality of life
Quality of life according to FACT-BP scores were significantly increased at all time points except at 52 weeks compared to baseline with P values of 0.001, 0.007, 0.001, 0.001, 0.001, and 0.221 at weeks 1, 4, 8, 12, 26, and 52 respectively. FACT-G scores were also increased at all time points after treatment, though only weeks 8 (P=0.023) and 26 (P=0.006) were found to have a statistically significant change from baseline. Figure 4 illustrates average FACT-BP and FACT-G scores over time.
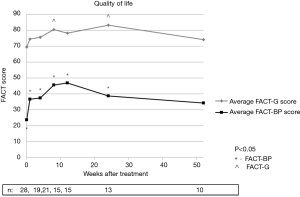
Retreatment
Two patients (7.1%) required re-treatment to one of their target lesions treated on protocol. Two patients (7.1%) had recurrences at the edge of the field of the target lesions and were treated with radiation to the areas of marginal recurrence.
Patient satisfaction
Seventy-four percent of patients reported feeling mostly or completely satisfied with the results of treatment at one week after treatment. At least 92% of patients reported being mostly or completely satisfied from week 8 until the end of follow-up at 1 year. There were two patients who reported feeling “not at all satisfied,” at any time point. Results from the satisfaction survey are shown in Figure 5.
All patients responded that they would or might recommend the treatment to others with a similar condition. For all time periods, there were 6 (7%) responses for “maybe” recommending treatment out of a total of 89 responses. All others responded that they would recommend treatment (93%). No patient said he or she would not recommend the treatment.
All patients except for one patient said they would or might choose this treatment again. There were 12 (13%) responses across all time periods which said they would not or might choose the treatment again out of 89 total responses. All other responses said they would choose the treatment again (87%).
QA methods
All patients’ treatment plans passed both the standard phantom QA and MCLog QA. The MCLogQA reconstructed dose was within 1% of the standard QA measured dose, well below the 5% threshold for passing QA (28).
Discussion
Our institution is the one of the first centers to our knowledge to use an expedited workflow to deliver SBRT to painful bone metastases in which CT simulation, planning, QA, and initial treatment are all performed in a single day to maximize patient convenience. The most similar workflow in the literature was published by Wong et al. and used a cone beam CT scan for planning purposes followed by a same-day single-fraction treatment delivered with simple one- or two-field techniques (14). Unlike the same-day workflow described by Wong et al., our same-day treatments are highly conformal and are not of any lower quality than our SBRT plans delivered with a conventional workflow.
Treatment with this patient centric workflow with the radiation oncology team was highly coordinated to provide same day planning and treatment proved to be feasible. Ninety-six percent of patients enrolled in the trial received their first treatment on the same day as their planning CT simulation, with a median time from CT simulation to 1st treatment of only 6.3 hours in those treated per protocol. Additionally, QA with the MCLogQA methodology was found to be clinically acceptable. Treatment with this workflow was safe, as no grade 3 or higher acute or late toxicities were observed.
While conventional radiotherapy has been reported to result in an improvement in pain in about 60% of patients (13,17), single-institution reports of the results of spine and non-spine SBRT have reported higher rates of pain improvement for osseous metastases (86–94%) (3,5,10). Treatment with the multi-fraction Scan-Plan-QA-Treat workflow was found to be as effective at relieving pain as other reports of SBRT for bone metastases, as 87% of the participants in this study had at least a PR at 12 weeks follow-up.
In addition to pain relief, these treatments demonstrated improvement in quality of life with respect to bone pain as well, but did not greatly impact measures of the patient’s general quality of life. The fact that opioid intake did not increase significantly over time is evidence that the reduction in pain noted was a result of the radiotherapy rather increased pain medications. Finally, our re-treatment rate of 7% is comparable to that of patients who receive more protracted conventional radiotherapy courses, and is lower than the 20% re-treatment rate seen with 8 Gy in 1 fraction with conventional radiotherapy (13). This is a small pilot study and the radiation fractionation schemas were heterogeneous and as such no dose response curve for retreatment can be determined. Our patients were treated with a mean dose of 21.6 Gy in 3.1 fractions, and our re-treatment rate of 7% (2/37 treated bone lesions) and pain response rate of 87% at 12 weeks are consistent with outcomes reported by other investigators for spinal SBRT (11,29) and with multi-fraction palliative care radiation regimens such as 30 Gy in 10 fractions.
High levels of patient satisfaction and treatment response were also achieved. The high patient satisfaction scores observed in this study likely reflect the patient’s appreciation of the convenience of same-day CT simulation, treatment planning, QA, and treatment delivery. Patient satisfaction may underestimate the improvement offered by this workflow because most patients enrolled had not experienced conventional, more protracted courses of radiotherapy for comparison. The Scan-Plan-QA-Treat workflow is a very patient-centric workflow for radiation oncology in that the entire radiation oncology healthcare team needs to be assembled and coordinated to participate in the patient’s care in a limited timeframe. In many ways, this treatment workflow mirrors requirements for current adaptive re-planning workflows used being clinically implemented for MR-LINACS (30). Team care coordination was one of the most difficult aspects of developing this patient-centric workflow and required a departmental e-mail alert of a STAT RT patient, scheduling of multiple healthcare providers efforts in series and coordination of CT simulation and TomoTherapy treatment unit schedules.
Limitations of this analysis include a small sample size and limited long term follow-up. Additionally, there is a potential for bias due to patients who died or were lost to follow-up and did not contribute data for the later time points. For example, the only 2 patients who were “not at all” satisfied with the results of the treatment were lost to follow-up after 4 weeks. However, this limitation is difficult to avoid in a study of patients with metastatic cancer with limited life expectancies.
Based on the results of the multi-fraction Scan-Plan-QA-Treat workflow, we have developed and completed accrual to a phase I/II single-fraction dose escalation trial with this same workflow and are currently analyzing the results of this study. In this way we will maximize patient convenience even more by utilizing single-fraction dose escalated radiation therapy to deliver 10–15 Gy per fraction. We further expedited the time from simulation to treatment by replacing phantom QA with Monte Carlo dose calculation and evaluation of multi-leaf collimator leaf opening times QA with the goal of completing CT simulation, planning, and treatment delivery in approximately 2–3 hours (31).
Conclusions
In conclusion the, results of this pilot study demonstrate that treating axial and extra-axial bone metastases with palliative SBRT via a multi-fraction Scan-Plan-QA-Treat workflow is feasible, safe, and results in a significant and durable improvement in pain and quality of life related to bone pain. Although performance status, general quality of life, and opioid use were not significantly altered, patient satisfaction was high with this same-day treatment workflow. With expedited workflows such as multi- and single-fraction Scan-Plan-QA-Treat, we hope to continue to develop treatments for patients with bone metastases that are highly effective, clinically efficient, and rapidly completed.
Acknowledgments
Funding: The project described was supported by Grant Number 1C1CMS331031 from the Department of Health and Human Services, Centers for Medicare & Medicaid Services.
Footnote
Conflicts of Interest: The authors have no conflicts of interest to declare.
Ethical Statement: Patients were prospectively enrolled on an IRB-approved pilot clinical trial and followed for one year.
References
- Fairchild A, Barnes E, Ghosh S, et al. International patterns of practice in palliative radiotherapy for painful bone metastases: Evidence-based practice? Int J Radiat Oncol Biol Phys 2009;75:1501-10. [Crossref] [PubMed]
- Gaze MN, Kelly CG, Kerr GR, et al. Pain relief and quality of life following radiotherapy for bone metastases: A randomised trial of two fractionation schedules. Radiother Oncol 1997;45:109-16. [Crossref] [PubMed]
- Gerszten PC, Burton SA, Ozhasoglu C, et al. Radiosurgery for spinal metastases: Clinical experience in 500 cases from a single institution. Spine (Phila Pa 1976) 2007;32:193-9. [Crossref] [PubMed]
- Tsai JT, Lin JW, Chiu WT, et al. Assessment of image-guided CyberKnife radiosurgery for metastatic spine tumors. J Neurooncol 2009;94:119-27. [Crossref] [PubMed]
- Nelson JW, Yoo DS, Sampson JH, et al. Stereotactic body radiotherapy for lesions of the spine and paraspinal regions. Int J Radiat Oncol Biol Phys 2009;73:1369-75. [Crossref] [PubMed]
- Gibbs IC, Kamnerdsupaphon P, Ryu MR, et al. Image-guided robotic radiosurgery for spinal metastases. Radiother Oncol 2007;82:185-90. [Crossref] [PubMed]
- Sheehan JP, Shaffrey CI, Schlesinger D, et al. Radiosurgery in the treatment of spinal metastases: Tumor control, survival, and quality of life after helical tomotherapy. Neurosurgery 2009;65:1052-61; discussion 1061-2. [Crossref] [PubMed]
- Bishop AJ, Tao R, Rebueno NC, et al. Outcomes for spine stereotactic body radiation therapy and an analysis of predictors of local recurrence. Int J Radiat Oncol Biol Phys 2015;92:1016-26. [Crossref] [PubMed]
- Owen D, Laack NN, Mayo CS, et al. Outcomes and toxicities of stereotactic body radiation therapy for non-spine bone oligometastases. Pract Radiat Oncol 2014;4:e143-9. [Crossref] [PubMed]
- Jhaveri PM, Teh BS, Paulino AC, et al. A dose-response relationship for time to bone pain resolution after stereotactic body radiotherapy (SBRT) for renal cell carcinoma (RCC) bony metastases. Acta Oncol 2012;51:584-8. [Crossref] [PubMed]
- Ryu S, Jin R, Jin JY, et al. Pain control by image-guided radiosurgery for solitary spinal metastasis. J Pain Symptom Manage 2008;35:292-8. [Crossref] [PubMed]
- Lutz S, Berk L, Chang E, et al. Palliative radiotherapy for bone metastases: An ASTRO evidence-based guideline. Int J Radiat Oncol Biol Phys 2011;79:965-76. [Crossref] [PubMed]
- Chow E, Zeng L, Salvo N, et al. Update on the systematic review of palliative radiotherapy trials for bone metastases. Clin Oncol (R Coll Radiol) 2012;24:112-24. [Crossref] [PubMed]
- Wong RK, Letourneau D, Varma A, et al. A one-step cone-beam CT-enabled planning-to-treatment model for palliative radiotherapy-from development to implementation. Int J Radiat Oncol Biol Phys 2012;84:834-40. [Crossref] [PubMed]
- Pope K, Fitzpatrick D, Potter A, et al. Dosimetric and clinical impact of 3D vs. 2D planning in palliative radiotherapy for bone metastases. Support Care Cancer 2013;21:2229-35. [Crossref] [PubMed]
- Mian OY, Thomas O, Lee JJ, et al. Timely stereotactic body radiotherapy (SBRT) for spine metastases using a rapidly deployable automated planning algorithm. Springerplus 2016;5:1337. [Crossref] [PubMed]
- Wu JS, Beaton D, Smith PM, et al. Patterns of pain and interference in patients with painful bone metastases: A brief pain inventory validation study. J Pain Symptom Manage 2010;39:230-40. [Crossref] [PubMed]
- Chow E, Wu JS, Hoskin P, et al. International consensus on palliative radiotherapy endpoints for future clinical trials in bone metastases. Radiother Oncol 2002;64:275-80. [Crossref] [PubMed]
- Broom R, Du H, Clemons M, et al. Switching breast cancer patients with progressive bone metastases to third-generation bisphosphonates: Measuring impact using the functional assessment of cancer therapy-bone pain. J Pain Symptom Manage 2009;38:244-57. [Crossref] [PubMed]
- Chen Q, Handsfield L, Wilson D, et al. SU‐D‐105‐06: A novel QA process based on monte carlo 2nd check and dose reconstruction from machine log file on TomoTherapy. Med Phys 2013.40.
- Timmerman R, Paulus R, Galvin J, et al. Stereotactic body radiation therapy for inoperable early stage lung cancer. JAMA 2010;303:1070-6. [Crossref] [PubMed]
- Schefter TE, Kavanagh BD, Timmerman RD, et al. A phase I trial of stereotactic body radiation therapy (SBRT) for liver metastases. Int J Radiat Oncol Biol Phys 2005;62:1371-8. [Crossref] [PubMed]
- Luo W, Li J, Price RA Jr, et al. Monte Carlo based IMRT dose verification using MLC log files and R/V outputs. Med Phys 2006;33:2557-64. [Crossref] [PubMed]
- Sterpin E, Salvat F, Olivera G, et al. Monte Carlo evaluation of the convolution/superposition algorithm of hi-art tomotherapy in heterogeneous phantoms and clinical cases. Med Phys 2009;36:1566-75. [Crossref] [PubMed]
- Sterpin E, Salvat F, Cravens R, et al. Monte Carlo simulation of helical tomotherapy with PENELOPE. Phys Med Biol 2008;53:2161-80. [Crossref] [PubMed]
- Chen Q, Lu W, Chen Y, et al. Validation of GPU based TomoTherapy dose calculation engine. Med Phys 2012;39:1877-86. [Crossref] [PubMed]
- Chen Q, Westerly D, Fang Z, et al. TomoTherapy MLC verification using exit detector data. Med Phys 2012;39:143-51. [Crossref] [PubMed]
- Handsfield LL, Jones R, Wilson DD, et al. Phantomless patient-specific TomoTherapy QA via delivery performance monitoring and a secondary Monte Carlo dose calculation. Med Phys 2014;41:101703. [Crossref] [PubMed]
- Gersten PC, Burton SA, Ozhasoglu C, et al. Radiosurgery for spinal metastases: clinical experience in 500 cases from a single institution. Spine 2007;32:193-9. [Crossref] [PubMed]
- Kontaxis C, Bol GH, Stemkens B, et al. Towards fast online intrafraction replanning for free-breathing stereotactic body radiation therapy with the MR-linac. Phys Med Biol 2017;62:7233-48. [Crossref] [PubMed]
- Wilson D, Sheng K, Yang W, et al. STAT RAD: A potential real-time radiation therapy workflow. In: Natanasabapathi G. editor. Modern Practices in Radiation Therapy. InTech, 2012.