Impact of permissive hypercapnia on regional cerebral oxygen saturation and postoperative cognitive function in patients undergoing cardiac valve replacement
Introduction
Postoperative cognitive dysfunction (POCD) is a common complication after cardiac surgery, occurring in 23–81% of patients undergoing cardiac surgery. Factors including cerebral embolism and hypoperfusion during cardiac surgery and nonpulsatile blood flow during cardiopulmonary bypass (CPB) may all lead to POCD (1). POCD has become a research hotspot in the recent years since it seriously impacts prognosis and postoperative quality of life. Permissive hypercapnia (PHC) is a lung-protective ventilation strategy whose efficacy has been proven in recent years. This method can improve patients’ cerebral oxygen metabolism and reduce the incidence of POCD, playing a protective and anti-inflammatory role in vital organs, including the heart and central nervous system (CNS) (2,3).
In recent years, near-infrared spectroscopy (NIRS) has been widely used to monitor regional cerebral oxygen saturation (rSO2) during surgical anesthesia since a decrease in cerebral oxygen saturation is associated with hypotension or cerebral anoxia during organ reperfusion (4). This study aimed to investigate the impact of the PHC ventilation strategy on rSO2 and postoperative cognitive function in patients undergoing cardiac valve replacement (CVR).
We present the following article in accordance with the CONSORT reporting checklist (available at http://dx.doi.org/10.21037/apm-20-2090).
Methods
Clinical data
A total of 66 patients undergoing CVR between January 2019 and March 2019 in our hospital were enrolled in this study, including 31 males and 35 females, with an age range of 40–74 years and American Society of Anesthesiologists class II or III. The patients were randomly divided into the PHC ventilation group (Group H) and conventional mechanical ventilation group (Group C) using a random number table. Inclusion criteria were as follows: the patient was scheduled to undergo CVR, had no surgical contraindications, was conscious and able to think independently, and had no diseases causing elevated intracranial pressure, such as cerebral hemorrhage; and the patient had a cardiac function grade ≤ III and a left ventricular ejection fraction (LVEF) ≥45%. Exclusion criteria were as follows: history of cranial cerebral trauma surgery; history of cerebral infarction, stroke, or other CNS diseases; severe diseases of vital organs, such as the liver or kidney; mental diseases or poor consciousness; and inability to undergo neuropsychological assessments due to severe visual or hearing impairment. The study was conducted in accordance with the Declaration of Helsinki (as revised in 2013). This study was approved by the ethics committee of our hospital (No. kssy2019-30). Each patient understood this study and voluntarily signed an informed consent form.
Anesthesia monitoring
After the patient entered the operating room, a peripheral venous access was established, and a face mask was given for oxygen. The patient was routinely monitored for electrocardiography, pulse oximetry, heart rate (HR), and noninvasive blood pressure, and the invasive arterial blood pressure was continuously monitored through radial arterial puncture under local anesthesia. The left forehead rSO2 was monitored by NIRS (Covidien INVOS 5100C). The consciousness index (cerebral state index, CSI) was continuously monitored by an anesthesia depth monitor (Danmeter, Denmark). After tracheal intubation, an 8.5-Fr double-lumen central venous catheter was placed into the right internal jugular vein, and the central venous pressure (CVP) was monitored. Cardiac function was monitored by transesophageal echocardiography, and cardiac output (CO) was measured by color Doppler flow imaging through the left ventricular outflow tract.
Anesthesia induction and maintenance
Anesthesia was induced by 0.05 mg/kg midazolam, 0.8 µg/kg sufentanil, 1–2 mg/kg propofol, and 0.2 mg/kg cisatracurium besylate. After tracheal intubation, the patient was subjected to intermittent positive-pressure breathing, with the tidal volume at 8–10 mL/kg, inspiratory:expiratory (I:E) ratio =1:2, breathing rate at 10–12 breaths/min, and fraction of inspired oxygen (FiO2) at 70%. The end-tidal carbon dioxide (PETCO2) was maintained at 35–45 mmHg. Anesthesia was maintained in both groups by 0.2–0.4 µg·kg−1·min−1 remifentanil, 0.2–0.3 mg·kg-1·h-1 cisatracurium besylate, and 3–4 mg·kg−1·h−1 propofol through an intravenous infusion pump. During the surgery, an appropriate amount of sufentanil was intermittently injected intravenously at 0.5–1.0 µg/kg. The CSI (anesthesia depth) was maintained between 40 and 60.
Ventilation mode management
Respiratory parameter settings and implementation of PHC: (I) Group C: after CPB was stopped, the patient continued to receive conventional mechanical ventilation with the tidal volume at 8–10 mL/kg, I:E=1:2, and respiratory rate at 10–12/min. The respiratory parameters were adjusted based on the difference between PETCO2 and the arterial partial pressure of carbon dioxide (PaCO2), so that PaCO2 was kept at 35–45 mmHg. (II) Group H: thirty minutes after stopping CPB and when the circulation function was stable, the patient was subjected to PHC ventilation with the tidal volume of 6–8 mL/kg, I:E =1:2, FiO2 =70%, and respiratory rate 10–15/min. During the surgery, the respiratory parameters were adjusted based on the difference between PETCO2 and PaCO2, so that PaCO2 was maintained at 46–60 mmHg. With CPB, PaCO2 was maintained at 35–45 mmHg according blood gas analysis.
Indicator recording
The HR, mean arterial pressure (MAP), CO, rSO2 and other indicators were recorded at five time points: before induction of anesthesia (T0), before chest opening (T1), 30 min into the CPB (T2), 30 min after CPB (T3), and at suture (T4). Blood samples were collected from the radial artery at the above time points for blood gas analysis, and the pH, PaCO2, PaO2, lactate (Lac), and blood glucose levels were recorded. The Mini-Mental State Examination (MMSE) score was recorded 1 d before surgery and 24 h and 7 d after surgery. Patients with a postoperative MMSE 2 points lower than baseline were diagnosed with POCD. All tests were completed by the same physician.
Statistical analysis
Calculation of sample size: Stata software was used for power analysis. The lower limit for normal rSO2 was 60 (%), and a 5-percentage-point increase in rSO2 (to 65%) was clinically significant. The standard deviation was 7 (%), with alpha =0.05 and power =0.8. Calculations showed that the sample size of each group needed to be 31. Data were analyzed by SPSS 21.0 statistical software. Qualitative data are expressed as percentages, and the chi-squared test was used for comparison between groups. Quantitative data conforming to a normal distribution are expressed as
Results
This study included a total of 66 patients, 33 in each group. No significant differences in the age, body weight, body surface area (BSA), New York Heart Association (NYHA) classification, aortic cross-clamping time (ACCT), CPB duration, or surgery duration were detected between the two groups (P>0.05, Table 1).
Comparison of hemodynamic parameters at each time point between the two groups
Group H had a lower HR at T0 and T1 (P<0.05) and higher CO at T3 and T4 than Group C (P<0.05). Inter- and intragroup comparisons of other hemodynamic parameters did not show any statistically significant differences (P>0.05) (Table 2).
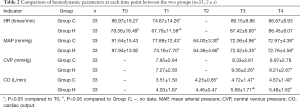
Full table
Comparison of rSO2 and relevant indicators at each time point between the two groups
Group H had higher rSO2 at T4 (P<0.05), lower pH and Lac at T4 (P<0.05), higher PaCO2 at T3 and T4 (P<0.05), and lower PaO2 at T3 and T4 than Group C (P<0.05). Intergroup comparisons of other relevant data did not show any statistically significant differences (P>0.05) (Table 3, and Figures 1-3).
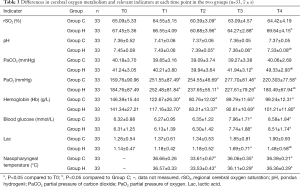
Full table
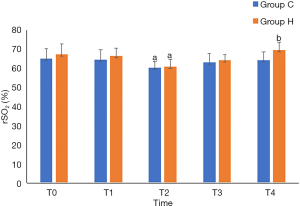
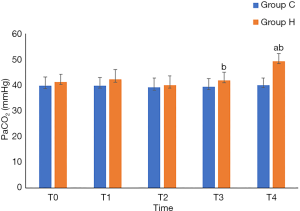
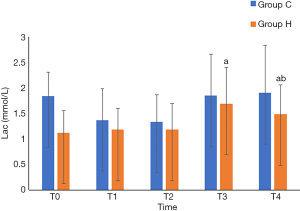
Comparison of MMSE scores at each time point between the two groups
Compared to their MMSE scores 1 d before the surgery, the MMSE scores at 24 h after surgery decreased in both groups (P<0.05). Group H had a higher MMSE score than Group C 24 h after surgery (P<0.05) (Table 4 and Figure 4). Compared to that at 1 d before surgery, the incidence of POCD in each group of patients differed significantly at 24 h after surgery (P<0.05). The POCD incidence also differed significantly between the two groups at 24 h after surgery (Table 5).
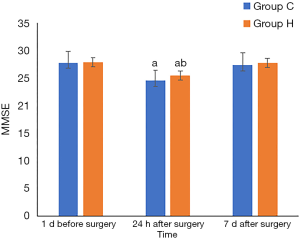
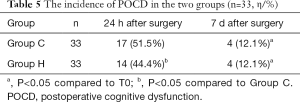
Full table
Discussion
Cardiac valvular disease is a common clinical disease, valve replacement is the main surgical treatment of heart valve disease, its indications mainly have the following categories: heart valve serious diseases, such as valve stenosis or closed incomplete; the postoperative complications of degenerative valvular disease in the elderly; the possible complications include: low cardiac output syndrome; severe arrhythmia; postoperative infection; perivalvular leakage of artificial valves. Many factors can cause lung injury in cardiac surgery, including general anesthesia, CPB, and intrathoracic surgical procedures. Under these circumstances, a lung-protective ventilation strategy is believed to play a critical role in lung protection, and it can reduce postoperative complications, increase safety, and accelerate recovery. Lung-protective ventilation strategies have received extensive attention from anesthesiologists. PHC is an important lung-protective ventilation strategy, as it not only increases PaCO2 within an acceptable range, reduces tidal volume, “allows” hypercapnia, and prevents lung damage from high tension but also has protective and anti-inflammatory effects on the heart, nervous system, and many other organs (5,6). A previous study (7) showed that mild hypercapnia (PaCO2 at 45–55 mmHg) increased rSO2, improved cerebral oxygenation, and reduced the incidence of POCD. The decrease in intraoperative rSO2 is significantly associated with early POCD. Therefore, this study applied a lung-protective ventilation strategy to patients undergoing CVR and investigated the impact of PHC on rSO2 and postoperative cognitive function of patients.
NIRS is a novel rSO2 monitoring method that enables real-time, continuous, noninvasive monitoring of the oxygenation and metabolism of the brain tissue. This method is not affected by nonpulsatile blood flow, low temperature, or other factors, and it has been extensively applied in anesthesia for cardiac surgery (8). Frogel et al. (9) believed that monitoring cerebral oxygen saturation during cardiac surgery can indirectly reflect the status of brain tissue perfusion, and effective monitoring can guide the implementation of intraoperative brain protection measures. rSO2 monitoring can effectively prevent cardiac surgery-related POCD, since insufficient brain oxygen supply during surgery is one of the causes of POCD (10,11). As a sensitive indicator of cerebral oxygen metabolism, rSO2 can monitor cerebral ischemia and hypoxia in real time and predict the risk of POCD after cardiac surgery. Using rSO2 to guide intraoperative management can improve the prognosis of patients undergoing surgery. It is generally believed that the normal baseline rSO2 among patients undergoing cardiac surgery is approximately 66% (12). Patients are diagnosed with cerebral hypoxia if intraoperative rSO2 is less than 50% or decreases more than 20% from the baseline for 1 min or more (13). Under these circumstances, clinicians should intervene promptly to prevent damage to the CNS. In this study, the MAP was maintained at 60–80 mmHg in all patients, which ensured effective cerebral perfusion. Compared to the rSO2 at T0 (before the surgery), the rSO2 in both groups dropped significantly during CPB (P<0.05). The rSO2 of three patients decreased more than 20% compared to the baseline in a short period (one patients in Group C and two patients in Group H), but it was returned to normal range by CPB and increasing the perfusion pressure promptly. At the postoperative 7-d follow-up of these three patients, no significant damage to the CNS or reduction in cognitive function were detected. The intraoperative reduction in rSO2 may be related to blood dilution due to CPB.
The results of this study showed that the Hb level was significantly lower than the preoperative level at each time point after CPB. Excluding the impacts of other relevant factors, blood dilution during CPB causes reductions in hematocrit and Hb, and insufficient blood oxygen-carrying capacity directly lowers the oxygen supply to the brain tissue. Here, Group H had a significantly higher rSO2 (P<0.05), indicating that PHC is conducive to improving the autoregulation of the cerebral blood flow and the cerebral oxygen supply/consumption balance, which is consistent with a previous study (7). The reason may be that since CO2 is the most effective regulator of cerebral blood flow, the PHC ventilation strategy in the patients undergoing CVR increases PaCO2, catalyzes the activity of neuronal nitric oxide synthase, increases nitric oxide production, causes the expansion of cerebral blood vessels, and increases cerebral blood flow and cerebral oxygen supply, thereby reducing the risk of cerebral ischemia and hypoxia and effectively protecting brain function (14).
POCD is characterized by changes in perception, recognition, thinking, and memory after anesthesia or surgery. It mainly manifests as a continuous decline in cognitive function and the occurrence of mental confusion, anxiety, personality changes, and memory impairment, accompanied by lower social activity. A previous study (15) reported that at one week after cardiac surgery, 53% of the patients showed POCD. There are a variety of methods to assess postoperative cognitive function. As the main tool for cognitive decline screening, MMSE has high specificity and sensitivity and is appropriate for the assessment of cognitive function after cardiac surgery. Both of our groups had significantly lower MMSE scores 24 h after than before surgery (P<0.05) though the MMSE scores 7 d after surgery were not significantly different, indicating that the early POCD may be mainly caused by anesthetics, surgical stress and postoperative analgesia. At 7 d after surgery, the cognitive function of our patients had returned to the level before surgery.
In cardiac surgery, the incidence of POCD is impacted by multiple factors, including age, previous diseases, anesthetics, intraoperative hypothermia, cerebrovascular insufficiency, cerebral embolism, and systemic inflammation. Currently, age is believed to be the main factor for the occurrence of POCD (16). No significant differences were detected in the age, body weight, BSA, NYHA, nasopharyngeal temperature, blood glucose, ACCT, CPB duration, or surgery duration between our two groups. Keeping the CSI (anesthetic depth) at 40–60 during surgery reduced the influence of objective external factors on the results. The decreased MMSE scores at 24 h but normal MMSE scores at 7 d suggest that early POCD may be mainly caused by multiple factors, including anesthetics, surgical stress, postoperative analgesia, and the use of analgesic drugs. Group H had a significantly higher MMSE score at 24 h after surgery than Group C (P<0.05), and the two groups’ scores at 7 d were not significantly different. These results indicate the PHC can help improve early POCD. The underlying mechanism may be that hypercapnia can reduce intracellular pH, promote the oxidative utilization of glucose by increasing the concentration of adenosine and adenosine triphosphate, and maintain the reserve of energy-rich phosphoric acid in the brain tissue, thus protecting the brain and reducing the incidence of POCD.
Many studies on POCD are from large centers with large sample sizes. The limitations of this study are that the sample size was relatively small, and long-term follow-up data were not available. In the future, biomarkers related to cognitive impairment can be included to more deeply investigate the impact of the PHC ventilation strategy on rSO2 and POCD.
In summary, this study showed that PHC can increase rSO2 and improve cerebral blood flow and the cerebral oxygen supply/consumption balance in patients undergoing CVR, thus having a brain protection effect. However, a significant effect of PHC on the incidence of POCD was not found in this study.
Acknowledgments
Funding: None.
Footnote
Reporting Checklist: The authors have completed the CONSORT reporting checklist. Available at http://dx.doi.org/10.21037/apm-20-2090
Data Sharing Statement: Available at http://dx.doi.org/10.21037/apm-20-2090
Conflicts of Interest: All authors have completed the ICMJE uniform disclosure form (available at http://dx.doi.org/10.21037/apm-20-2090). The authors have no conflicts of interest to declare.
Ethical Statement: The authors are accountable for all aspects of the work in ensuring that questions related to the accuracy or integrity of any part of the work are appropriately investigated and resolved. The study conformed to the provisions of the Declaration of Helsinki (as revised in 2013). This study was approved by the ethics committee of our hospital (No. kssy2019-30). Each patient understood this study and voluntarily signed an informed consent form.
Open Access Statement: This is an Open Access article distributed in accordance with the Creative Commons Attribution-NonCommercial-NoDerivs 4.0 International License (CC BY-NC-ND 4.0), which permits the non-commercial replication and distribution of the article with the strict proviso that no changes or edits are made and the original work is properly cited (including links to both the formal publication through the relevant DOI and the license). See: https://creativecommons.org/licenses/by-nc-nd/4.0/.
References
- Holmgaard F, Vedel AG, Rasmussen LS, et al. The association between postoperative cognitive dysfunction and cerebral oximetry during cardiac surgery: a secondary analysis of a randomised trial. Br J Anaesth 2019;123:196-205. [Crossref] [PubMed]
- Contreras M, Masterson C, Laffey JG. Permissive hypercapnia: what to remember. Curr Opin Anaesthesiol 2015;28:26-37. [Crossref] [PubMed]
- Li L, Geng L, Ma C, et al. Effect of permissible hypercapnia on cognitive function after carotid endarterectomy. Int J Anesth Resus 2019;40:1051-5.
- Chan MJ, Chung T, Glassford NJ, et al. Near-Infrared Spectroscopy in Adult Cardiac Surgery Patients: A Systematic Review and Meta-Analysis. J Cardiothorac Vasc Anesth 2017;31:1155-65. [Crossref] [PubMed]
- Hummler HD, Banke K, Wolfson MR, et al. The Effects of Lung Protective Ventilation or Hypercapnic Acidosis on Gas Exchange and Lung Injury in Surfactant Deficient Rabbits. PLoS One 2016;11:e0147807. [Crossref] [PubMed]
- He M, Chen Y. Effects of permissive hypercapnia on lung function and reaction to collapsed pneumonia after one-lung ventilation. Journal of Clinical Anesthesiology 2015;(12):1172-5.
- Wong C, Churilov L, Cowie D, et al. Randomised controlled trial to investigate the relationship between mild hypercapnia and cerebral oxygen saturation in patients undergoing major surgery. BMJ Open 2020;10:e029159. [Crossref] [PubMed]
- Sørensen H. Near infrared spectroscopy evaluated cerebral oxygenation during anesthesia. Dan Med J 2016;63:B5318. [PubMed]
- Frogel J, Kogan A, Augoustides JGT, et al. The Value of Cerebral Oximetry Monitoring in Cardiac Surgery: Challenges and Solutions in Adult and Pediatric Practice. J Cardiothorac Vasc Anesth 2019;33:1778-84. [Crossref] [PubMed]
- Murniece S, Soehle M, Vanags I, et al. Near Infrared Spectroscopy Based Clinical Algorithm Applicability During Spinal Neurosurgery and Postoperative Cognitive Disturbances. Medicina (Kaunas) 2019;55:179. [Crossref] [PubMed]
- Ortega-Loubon C, Herrera-Gómez F, Bernuy-Guevara C, et al. Near-Infrared Spectroscopy Monitoring in Cardiac and Noncardiac Surgery: Pairwise and Network Meta-Analyses. J Clin Med 2019;8:2208. [Crossref] [PubMed]
- Deschamps A, Hall R, Grocott H, et al. Cerebral Oximetry Monitoring to Maintain Normal Cerebral Oxygen Saturation during High-risk Cardiac Surgery: A Randomized Controlled Feasibility Trial. Anesthesiology 2016;124:826-36. [Crossref] [PubMed]
- Kumpaitiene B, Svagzdiene M, Drigotiene I, et al. Correlation among decreased regional cerebral oxygen saturation, blood levels of brain injury biomarkers, and cognitive disorder. J Int Med Res 2018;46:3621-9. [Crossref] [PubMed]
- Nayak S, Jindal A. Permissive hypercapnia: Is there any upper limit? Indian J Crit Care Med 2015;19:56-7. [Crossref] [PubMed]
- Glumac S, Kardum G, Karanovic N. Postoperative Cognitive Decline After Cardiac Surgery: A Narrative Review of Current Knowledge in 2019. Med Sci Monit 2019;25:3262-70. [Crossref] [PubMed]
- Jungwirth B, Zieglgänsberger W, Kochs E, et al. Anesthesia and postoperative cognitive dysfunction (POCD). Mini Rev Med Chem 2009;9:1568-79. [Crossref] [PubMed]