Blueberry polyphenols play a preventive effect on alcoholic fatty liver disease C57BL/6 J mice by promoting autophagy to accelerate lipolysis to eliminate excessive TG accumulation in hepatocytes
Introduction
Fatty liver disease (FLD), caused by excessive accumulation of fat in hepatocytes, which is a frequent pathological change of liver, not an independent disease. In China, FLD is the seriously threatening hepatopathy that second to viral hepatitis with the increasing incidence, and the age of onset is getting younger and younger. It is divided into alcoholic fatty liver disease (AFLD) and nonalcoholic fatty liver disease (NAFLD) clinically, and both of them are significant contributors to the global financial burden of liver disorders that are consuming substantial healthcare resources and having a strong impact on mortality, morbidity, and life quality (1,2). Compared with NAFLD, AFLD has been focused on lesser studies, and biopsy-proven AFLD patients have been verified higher risk to developing hepatocellular carcinoma and liver-related mortality than biopsy-proven NAFLD in the few existing studies (1,3). Thus, we will select AFLD as the research object.
AFLD is an aftermath of chronic or excessive alcohol drinking. It is well-accepted that chronic alcohol intake can also bring about more severe forms of liver injury, such as hepatic steatosis, steatohepatitis, fibrosis, cirrhosis, hepatocarcinoma, and hepatic failure (4-6). Moreover, steatosis and inflammation, which lead to liver injury, are the significant symptoms of AFLD (7). By now, the pathogenesis of AFLD is still extremely limited. Recently, hepatocyte autophagy has been proved to play a protective effect in NAFLD and its complications by improving lipid accumulation and hepatic steatosis (8-10). Few pieces of literature reported that defective autophagy is also involved in the pathogenesis of AFLD, chronic ethanol-induced liver damage, and steatosis (11,12). Therefore, hepatocyte autophagy will be the focus of our study.
Blueberry polyphenol, extracted from blueberries, plays a potential preventive effect on liver injuries such as hepatic steatosis and NAFLD (13,14). Additionally, it functions as antioxidant, anti-inflammatory, and anti-obesity roles and took part in glucose and lipid metabolism (15-17). Anthocyanins, one of the main ingredients of blueberry polyphenols, are beneficial for ameliorating the metabolic syndrome issues, such as obesity, hyperglycemia, hyperinsulinemia, and hyperlipidemia (18). Nevertheless, the effects of blueberry polyphenol in AFLD are unclear, and if blueberry polyphenol accelerating cell autophagy is unprecedented. Thereby, the present work also aims to determine the impact of blueberry polyphenol on AFLD and its corresponding mechanism.
Methods
Extraction of blueberry polyphenols
Fresh Northeast China blueberries (Vacciniun spp.) were bought, and the extraction and purification of blueberry polyphenols were performed according to the previous report with some alterations (13,16,19). Briefly, the wild blueberries were lyophilized, then the crushed and homogenized dry blueberry pulp (15.0 g) was made by using a homogenizer (XHF-D; Ningbo Science & Biotechnology Co., Ningbo, Zhejiang, China) at 5,000 rpm for 1 min. Next, the homogenized sample was extracted with 200 mL ethanol solution (60%) for 120 min at 50 °C using the ultrasonic-assisted method. Subsequently, the crude blueberry extract was obtained after the extraction mixture was filtered by vacuum filtration with a filter paper (Hangzhou Special Paper Industry Co. Ltd., Zhejiang, China). Then, the purified blueberry extract was gained from the crude extract that underwent a purification with XAD-7 macroporous resin column (4.0 cm × 60 cm). Finally, the purified blueberry polyphenol extract (PPE) was freeze-dried into powder and stored in a −80 °C refrigerator until use.
Animals and groups
A total of 60 male C57BL/6J of 4-week-old mice were bought from the Laboratory Animal Center of Shanghai Public Health Clinical College. All mice were raised for at least one week in a temperature-controlled room at 25 °C and 60% relative humidity with 12 hours’ light cycle and given free access to standard laboratory diet and water before experiments. The mice were treated and cared for according to the ethical guidelines of the National Institute of Health Guide and Use of Laboratory Animals of China. In addition, they were divided into six groups, and each group included 10 mice: (I) low-fat diet alone, named as control group (LF, 10% of calories as fat (6% cocoa butter and 4% safflower oil)); (II) low-fat diet plus 100 mg/kg body weight (bw)/day PPE (control + PPE100); (III) low-fat diet plus 200 mg/kg bw/day PPE (control + PPE200); (IV) low-fat diet plus ethanol, named as model group (identical to the control LF diet but with ethanol added to account for 29% of total calories); (V) low-fat diet plus ethanol and 100 mg/kg body weight (bw)/day PPE (Model + PPE100); and (VI) low-fat diet plus ethanol and 200 mg/kg bw/day PPE (Model + PPE200). The PPE was dissolved in distilled water, while control and model groups were fed the same volume of normal saline. The work was performed for a continuous 1 month.
Sample harvest
Mice were anesthetized with Hypnorm, Dormicum, and water (1:1:2) at a dose of 0.15 mL/100 g bw by subcutaneous injection. Then, saline (0.9%) was infused into the heart for 15 min via the left ventricle, the heart was spontaneously beating on the initiation of infusion, and the animals were simultaneously allowed to exsanguinate via the right atrium puncture. The arterial blood from the left ventriclewas collected and centrifuged to gain theserum for total cholesterol (CHOL) and triglyceride (TG) detection. Subsequently, anterior fixation of tissue was conducted with 4% paraformaldehyde in phosphate buffer saline. Liver specimens obtained were after-fixed in 4% paraformaldehyde for 4 to 6 hours and placed in 20% sucrose solution in PBS overnight at 4 °C. Serial liver tissue sections were cut at 50 or 100 µm and reserved in the ultralow freezer at −80 °C for later use for the TG contents and configurations measurements of liver tissues by the Oil Red O (ORO) and hematoxylin and eosin (HE) dyeing. Moreover, the fresh liver tissues that untreated with 4% paraformaldehyde were stored at −80 °C for qRT-PCR and WB experiments.
Blood biochemical tests
Three hundred µL serum was taken out into Kuhlman AU480 blood biochemical analyzer to perform the CHOL and TG contents measurements by using kits (Hangzhou Yanchuang Bioengineering Institute, Zhejiang, China) according to manufacturer instructions.
ORO staining
ORO staining was performed for the determination of TG contents in liver tissues according to published literature (20). Briefly, tissue sections were fixed for 10 min with 10% ice paraformaldehyde and washed by distilled water three times after placed on slides for 30 min at room temperature. After slices underwent drying for several minutes, then slices were performed ORO staining with oil red and deionized water (3:2) for 8 min, 85% propylene glycol solution differentiation for 2 min, washed twice, hematoxylin counterstained for 30 s, flushed with water for 3 min. Lastly, an electronic microscope and the Olympus Image-Pro Plus 6.0 software were utilized for observation and quantitative analysis of ORO staining contents of TG.
The HE stains
According to the earlier paper (20), liver sections were executed HE stains for measurement of hepatic histology. The tissue sections were observed and photographed with ×100 and ×200 original magnification under an electronic microscope.
Real-time quantitative reverse transcription-polymerase chain reaction (qRT-PCR)
qRT-PCR was adopted to evaluate the mRNA expression level of lipogenesis-related genes such as sterol regulatory element-binding protein 1 (SREBP-1), fatty acid synthase (FAS), acetyl coenzyme A carboxylase (ACC) α (ACCα), and lipolysis-related genes such as adipose triglyceride lipase (ATGL) and sirtuin 1 (SIRT1) in mice liver tissues. Total RNA was obtained by using Trizol reagent (Invitrogen, USA) according to the manufacture’s instruction. Broadly, cDNA was produced by using cDNA chain synthesis kit (ThermoFisher) reagent, and DNA contamination was evaluated by RNAse-free DNase I. The determinations of high mRNA levels were performed by quantitative PCR (qPCR) instrument. GAPDH (PPM02946E) was used as an internal control to assess the relative quantification of detected genes by the 2−ΔΔCT method.
Western blot (WB)
WB was performed to detect the expression of autophagy proteins p62, LC3-I, and LC3-II. Proteins were extracted from mice liver tissues (250 mg) and then treated with RIPA lysis buffer (Beyotime, Jiangsu, China) holding a 2% cocktail pill (Roche). Samples were centrifuged at 12,000 g for 15 min at 4 °C, then the supernatant was collected, and the protein concentration was measured by BCA protein assay kit (Beyotime Institute). For detection of p62, LC3-I and LC3-II, the precipitated proteins (80 µg) were separated on 5% SDS-PAGE gel at 75 V for 2 h and transferred to PVDF membranes at 350 mA for 2 h. Then the membrane was blocked with 5% nonfat milk and diluted by TBST for 1h at room temperature. Subsequently, the membranes were incubated overnight with the primary antibody of p62, light chain 3 (LC3)-I and LC3-II (rabbit, Abcam, USA; 1:2,500) at 4 °C, and proteins were visualized by incubating in the secondary antibody (goat anti-rabbit IgG, ZSGB-BIO, China, 1:5,000) for 2 h, then scanned in Alpha Innotech (Bio-Rad) with ECL. Anti-β-actin was used as the internal control (mouse, Santa Cruz Biotechnology, USA; 1:5,000).
Statistical analysis
All data were analyzed by using One-way ANOVA, LSD-t, and S-N-K-q test by SPSS 16.0 software and expressed as the mean ± SD. Statistical significance was defined as P<0.05.
Results
Blueberry polyphenol reversed the alcohol-induced dyslipidemia
Blood biochemical tests showed that chronic alcohol intake could cause unusual blood fat, while blueberry polyphenol produced no effects on serum lipid levels. Whereas blueberry polyphenol could play therapeutic effects on alcohol-induced dyslipidemia and then presented a gradient effect (Figure 1). Concretely, compared with the control group, the serum contents of CHOL was significantly declined and TG was markedly elevatedin the model group (P<0.01), but the CHOL was slightly raised and TG was mildly slumped in both control + PPE100 and control + PPE200 groups with no statistical differences (P>0.05). Compared with the model group, the serum contents of CHOL were increased, and TG was reduced in both the model + PPE100 (P<0.05) and model + PPE200 groups (P<0.01). Besides, lower CHOL and higher TG were found in model + PPE100 than in control + PPE100 group, and in model + PPE200 than in control + PPE200 group (P<0.05).
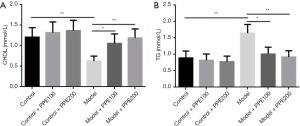
Blueberry polyphenol improved the alcohol-induced hepatic steatosis
ORO and HE staining revealed that chronic alcohol consumption led to hepatic steatosis with accumulated lipid droplets in the livers, whereas rare lipid droplets existed in livers in low-fat diet control mice or control mice treated with blueberry polyphenol. Meanwhile, the model mice administered with ethanol-fed showed no hepatic lipid droplets accumulation after accepted blueberry polyphenol managements, and blueberry polyphenol administration displayed a gradient effect (Figure 2A,B). Furthermore, the quantification of hepatic lipid TG contents showed that markedly higher liver TG levels in model group than in control group (P<0.01), and in model + PPE100 than in control + PPE100 group (P<0.05), as well as in model + PPE200 than in control + PPE200 group (P<0.05). Nevertheless, no statistical differences were showed between control and control + PPE100 or control and control+ PPE200 group (P>0.05). Notably, blueberry polyphenol administration observably decreased hepatic TG contents in model mice, and the declined TG levels were positive with the contents of blueberry polyphenol (Figure 2C).
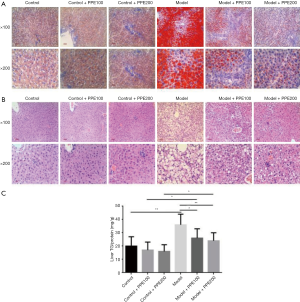
Blueberry polyphenol inhibited the effects of alcohol on lipogenic and lipoclastic genes
qRT-PCR attested that ethanol-fed remarkably raised the expression levels of lipogenic genes SREBP1, FAS, and ACCα, and markedly reduced the levels of the lipoclastic gene of ATGL and Sirt1 (P<0.01), whereas blueberry polyphenol alone did not transform the lipogenic and lipoclastic genes levels in control mice (P>0.05). Interestingly, compared with the model group, the lipogenic genes were decreased in model + PPE100 group (P<0.05) and significantly reduced in model + PPE200 group (P<0.01), and the lipoclastic genes were increased in model + PPE100 group (P<0.05) and significantly enhancive in model + PPE200 group (P<0.01) (Figure 3).
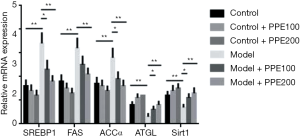
Blueberry polyphenol prevented alcohol-induced hepatocyte autophagy damage
WB identified that chronic drinking decreased or damaged the hepatocyte autophagy with higher level of p62 and lower specific value of LC3-II/LC3-I than in corresponding control mice (P<0.05 or P<0.01), however, compared with the control group, blueberry polyphenol added 100 or 200 mg/kg bw/day increased this autophagy with antipodal finds with model group (ethanol-fed added) with no statistical differences (P>0.05). Compared with the model group, blueberry polyphenol fortified the autophagy in hepatocyte with declined p62 level and elevated the specific value of LC3-II/LC3-I in model mice, and this stimulative effect of autophagy by blueberry polyphenol displayed a gradient tendency (Figure 4).
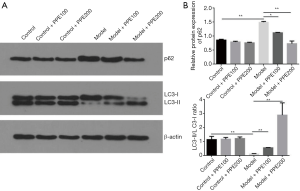
Discussion
In the current work, we initially demonstrated that the effects of blueberry polyphenols in AFLD and on hepatocyte autophagy: blueberry polyphenols alleviates AFLD in mice via promoting hepatocyte autophagy with declined p62 and increased LC3-II/LC3-I, then to decrease the serum lipid level with reductive TG and elevated CHOL contents in serum, as well as lower the hepatic steatosis conditions with lessened hepatic lipid droplets accumulation of TG, reduced lipogenic genes of SREBP1, FAS and ACCα, and accessorial lipodieretic genes of ATGL and Sirt1 in liver tissues.
As everyone knows, blueberry polyphenols have diversified potential health benefits. It can inhibit endogenous and exogenous oxidative productions of nitric oxide (NO) and intracellular reactive radical species (ROS) to act as antioxidants then to regulate homeostasis, wound healing, inflammation and mitochondrial respiration, prevent cell damage and the occurrence and development of cancers (15,21,22). Blueberry polyphenols have been reported anti-inflammatory through reducing the inflammatory markers of cyclooxygenase-2 (Cox-2), inducible nitric oxide synthase (iNOS), and interleukin-1b (IL-1β) (15,23,24). Blueberry polyphenols have also been proved to take part in glucolipid metabolism. It can down-regulate PEPCK mRNA (a key-enzyme of the citric acid cycle (TCA), involved in hepatic energy metabolism and gluconeogenesis) in rat hepatoma cells (H4IIE), and the inhibition of PEPCK mRNA will lead to repressing gluconeogenesis and consequent blood glucose decline to play antidiabetic activity, and inhibit anabolic reactions to functions as tumor-suppressor (15,25-27). Besides, excessive lipid accumulation could cause lipid peroxidation to induce the cells’ injury (17). Steatosis and inflammation, which lead to liver injury, are the significant symptoms of AFLD (7). Thus, these reported conclusions were forcefully supported our finding that blueberry polyphenols improved the steatosis in the liver tissue induced by chronic alcohol intake. In Jiao et al.’s study, blueberry polyphenols were attested to decrease the low-density lipoprotein (LDL-C) and increased high-density lipoprotein cholesterol (HDL-C) level in serum, significantly reduced the TCHO levels in liver in high-fat diet(HFD)-induced obese C57BL/6 J mice. Complementary, blueberry polyphenols also decreased the serum TG, alanine aminotransferase (ALT), aspartate transaminase (AST), and leptin levels, but not significantly (P>0.05), and not reduced the serum T-CHO levels. Blueberry polyphenols did not reduce the TG levels in the liver in HFD-induced obese mice (16). In our study, we found that blueberry polyphenols alone did not impact the serum TCHO and TG levels, as well as TG content in the liver compared with the normal mice, but increased the serum TCHO and decreased TG levels both in serum and liver in AFLD mice. Despite some contradictions existed, the model is different, and the effect of attenuating the abnormally elevated lipid metabolism is consistent with Jiao et al.’s report. Additionally, in our unreported paper, although serum CHOL was abnormally elevated in 42.86% NAFLD patients, there were 5.49% of patients showed abnormally descending serum CHOL. So, blueberry polyphenols increased the serum TCHO in AFLD mice also could prove its effects of lowering fat. Besides, rare evidence supported that blueberry polyphenols can play potential protective roles on liver injuries such as hepatic steatosis and NAFLD (13,14), and it can ameliorate microvascular steatosis in hepatocytes and reduce adipose cell sizes via reducing the levels of the lipogenic gene of PPARγ, FAS, and SREBP-1 and significantly increasing the levels of the lipoclastic gene of CPT 1 and PPARα in liver tissue in HFD-induced obese C57BL/6J mice (16). However, the effects of blueberry polyphenols in AFLD are unreported. Here, we first identified that blueberry polyphenols were beneficial to AFLD that improved the chronic ethanol intake-induced hepatic steatosis, elevated blood lipids and impaired hepatocyte autophagy in male C57BL/6J mice, and these preventive effects of blueberry polyphenols in AFLD appeared with a concentration gradient effect. In our study, we found that blueberry polyphenols did not produce effects on the lipogenic or lipoclastic genes levels in normal mice, and attenuated ethanol intake-induced hepatic steatosis with decreased TG content, reduced lipogenic genes levels of SREBP1, FAS, and ACCα, and raised lipoclastic genes levels of ATGL and Sirt1in liver in AFLD mice that supported by the above reports.
Although the pathogenesis of AFLD is still very limited, a small number of reports demonstrated defective hepatocyte autophagy is a crucial pathogenesis of both NAFLD and AFLD, even the complications of them, that lead to lipid accumulation and hepatic steatosis, while up-regulation of autophagy can accelerate the fat elimination in liver then to prevent hepatocytes injuries such as steatosis and cell death (8-12). Here, we also proved that chronic alcohol consumption induced hepatic steatosis with accumulated lipid droplets and increased TG content in the livers compared with low-fat diet control mice, and these alterations induced by ethanol is associated with down-regulated autophagy (enhancive protein expression level of p62, and decreased protein ratio of LC3-II/LC3-I), increased lipogenic mRNA levels of SREBP1, FAS, and ACCα, and declined lipodieretic mRNAs of ATGL and Sirt1. As we all know, LC3-I and LC3-II are only and disparate forms of LC3 protein (a biomarker protein on the autophagic membrane) in cell, when the LC3-I converts into LC3-II via proteolytic cleavage and lipidation or LC3-II/LC3-I is increased and the total level of LC3 does not increase, that can represent a normal autophagy function in mammalian and also can reflect the autophagy level in cells (9,28,29). Moreover, the accumulation or increase of p62 in vivo is a hallmark of impaired autophagy (30,31). However, the roles of blueberry polyphenols on cell autophagy are unclear by now, and we firstly found that blueberry polyphenols promote cell autophagy in low-fat/normal diet control mice, but there was no statistical difference noted. However, blueberry polyphenols 100 or 200 mg/kg bw/day feeding for a continuous 1 month can markedly promote cell autophagy with decreased p62 and increased LC3-II/LC3-I in liver compared with the low-fat diet plus ethanol model mice, and the effect is more distinct while feeding with 200 mg/kg bw/day.
Cell autophagy but not lipid droplets is a compensatory mechanism to promote energy metabolism, and cell autophagy can accelerate the fat elimination (9,32). ATGL is a lipoclastic enzyme by catalyzing the initiating step of lipolysis via hydrolyzing TGs in cytosolic lipid droplets, and scarce ATGL results in the accumulation of TGs and LC3-II, decreased p62 expression in most organs and cells, such as macrophages, that indicates down-regulation of ATGL will bring about the increased autophagy (32). Besides, MnCl2-induced autophagic dysregulation with decreased LC3-II/LC3-I level causes an increase of SIRT1level, and this suggests that autophagy is associated with SIRT1 (33). Autophagy is known to be a pivotal mechanism in keeping the balance of liver lipid metabolism. In the study of Zhang et al., a spontaneous hepatic steatosis, presented as massive accumulation of lipid droplets with significant accessorial TG in hepatocytes, which was accompanied by upregulated lipid synthesis-associated proteins (primarily triglyceride synthesis) levels of ACCα, FAS and SREBP1 that might related to the autophagy dysfunction with upregulated P62 and reduced LC3BII/LC3BI ratio both in vitro (34), these findings are consistent with our outcomes. Hence, blueberry polyphenols resist AFLD may through facilitating hepatocytes autophagy to promote lipolysis and remove excessive TG accumulation in liver cells.
Conclusions
In the present work, we can obtain several conclusions as follows:
- Blueberry polyphenols can alleviate alcohol-induced fatty liver disease with a concentration gradient effect;
- Blueberry polyphenols can inhibit the expression of lipid synthesis-associated genes caused by alcohol;
- Blueberry polyphenols can facilitate the expression of lipid disintegration-associated genes induced by alcohol;
- The mechanism of blueberry polyphenols improving the hepatic steatosis in AFLD C57BL/6J mice may be associated with enhanced cell autophagy to accelerate the decomposition of fat than to eliminate excessive TG accumulation in hepatocytes.
Acknowledgments
Funding: The present study was supported by a grant from the Key Project of Natural Science Foundation of Zhejiang Province (No. LZ16H160003).
Footnote
Conflicts of Interest: All authors have completed the ICMJE uniform disclosure form (available at http://dx.doi.org/10.21037/apm.2020.03.38). The authors have no conflicts of interest to declare.
Ethical Statement: The authors are accountable for all aspects of the work in ensuring that questions related to the accuracy or integrity of any part of the work are appropriately investigated and resolved.The mice were treated and cared for according to the ethical guidelines of the National Institute of Health Guide and Use of Laboratory Animals of China.
Open Access Statement: This is an Open Access article distributed in accordance with the Creative Commons Attribution-NonCommercial-NoDerivs 4.0 International License (CC BY-NC-ND 4.0), which permits the non-commercial replication and distribution of the article with the strict proviso that no changes or edits are made and the original work is properly cited (including links to both the formal publication through the relevant DOI and the license). See: https://creativecommons.org/licenses/by-nc-nd/4.0/.
References
- Younossi Z, Henry L. Contribution of Alcoholic and Nonalcoholic Fatty Liver Disease to the Burden of Liver-Related Morbidity and Mortality. Gastroenterology 2016;150:1778-85. [Crossref] [PubMed]
- Valencia-Rodríguez A, Vera-Barajas A, Barranco-Fragoso B, et al. New insights into the association between non-alcoholic fatty liver disease and atherosclerosis. Ann Transl Med 2019;7:S300. [Crossref] [PubMed]
- Haflidadottir S, Jonasson JG, Norland H, et al. Long-term follow-up and liver-related death rate in patients with non-alcoholic and alcoholic related fatty liver disease. BMC Gastroenterol 2014;14:166. [Crossref] [PubMed]
- Gao B, Bataller R. Alcoholic liver disease: pathogenesis and new therapeutic targets. Gastroenterology 2011;141:1572-85. [Crossref] [PubMed]
- Lucey MR, Mathurin P, Morgan TR. Alcoholic hepatitis. N Engl J Med 2009;360:2758-69. [Crossref] [PubMed]
- Lakshman MR. Some novel insights into the pathogenesis of alcoholic steatosis. Alcohol 2004;34:45-8. [Crossref] [PubMed]
- Zhong F, Hu Z, Jiang K, et al. Complement C3 activation regulates the production of tRNA-derived fragments Gly-tRFs and promotes alcohol-induced liver injury and steatosis. Cell Res 2019;29:548-61. [Crossref] [PubMed]
- Wu WKK, Zhang L, Chan MTV. Autophagy, NAFLD and NAFLD-Related HCC. Adv Exp Med Biol 2018;1061:127-38. [Crossref] [PubMed]
- Yan R, Niu CY, Tian Y. Roles of Autophagy and Protein Kinase C-epsilon in Lipid Metabolism of Nonalcoholic Fatty Liver Cell Models. Arch Med Res 2018;49:381-90. [Crossref] [PubMed]
- Wang Y, Zhao H, Li X, et al. Tangshen Formula Alleviates Hepatic Steatosis by Inducing Autophagy Through the AMPK/SIRT1 Pathway. Front Physiol 2019;10:494. [Crossref] [PubMed]
- Chao X, Wang S, Zhao K, et al. Impaired TFEB-Mediated Lysosome Biogenesis and Autophagy Promote Chronic Ethanol-Induced Liver Injury and Steatosis in Mice. Gastroenterology 2018;155:865-79.e12. [Crossref] [PubMed]
- Tang L, Yang F, Fang Z, et al. Resveratrol Ameliorates Alcoholic Fatty Liver by Inducing Autophagy. Am J Chin Med 2016;44:1207-20. [Crossref] [PubMed]
- Liu Y, Wang D, Zhang D, et al. Inhibitory effect of blueberry polyphenolic compounds on oleic acid-induced hepatic steatosis in vitro. J Agric Food Chem 2011;59:12254-63. [Crossref] [PubMed]
- Håkansson A, Bränning C, Molin G, et al. Blueberry husks and probiotics attenuate colorectal inflammation and oncogenesis, and liver injuries in rats exposed to cycling DSS-treatment. PLoS One 2012;7:e33510. [Crossref] [PubMed]
- Hoskin RT, Xiong J, Esposito DA, et al. Blueberry polyphenol-protein food ingredients: The impact of spray drying on the in vitro antioxidant activity, anti-inflammatory markers, glucose metabolism and fibroblast migration. Food Chem 2019;280:187-94. [Crossref] [PubMed]
- Jiao X, Wang Y, Lin Y, et al. Blueberry polyphenols extract as a potential prebiotic with anti-obesity effects on C57BL/6 J mice by modulating the gut microbiota. J Nutr Biochem 2019;64:88-100. [Crossref] [PubMed]
- Liu Y, Liu M, Chen Q, et al. Blueberry Polyphenols Ameliorate Visible Light and Lipid-Induced Injury of Retinal Pigment Epithelial Cells. J Agric Food Chem 2018;66:12730-40. [Crossref] [PubMed]
- Jayaprakasam B, Olson LK, Schutzki RE, et al. Amelioration of obesity and glucose intolerance in high-fat-fed C57BL/6 mice by anthocyanins and ursolic acid in Cornelian cherry (Cornus mas). J Agric Food Chem 2006;54:243-8. [Crossref] [PubMed]
- Liu Y, Zhang D, Hu J, et al. Visible Light-Induced Lipid Peroxidation of Unsaturated Fatty Acids in the Retina and the Inhibitory Effects of Blueberry Polyphenols. J Agric Food Chem 2015;63:9295-305. [Crossref] [PubMed]
- Zhang YH, Ma Q, Ding P, et al. S100A4 Gene is Crucial for Methionine-Choline-Deficient Diet-Induced Non-Alcoholic Fatty Liver Disease in Mice. Yonsei Med J 2018;59:1064-71. [Crossref] [PubMed]
- Lo Faro ML, Fox B, Whatmore JL, et al. Hydrogen sulfide and nitric oxide interactions in inflammation. Nitric Oxide 2014;41:38-47. [Crossref] [PubMed]
- Prasad S, Gupta S, Tyagi A. Reactive oxygen species (ROS) and cancer: Role of antioxidative nutraceuticals. Cancer Letters 2017;387:95-105. [Crossref] [PubMed]
- Correia R, Grace MH, Esposito D, et al. Wild blueberry polyphenol-protein food ingredients produced by three drying methods: Comparative physico-chemical properties, phytochemical content, and stability during storage. Food Chemistry 2017;235:76-85. [Crossref] [PubMed]
- Lee YM, Yoon Y, Yoon H, et al. Dietary anthocyanins against obesity and inflammation. Nutrients 2017. [Crossref] [PubMed]
- Welch C, Zhen J, Bassène E, et al. Bioactive polyphenols in kinkéliba tea (Combretum micranthum) and their glucose-lowering activities. Journal of Food and Drug Analysis 2018;26:487-96. [Crossref] [PubMed]
- Yan F, Dai G, Zheng X. Mulberry anthocyanin extract ameliorates insulin resistance by regulating PI3K/AKT pathway in HepG2 cells and db/db mice. J Nutr Biochem 2016;36:68-80. [Crossref] [PubMed]
- Montal ED, Dewi R, Bhalla K, et al. PEPCK coordinates the regulation of central carbon metabolism to promote cancer cell growth. Molecular Cell 2015;60:571-83. [Crossref] [PubMed]
- Kadowaki M, Karim MR. Cytosolic LC3 ratio as a quantitative index of macroautophagy. Methods Enzymol 2009;452:199-213. [Crossref] [PubMed]
- Oh SH, Choi YB, Kim JH, et al. Quantification of autophagy flux using LC3 ELISA. Anal Biochem 2017;530:57-67. [Crossref] [PubMed]
- Bjørkøy G, Lamark T, Brech A, et al. p62/SQSTM1 forms protein aggregates degraded by autophagy and has a protective effect on huntingtin-induced cell death. J Cell Biol 2005;171:603-14. [Crossref] [PubMed]
- Nakai A, Yamaguchi O, Takeda T, et al. The role of autophagy in cardiomyocytes in the basal state and in response to hemodynamic stress. Nat Med 2007;13:619-24. [Crossref] [PubMed]
- Goeritzer M, Vujic N, Schlager S, et al. Active autophagy but not lipophagy in macrophages with defective lipolysis. Biochim Biophys Acta 2015;1851:1304-16. [Crossref] [PubMed]
- Ma J, Zhang Y, Ji H, et al. Overexpression of miR-138-5p suppresses MnCl2 -induced autophagy by targeting SIRT1 in SH-SY5Y cells. Environ Toxicol 2019;34:539-47. [Crossref] [PubMed]
- Zhang X, Zhang P, Gao J, et al. Autophagy dysregulation caused by ApoM deficiency plays an important role in liver lipid metabolic disorder. Biochem Biophys Res Commun 2018;495:2643-8. [Crossref] [PubMed]