Gandou decoction, a Chinese medicinal formula, in the treatment of hepatic injury by Wnt/β-catenin pathway regulation in models of Wilson disease
Introduction
Wilson disease (WD), also known as hepatolenticular degeneration (HLD), is an autosomal recessive inherited copper metabolism disorder, which is caused by mutations of the copper-transporting P-type ATPase (ATP7B) gene located on chromosome 13q14.3. It is characterized by excess copper accumulation in tissues and organs like the liver, brain, and cornea. It leads to hepatic damage, neuropsychiatric syndromes, corneal K-F ring, and other symptoms and signs. WD commonly occurs during adolescence, and its prevalence is estimated to be 1 case per 30,000 in populations of European descendants (1), and 5.87 cases per 100,000 in East Asian populations (2). Hepatic injury is one of the most common symptoms of WD patients. ATP7B, the pathogenic gene, is mainly expressed in the liver, and this is likely the reason why the liver is the premier and the uppermost target injury organ of WD (3). The hepatic injury occurs across the entire disease course beginning from birth, and, as the leading WD-related cause of death, has become a focal issue in the prevention and treatment of WD. Therefore, the remedy for hepatic injury is one of the most crucial aspects of WD treatment.
The metal-chelating agent, D-penicillamine (PCA), has been the preferred medication for WD therapy for decades, but its adverse reactions are too severe to be tolerated for patients. They can cause irreversible deterioration of neurology, drug allergy, and hematologic complications (4). Consequently, the development of drugs with less adverse reactions and diversified effect targets has become a critical breakthrough point for treating WD, especially with liver injury.
Traditional Chinese medicine has been widely used to treat WD, and its high therapeutic effects and low adverse reaction rate have been demonstrated (5). Gandou decoction (GDD) is a Chinese herb formula directed by “heat elimination, detoxification, diuresis, and viscus regulation”, and has proven to be effective in treating WD patients, with its effective rate being 84.1% (6). Additionally, studies that showed a regulative effect on programmed cell death (PCD) after GDD administration demonstrated that GDD protected hepatocytes via varieties of pathways, including Wnt/β-catenin, extracellular signal-regulated kinase (ERK). and mitogen-activated protein kinase (MAPK). However, the exact mechanism of GDD is still unclear.
Wnt/β-catenin signaling pathway affects physiological processes such as liver growth, development, metabolism, and participates in the pathological processes of liver diseases, including liver fibrosis and liver cancer (7). Stress and cell damage such as DNA damage response (DDR) are closely related to this classic signal pathway (8). Our previous studies had found that high a concentration of copper could induce oxidative stress and inactivation of the Wnt/β-catenin signaling pathway in hepatocytes, thereby inducing hepatocyte damage. In order to further clarify the efficacy mechanism of GDD in the treatment of WD liver injury, we hypothesized that the pathophysiological process of WD liver injury is related to Wnt/β-catenin pathway and that GDD treats liver injury by activating Wnt/β-catenin signal pathway. Therefore, to confirm this hypothesis, this study aimed to investigate the effect of GDD on the WD model. We present the following article in accordance with the ARRIVE reporting checklist (available at http://dx.doi.org/10.21037/apm-20-301).
Methods
GDD preparation
Components of GDD are listed in Table 1. The standardized GDD extract was purchased from Tongrentang Chinese Medicine (Hefei, China) and met the standards set by the Chinese Pharmacopoeia (2015 Edition). All the components were extracted with 400 mL boiling water for 2 h, and the yield was 37.5%. Standardization was conducted using high-performance liquid chromatography as in the previous study (9).
The composition of GDD of previous report of preparation and standardization performed by high performance liquid chromatography (HPLC).
To simulate the metabolic process of traditional Chinese formula for following in vitro experiments, we acquired the serum of GDD from mice after 15 days of GDD intragastric administration. Whole blood sera were collected 6 h after the last administration from the abdominal aorta.
Reagents and materials
PCA was purchased from Shangyao Xinyi Pharmaceutical Co., Ltd. (Shanghai, China). Xav-939, a Wnt/β-catenin inhibitor, was purchased from Selleck Chemicals (TX, USA). Roswell Park Memorial Institute (RPMI)-1640 and fetal bovine serum (FBS) were purchased from GE Healthcare-Hyclone (Chicago, IL, USA). Anti-beta actin antibody (ab8227), anti-β-catenin antibody (ab16051), anti-GSK3β antibody (ab32391), anti-GSK3β (phospho-Y216) antibody (ab75745), anti-DKK1 antibody (ab61275), anti-c-Myc antibody (ab39688), and anti-Dishevelled-3 antibody (ab93850) were purchased from Abcam (Cambridge, UK). Other reagents were bought from Beijing Solarbio Science & Technology Co. Ltd. (Beijing, China). 3-(4,5-dimethylthiazol-2-yl)-2,5-diphenyl tetrazolium bromide (MTT) assay, lactate dehydrogenase (LDH), and enzyme-linked immunosorbent assay (ELISA) test was performed by Spectra Max-M5 Multifunctional Full-wavelength Microplate Reader (MD, USA). Flow cytometry detection was performed by Cytomics TM FC500 (Beckman, USA). Western blot was performed by a lab kit manufactured by Bio-Rad Laboratories (Philadelphia, PA, USA).
Animal experiment design and grouping
A total of 115 Sprague-Dawley (SD) rats (3 months old, 210±25 g) were grouped into the healthy control normal group and model group. Hepatic injury was induced by copper(II) sulfate pentahydrate (CuSO4·5H2O) gastric administration for 12 weeks in the model group (n=95). The concentration and dose of CuSO4·5H2O was 0.185% and 0.2 mL/10 g/d, while the normal control (NC) group rats (n=20) were intragastrically administered for 16 weeks with the same volume of normal saline daily. For grouping, 95 well-modeled rats were grouped into the model group (n=45), GDD group (n=25), and the PCA group (n=25), and were continuously given CuSO4·5H2O gastric administration for 4 weeks. During these 4 weeks, the GDD group and PCA group were treated with GDD (0.4 mL/10 g/d) and PCA (0.09 g/kg/d) respectively, while the model group rats were intragastrically administered with the same volume of normal saline daily. Liver tissue and whole blood were collected 10 h after the last administration. Each experiment repeated for 3 times.
Serum alanine aminotransferase (ALT) detection and liver tissue microscope-observation were used to assess the hepatic injury level. Liver tissue was fixed in 4% polycondensation formaldehyde solution and dehydrated for 12 h to make a wax block, which was sectioned at 3 µm and stained with hematoxylin and eosin (HE). The tissue wax was then observed under a microscope. This study was performed under a project license granted by the Ethics Committee for Animals Experiments of the Anhui University of Chinese Medicine, in compliance with national guidelines for the care and use of animals.
Immunochemistry of hepatic tissue
Liver tissues of each group were collected, incubated with primary antibody at 4 °C overnight after dehydration, immersed and embedded in wax, and sectioned. The secondary antibody was incubated at room temperature for 30 min. Then counterstaining, dehydrating, and mounting was performed with 3,3'-diaminobenzidine (DAB). The number of positive cells was counted by ImageJ to calculate the positive rate and the expression level of 8-hydroxyguanosine and nitrotyrosine proteins in the liver tissue of each group.
Cell culture and hepatic injury induction
The BRL-3A rat hepatic cell line was obtained from the American Type Culture Collection (ATCC) (CRL-1442), and cultured in RPMI-1640 media supplemented with 10% FBS and 1% penicillin-streptomycin mixture. Cells were cultured at 37 °C in an environment of 5% CO2 and 95% air at an appropriate growth density of 3×104–9×104.
Cell experiment design and grouping
BRL-3A cell line was treated with 1640 medium as the NC group. NC group cell was stimulated by copper(II) sulfate pentahydrate (CuSO4·5H2O) at a concentration of 325 nM (model group). Model group cell was treated with GDD serum and Wnt-inhibitor XAV-939 (respectively defined as GDD group and XAV-939 group) to explore the curative effect and exact target mechanism of GDD. The concentration of XAV-939 was 11 nM, as suggested by the Selleck official website.
Cell proliferation and cytotoxicity assay
MTT assay was used to evaluate viability in BRL-3A. Briefly, the cells were seeded in 96-well tissue culture plates at a density of 1×104 cells/well, and the outermost wells were filled with phosphate-buffered saline (PBS). Then, cell lines were treated with different doses (100, 200, 300, 400, 600, 800, 1,200, and 1,600 µM) of CuSO4·5H2O solution, PBS, and 1640 medium for 24 h. The level of dissolved formazan was quantified by detecting absorbance measurements at 570 nm (n=6). Inhibition rates were calculated by the following formula:
[1]
Inhibitory concentration 50 (IC50) was calculated according to the inhibition rate. BRL-3A was treated with PBS, 1640 medium, CuSO4·5H2O solution (concentration of IC50), and different doses (5%, 10%, 15%, and 20%) of GDD serum for 24 h. Survival rates were calculated by the following formula, and the most appropriate concentration was selected based on the results:
[2]
Flow cytometry analysis
After the BRL-3A was stimulated and treated for 24 h, cells were washed and collected by PBS solution and 0.25% trypsin-EDTA Solution. A fluorescent probe (DCFH-DA) was added to resuspended cells and incubated in the incubator for 20 min. Flow cytometry tests were then performed to detect the reactive oxygen species (ROS) level and mitochondrial membrane potential (MMP) of each group, and the peak plot was analyzed by WinMDI 2.9.
Western blot
Liver tissue and BRL-3A cell lines were collected and homogenized in radioimmunoprecipitation assay (RIPA) lysis buffer and centrifuged at 13,000 RPM and 4 °C for 10 min. Protein concentration was measured by the bicinchoninic acid (BCA) protein assay kit. After that, a 4× loading buffer was added into the protein solution and heated for 8 min to denature the protein. Then, the protein sample was loaded onto sodium dodecyl sulfate-polyacrylamide gel electrophoresis (SDS-PAGE) gels for electrophoresis and transferred to polyvinylidene difluoride membranes. After blocking with 5% fat-free dry milk in PBS containing 0.1% Tween-20, the membranes were incubated with primary antibodies at 4 °C overnight. Horseradish peroxidase-labeled secondary antibodies were used to detect the primary antibodies. Bands were visualized with enhanced chemiluminescence (ECL) kit in the automatic chemiluminescence system, and the results were analyzed with ImageJ.
Statistical analysis
Data analysis was performed using ImageJ, WinMDI (version 2.9), and SPSS 17.0. All results are presented as mean ± standard error of the mean and were compared with the group of NC and model using the Student’s t-test (unpaired, two-tailed). P<0.05 was considered statistically significant.
Results
Hepatic injury detection of copper-loaded rats
A total of 95 rats were hepatic injury-induced, and 95 models were successful, yielding a model success rate was 100%.
GDD reduced the level of ALT and copper
As shown in Figure 1, the ALT and copper results showed the following: (I) copper-loaded rats had the same impaired hepatic function as WD patients, (II) copper-loaded rats had the same copper-related biological changes as WD patients, and (III) the treatment with GDD extract strikingly ameliorated these dysfunctions. Compared with the NC rats, the ALT and copper levels of the model group rats were significantly higher (P<0.01). Compared with the model rats, these indexes in the GDD and PCA rats were significantly lower (P<0.01).
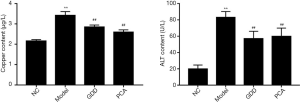
GDD ameliorated morphological and pathological changes of liver in copper-loaded rats
We collected and observed the external morphologies and pathological changes of the liver tissues from the copper-loaded rats. GDD significantly ameliorated both the morphological and pathological changes in the livers of copper-loaded rats, as shown in Figure 2.
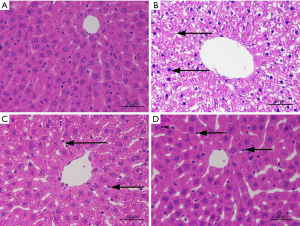
HE staining in the NC rats showed that the structural arrangement of liver tissue and cells were normal and neat (Figure 2A). Only a few hepatocytes showed granular degeneration, and the cytoplasm showed fine red particles. In the copper-loaded rats (Figure 2B), the structures of tissue and hepatocytes were disorderly and messily arranged, and most of the hepatocytes had extensive, severe vesicular degeneration and cytoplasmic vacuolation. In the GDD-treated copper-loaded rats (Figure 2C), hepatocytes were extensively denatured, and the cytoplasm and nucleus were lightly stained. Red spots were seen in the cytoplasm along with hepatic sinus dilatation and red plasma. In the PCA-treated copper-loaded rats (Figure 2D), hepatocytes were extensively denatured with granules, the cytoplasm and nucleus were lightly stained, and small red particles were seen in the cytoplasm. Compared with the copper-loaded rats, GDD- and PCA-treated copper-loaded rats had less hepatocyte degeneration, necrosis, pathological changes, and complete cell structure.
GDD reduced the expression of 8-hydroxyguanosine and nitrotyrosine
Immunochemistry was performed to detect the expression level of 8-hydroxyguanosine (Figure 3) and nitrotyrosine (Figure 4), 2 of the most important biomarkers of oxidative injury. GDD could significantly reduce the level of 8-hydroxyguanosine and nitrotyrosine in the liver of copper-loaded rats.
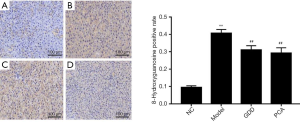
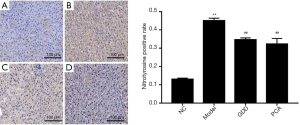
Compared with the NC rats, 8-hydroxyguanosine- and nitrotyrosine-positive expressions of liver tissue in copper-loaded rats were significantly higher (P<0.01). Compared with copper-loaded rats, 8-hydroxyguanosine- and nitrotyrosine-positive expressions in GDD and PCA-treated copper-loaded rats were significantly lower (P<0.01).
GDD ameliorated the cell viability and morphology of stimulated BRL-3A
CuSO4·5H2O was added into the BRL-3A cell line according to an incremental concentration gradient described in section “Cell proliferation and cytotoxicity assay” above. Cell viabilities were detected by MTT 24 h after CuSO4·5H2O was added (Figure 5). Results showed that the IC50 level 24 h after CuSO4·5H2O (according to the formula in section “Cell proliferation and cytotoxicity assay”) was 326 µM. GDD was added into CuSO4·5H2O-stimulated BRL-3A, and the EC50 level 24 h after was close to 20% (Figure 5). As shown in Figure 6, GDD could significantly ameliorate the viability, morphology, quantity, and density of copper-stimulated cells.
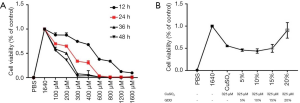
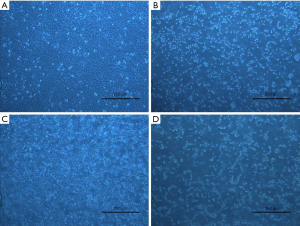
GDD reduced the expression of ROS and promoted MMP in copper-stimulated BRL-3A cells
We assessed the ROS expression and MMP after adding CuSO4·5H2O, GDD, and XAV-939 to BRL-3A. The results were analyzed 24 h after the administration of medication. Compared with NC BRL-3A, the expression of the ROS of copper-stimulated BRL-3A cells was significantly higher (P<0.01); compared with copper-stimulated BRL-3A cells, the expression of ROS was significantly lower in GDD-treated cells. Compared with the BRL-3A of the NC group, the MMP of copper-stimulated BRL-3A cells was significantly higher (P<0.01); compared with copper-stimulated BRL-3A cells, the MMP were significantly lower in GDD-treated cells. The results thus show that GDD reduced the expression of ROS (Figure 7) and promoted that of MMP (Figure 8) induced by CuSO4·5H2O.
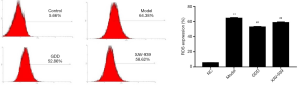
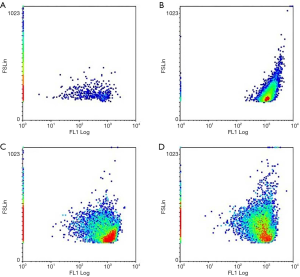
Effect of GDD on the protein expression of the Wnt/β-catenin pathway in the liver of copper-loaded rats and copper-stimulated BRL-3A
To investigate the protective effect of GDD on the copper-accumulating hepatic injury, we examined the expression of the protein, including β-catenin, GSK3β, p-GSK3β, Dishevelled-3, and c-Myc, in the livers of copper-loaded rats and copper-stimulated BRL-3A cells. The trends in the expression of the proteins for both the liver of copper-loaded rats and copper-stimulated BRL-3A cells were as follows: compared with NC, the expression of β-catenin, p-GSK3β, and c-Myc in models were significantly lower (P<0.01), while the expression of GSK3β and Dishevelled-3 were significantly higher (P<0.01). Compared with the model, the expression of β-catenin, p-GSK3β, and c-Myc in the GDD-treated model was significantly higher (P<0.01). Furthermore, the expression of GSK3β and Dishevelled-3 was significantly lower (P<0.01). The results and statistical data are shown in Figures 9,10.
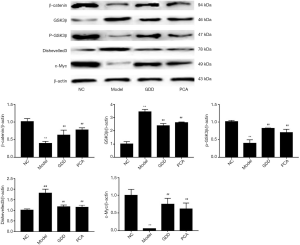
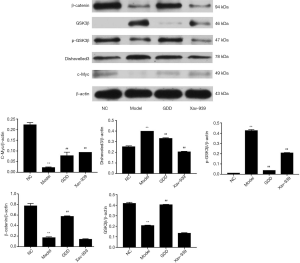
Discussion
WD is a hereditary disease caused by copper metabolism dysfunction. The pathogenic gene ATP7B is mainly expressed in the liver, and its functional defects cause ceruloplasmin and copper to be unable to be excreted from the body so that a large amount of copper accumulates in the liver first. Thus, the liver is the prime organ of the defect and the main target organ when considering treatment. Copper-loaded rats were shown to have copper metabolism disorder and liver damage performance similar to WD patients. Therefore, the copper-loaded rats are regarded as an appropriate animal model for the therapeutic research, pathogenesis, and efficacy mechanism of WD. BRL-3A cell lines separated from the liver tissue of normal rats have been found to stimulate the pathological and therapeutic process. We added CuSO4·5H2O solution to BRL-3A to simulate the pathological process of copper accumulation in hepatocytes. GDD is a Chinese medicine formula that has been widely used in WD treatment for decades. It is directed by “heat elimination, detoxification, diuresis, and viscus regulation”, and has been proven to be efficient in treating WD patients, with an effective rate of 84.1% (6). As described above, the exact mechanism of GDD’s cytoprotective effect has not been well understood. Thus, we attempted to explore the pathogenic mechanism of WD and the therapeutic theory of GDD further by combining the animal and cell experiments.
Copper is a crucial trace element in the human body, but it is also a major factor in liver injury in WD patients. Results showed that the copper content in the liver of GDD-treated copper-loaded rats was lower than that in copper-loaded rats. Results of the ALT test showed that ALT was lower after GDD treatment. Pathological observation of the liver slices indicated that there was a milder liver injury of the GDD treated copper-loaded rats. The evaluation of BRL-3A also gave evidence for the hepatic protection of GDD, which ameliorated the cell viability, morphology, quantity, and density of copper-stimulated cells. In summary, GDD showed a hepatic-protection effect in both copper-loaded rats and copper-stimulated BRL-3A cells.
Oxidative stress is regarded as a mainly pathological factor of liver or neurological dysfunction in WD (10). 8-hydroxyguanosine is a hydroxyl radical generated during oxidative stress or oxygen-containing metabolism (11). An abnormal increase in free radicals is associated with various aging and neurodegenerative diseases such as atherosclerosis, along with brain and heart ischemia-reperfusion injury (12). Nitrotyrosine is formed by peroxynitrite (ONOO−) and nitric oxide (NO) in the body, and its content can reflect the degree of oxidative stress in tissue and cells (13). The abnormal content of metal elements such as copper and iron in cells and free radicals can cause oxidative stress (14). Results showed that the levels of 8-hydroxyguanosine and nitrotyrosine the liver of copper-loaded rats were significantly lower after GDD treatment. The biomarkers of oxidative stress are ROS and MMP, with MMP being a landmark event in the early stage of apoptosis. Rising ROS level and declining MMP levels represent the deterioration of redox imbalance and the level of oxidative stress. Results showed that in copper-stimulated BRL-3A cells, ROS levels were significantly lower, and MMP levels were significantly higher after GDD treatment. Our results showed that the therapeutic effects of GDD might be related to its ability to inhibit oxidative stress.
Recent research has shown that the Wnt/β-catenin pathway has a close relationship with liver fibrosis, hepatic carcinoma, rehabilitation of cerebrovascular disease, etc. (15). This classic signal pathway was first reported by Fagotto (16) in 1997, and it is believed to be closely related to physiological processes such as growth, development, and metabolism. β-catenin and p-β-catenin proteins are important downstream links in Wnt/β-catenin signaling pathway, and p-β-catenin is a phosphorylated form of β-catenin (17). When Wnt has not been activated, β-catenin and GSK3β bind to a degradation state (18). Inhibition of GSK3β can change this binding state with β-catenin protein. p-GSK3β, as a phosphorylated form of GSK3β, can improve the decrease of GSK3β and can dephosphorylate the target protein while increasing the expression level when the Wnt signal is activated (19). c-Myc protein is an important DNA-binding protein in the nucleus (20), and its overexpression can cause apoptosis (21). Dishevelled-3 can react with receptor proteins, phosphatases, and kinases (22). When it is activated, it can inhibit GSK3β, increase β-catenin protein expression, and activate the Wnt/β-catenin signaling pathway (23). The experimental results showed that the expression of β-catenin, p-GSK3β, and c-Myc proteins in the liver of copper-loaded rats, and copper-stimulated cells were significantly lower than of the NC rats and normal BRL-3A cells, and the expression levels of GSK3β and Dishevelled-3 were significantly increased. Thus, the inactivation of the Wnt/β-catenin signaling pathway might be one of the pathological changes of liver injury in WD. Copper accumulation in liver and hepatocytes induce toxicity injury while the inactivation of the Wnt/β-catenin signaling pathway prevents physiological self-healing, which intensifies copper-induced hepatic injury. After GDD medication, the expression of β-catenin, p-GSK3β, and c-Myc proteins in the liver of copper-loaded rats and copper-stimulated BRL-3A cells was significantly upregulated, while the expression of GSK3β and Dishevelled-3 was significantly downregulated. These results clearly show that GDD can rectify the expression of proteins in the Wnt/β-catenin signaling pathway.
Past research has confirmed that Wnt/β-catenin signaling pathway is closely related to oxidative stress (24-26). This study found that a high concentration of copper inhibited Wnt/β-catenin signaling pathway and induced oxidative stress injury in rat liver and hepatocyte cell lines. Meanwhile, GDD reduced the copper content and ALT level in the liver of copper-loaded rats, and the activation of the Wnt/β-catenin signaling pathway improved the self-repair of the liver and hepatocytes, thereby reducing the hepatic oxidative stress-related injury. Although the copper-chelating effect of PCA is better than GDD, the regulation of the GSK3β and DKK1 proteins was not as significant as GDD. Xav-939 is a widely accepted inhibitor of the Wnt/β-catenin signaling pathway; however, its application could not undo the therapeutic effect of GDD in this study. A lack of consistency in the trends observed suggests that the hepatic-protection effect of GDD does not only arise from its copper-expelling ability, as the inactivation of Wnt/β-catenin signaling pathway was triggered by a high concentration of copper.
Furthermore, when Wnt/β-catenin signaling pathway was completely inhibited, the hepatic-protection effect was still effective. This result implies that the therapeutic effect of GDD is independent of Wnt/β-catenin signaling pathway. Multiple-therapeutic-targeting is a unique characteristic of Chinese medicinal formulae, and GDD is no exception. It is hoped that this insight can further propel research into the therapeutic mechanism of GDD, which warrants further study.
Acknowledgments
Funding: This work was supported by the National Natural Science Foundation of China (No. 81673948).
Footnote
Reporting Checklist: The authors have completed the ARRIVE reporting checklist. Available at http://dx.doi.org/10.21037/apm-20-301
Data Sharing Statement: Available at http://dx.doi.org/10.21037/apm-20-301
Conflicts of Interest: All authors have completed the ICMJE uniform disclosure form (available at http://dx.doi.org/10.21037/apm-20-301). All authors report grants from National Natural Science Foundation of China, during the conduct of the study.
Ethical Statement: The authors are accountable for all aspects of the work in ensuring that questions related to the accuracy or integrity of any part of the work are appropriately investigated and resolved. Experiments were performed under a project license granted by the Ethics Committee for Animals Experiments of the Anhui University of Chinese Medicine (No. IACUC19001), in compliance with national guidelines for the care and use of animals.
Open Access Statement: This is an Open Access article distributed in accordance with the Creative Commons Attribution-NonCommercial-NoDerivs 4.0 International License (CC BY-NC-ND 4.0), which permits the non-commercial replication and distribution of the article with the strict proviso that no changes or edits are made and the original work is properly cited (including links to both the formal publication through the relevant DOI and the license). See: https://creativecommons.org/licenses/by-nc-nd/4.0/.
References
- Huster D. Wilson disease. Internist (Berl) 2018;59:159-74. [PubMed]
- Cheng N, Wang K, Hu W, et al. Wilson disease in the South chinese han population. Can J Neurol Sci 2014;41:363-7. [PubMed]
- Zhong HJ, Sun HH, Xue LF, et al. Differential hepatic features presenting in Wilson disease-associated cirrhosis and hepatitis B-associated cirrhosis. World J Gastroenterol 2019;25:378-87. [Crossref] [PubMed]
- Kim B, Chung SJ, Shin HW. Trientine-induced neurological deterioration in a patient with Wilson's disease. J Clin Neurosci 2013;20:606-8. [Crossref] [PubMed]
- Liu Y, Cheng MM, Dong JJ, et al. Intestinal absorptive characteristics of ingredients from ethanol extracts of Gandou decoction by rat everted intestinal sac models. Zhongguo Zhong Yao Za Zhi 2017;42:2571-6. [PubMed]
- Xue BC, Yang RM, Hu JY. Effect of Gandou Decoction IV combined with short-term decoppering therapy with sodium dimercapto-sulphonate on serum indexes of hepatic fibrosis in patients with Wilson' s disease. Zhongguo Zhong Xi Yi Jie He Za Zhi 2007;27:785-8. [PubMed]
- Khosla R, Rastogi A, Ramakrishna G, et al. EpCAM+ Liver Cancer Stem-Like Cells Exhibiting Autocrine Wnt Signaling Potentially Originate in Cirrhotic Patients. Stem Cells Transl Med 2017;6:807-18. [Crossref] [PubMed]
- Reabroi S, Chairoungdua A, Saeeng R, et al. A silyl andrographolide analogue suppresses Wnt/β-catenin signaling pathway in colon cancer. Biomed Pharmacother 2018;101:414-21. [Crossref] [PubMed]
- Cheng M, Wu H, Wu H, et al. Metabolic profiling of copper-laden hepatolenticular degeneration model rats and the interventional effects of Gandou decoction using UPLC-Q-TOF/MS. J Pharm Biomed Anal 2019;164:187-95. [PubMed]
- Nagasaka H, Inoue I, Inui A, et al. Relationship between oxidative stress and antioxidant systems in the liver of patients with Wilson disease: hepatic manifestation in Wilson disease as a consequence of augmented oxidative stress. Pediatr Res 2006;60:472-7. [PubMed]
- Riveiro-Naveira RR, Valcárcel-Ares MN, Almonte-Becerril M, et al. Resveratrol lowers synovial hyperplasia, inflammatory markers and oxidative damage in an acute antigen-induced arthritis model. Rheumatology (Oxford) 2016;55:1889-900. [Crossref] [PubMed]
- Stanicka J, Russell EG, Woolley JF, et al. NADPH oxidase-generated hydrogen peroxide induces DNA damage in mutant FLT3-expressing leukemia cells. J Biol Chem 2015;290:9348-61. [Crossref] [PubMed]
- Tabak O, Gelisgen R, Erman H, et al. Oxidative lipid, protein, and DNA damage as oxidative stress markers in vascular complications of diabetes mellitus. Clin Invest Med 2011;34:E163-71. [Crossref] [PubMed]
- Uversky VN, Li J, Fink AL. Metal-triggered structural transformations, aggregation, and fibrillation of human alpha-synuclein. A possible molecular NK between Parkinson's disease and heavy metal exposure. J Biol Chem 2001;276:44284-96. [Crossref] [PubMed]
- Kleszcz R. The canonical Wnt pathway. Postepy Biochem 2019;65:183-92. [Crossref] [PubMed]
- Fagotto F, Guger K, Gumbiner BM. Induction of the primary dorsalizing center in Xenopus by the Wnt/GSK/beta-catenin signaling pathway, but not by Vg1, Activin or Noggin. Development 1997;124:453-60. [PubMed]
- Stamos JL, Weis WI. The β-catenin destruction complex. Cold Spring Harb Perspect Biol 2013;5:a007898. [Crossref] [PubMed]
- Li VS, Ng SS, Boersema PJ, et al. Wnt signaling through inhibition of β-catenin degradation in an intact Axin1 complex. Cell 2012;149:1245-56. [Crossref] [PubMed]
- Hur EM, Zhou FQ. GSK3 signalling in neural development. Nat Rev Neurosci 2010;11:539-51. [Crossref] [PubMed]
- Gardner LB, Corn PG. Hypoxic regulation of mRNA expression. Cell Cycle 2008;7:1916-24. [Crossref] [PubMed]
- Schmidt EV. The role of c-myc in regulation of translation initiation. Oncogene 2004;23:3217-21. [Crossref] [PubMed]
- De Marco P, Merello E, Consales A, et al. Genetic analysis of disheveled 2 and disheveled 3 in human neural tube defects. J Mol Neurosci 2013;49:582-8. [Crossref] [PubMed]
- Petersen CP, Reddien PW. Wnt signaling and the polarity of the primary body axis. Cell 2009;139:1056-68. [Crossref] [PubMed]
- Ebrahimi KB, Cano M, Rhee J, et al. Oxidative Stress Induces an Interactive Decline in Wnt and Nrf2 Signaling in Degenerating Retinal Pigment Epithelium. Antioxid Redox Signal 2018;29:389-407. [Crossref] [PubMed]
- Vallée A, Lecarpentier Y. Crosstalk Between Peroxisome Proliferator-Activated Receptor Gamma and the Canonical WNT/β-Catenin Pathway in Chronic Inflammation and Oxidative Stress During Carcinogenesis. Front Immunol 2018;9:745. [Crossref] [PubMed]
- Mishra A, Singh S, Tiwari V, et al. Dopamine receptor activation mitigates mitochondrial dysfunction and oxidative stress to enhance dopaminergic neurogenesis in 6-OHDA lesioned rats: A role of Wnt signalling. Neurochem Int 2019;129:104463. [Crossref] [PubMed]