Sphingosine 1-phosphate and osteoporosis: pathophysiology and therapeutic aspects—a narrative review
Introduction
Sphingosine 1-phosphate (S1P) is a bioactive sphingolipid containing 2-amino-1,3-dihydroxy-octadec-4-ene. Sphingolipids are specific cellular lipids in eukaryotes and are characterized by a particular aliphatic amino alcohol known as sphingosine (1-3). S1P is produced in the cytosol and the nucleus in a variety of cells via the phosphorylation of two sphingosine kinases (SPHK1 and SPHK2 or simply SK1 and SK2, respectively). S1P-specific phosphatases can dephosphorylate S1P to form sphingosine, or S1P lyase enzyme (SPL1) can irreversibly degrade S1P to form fatty aldehyde and phosphoethanolamine (4-6). Increased levels of S1P are found in the circulation, whereas S1P levels are very low in most other tissues. Biologically active S1P regulates multiple cellular processes, including cell differentiation, migration, proliferation, morphogenesis, cytoskeletal organization, adhesion, tight junction assembly, apoptosis and the localization of various cell types (Figure 1) (7,10).
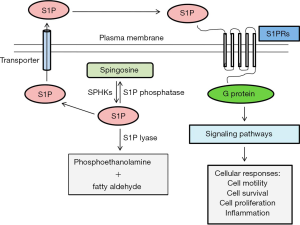
S1P has been identified as a major regulator of cell trafficking in immune systems. Moreover, S1P may act as a coupling factor in bone metabolism (11-13). Formerly, S1P was thought to be an intracellular second messenger that connects and maintains the activity of different intracellular regulatory molecules. Later, it was discovered that S1P was an extracellular ligand for G protein-coupled receptors of the EDG family, which transfer signals to the inside of the cell (8,14). Cell surface receptors regulate the activity of S1P, and S1P signalling has been regarded as a model of inside-out signalling. S1P is produced inside the cell and exported to the extracellular space. Endothelial cells release S1P in the extracellular milieu through specific types of transporters, including ATP-binding cassette (ABC) receptors, Spinster Homologue 2 (SPNS2) or major facilitator superfamily transporter 2b (Mfsd2b), and S1P binds to and transfers signals through one of the five specific G protein-coupled receptors on the cell surface (S1PR1, S1PR2, S1PR3, S1PR4, and S1PR5) to promote various types of cellular functions (9,15-17).
S1P plays an important role in maintaining bone metabolism, including osteoporosis. This review article focuses on the role of S1P in maintaining bone homeostasis and how S1P could serve as a diagnostic and therapeutic target in osteoporosis. We present the following article in accordance with the Narrative Review reporting checklist (available at http://dx.doi.org/10.21037/apm-20-1255).
Role of S1P in bone metabolism
S1P and its metabolic precursor ceramide are the central molecules of sphingolipid metabolism. Ceramide mainly controls the balance between the levels of sphingosine and S1P and a phenomenon termed sphingolipid rheostat (6,18). Ceramide/S1P metabolism can determine whether a cell will die or proliferate. This metabolic pathway is associated with skeletal development, bone mineralization and bone homeostasis. However, S1P can play a critical role in various bone diseases, including osteoarthritis, osteoporosis, rheumatoid arthritis, spondyloarthritis and bone cancer and metastasis (19).
Bone undergoes continuous remodelling through the removal of aged bone by osteoclasts and new bone formation by osteoblasts. Regulation of these activities is essential for maintaining consistent bone quality and quantity. In the remodelling process, cellular balances between bone formation and resorption are critical and strictly controlled by many factors (20-22). S1P exerts receptor-mediated effects on the proliferation, differentiation and migration of both osteoclasts and osteoblasts. S1P stimulates bone formation and plays an essential role in bone homeostasis by targeting osteoclastogenesis and osteogenesis. S1P released by cells into the extracellular environment binds with S1P receptors on osteoblasts, leading to an increase in RANKL expression through the induction of COX2. The enhanced RANKL in osteoblasts then binds with more RANK and mediates osteoclast differentiation. Extracellular S1P also regulates bone homeostasis by coupling osteoclast and osteoblast activity (17,23).
However, in pathological conditions, this equilibrium is disrupted. The removal of aged bone is the distinctive function of osteoclasts. Osteoclasts establish an isolated microenvironment between themselves and bone by developing a specialized cytoskeleton. The bone resorption ability of osteoclasts is controlled by different factors (20,24). S1P has been shown to control the migration of osteoclast precursors via G protein-coupled receptors for S1P (S1PRs). S1P keeps osteoclast precursors away from bone surfaces to reduce bone degradation and prevent bone loss (22,25,26).
Osteoporosis: an age old problem in bone
Bones are living tissue, and aged bone tissue is continually absorbed, while osteophytes are generated to regulate normal bone mineral homeostasis. In an older person, bone resorption occurs faster than bone formation (27,28). Osteoporosis is a disease in which there is a disturbance in the balance between the formation and resorption of bone. The bones become more permeable, fragile and prone to fracture, especially in the hip, wrists or spinal vertebrae (29,30). Osteoporosis can affect both sexes, but women suffer mostly from reduced levels of oestrogen after menopause (31,32). Osteoporosis develops slowly, and typically, there are no symptoms in the early stage. However, once bones have been weakened by osteoporosis, patients might show signs and symptoms, including back pain, loss of stature, and a curved posture, and there is a risk of bone fracture (33,34). Several osteoporosis risk factors have been identified. Some are modifiable, and some are not modifiable, including age, sex, ethnicity, lifestyle, height, weight, genetic factors, and fracture history (35,36). Patients with osteoporosis may change lifestyle, supplementation, and appropriate medical treatment to achieve the desired goals. Medical treatment of osteoporosis can help to impede disease progression, reduce pain, prevent fractures, and maintain healthy bone mineral density (BMD) and bone mass (37-39).
S1P in osteoporosis
S1P is a lipid molecule that has a regulatory effect on bone metabolism. S1P has been shown to have multiple contributions to osteoclast and osteoblast crosstalk and can play an essential role in maintaining the balance between bone resorption and formation. Disturbances in the activities of osteoblasts and osteoclasts may induce various types of metabolic bone disorders, including osteoporosis (5,40). Increased levels of S1P are responsible for reduced levels of BMD and increased levels of bone resorption markers (BRMs), which are associated with an increased risk of osteoporosis (41,42). These risk factors are especially frequent in postmenopausal females. S1P affects osteoclasts to activate TGF-β1, resulting in an increased possibility of bone resorption (Figure 2) (43,44). Ota et al. (43) examined the effects of TGF-β1 on osteoclast-mediated cell mineralization. During resorption, the level of TGF-β1 is increased, leading to the production of osteoclasts to restore bone loss (43).
Therapeutic role of S1P in osteoporosis
Osteoporosis is a progressive bone disorder, but therapeutic agents that stimulate new bone formation are very limited. An improved understanding of S1P receptor biology may facilitate the development of new therapeutic strategies for osteoporosis (45,46). S1P is a potential therapeutic target for treating osteoporosis by controlling the migration of osteoclast precursors, vigorously maintaining the bone mineralization process, and playing a critical regulatory role during osteoclastogenesis (25,47).
It has been revealed that blocking S1P lyase can increase S1P levels and stimulate the differentiation of osteoblasts through S1PR2 in mouse osteoporosis models (48,49). Weske et al. further showed that increasing the level of S1P by inhibiting S1P lyase enhanced bone formation and potently stimulated osteoblastogenesis by inducing adipogenesis and inhibited osteoclastogenesis by inducing osteoprotegerin production (50). Weske et al. (48) found that the S1P2 agonist CYM5520 exerted osteoanabolic effects and counteracted the decrease in bone quality in ovariectomized mice. Therapeutic treatment with CYM5520 enhanced the number of osteoblasts, osteoid surface and vertebral bone mass in osteopenic ovariectomized mice by inducing the formation of new bones. Luo et al. (51) investigated the anti-osteoporotic effect of the traditional Chinese medicine FufangZhenzhuTiaozhi (FTZ) in an osteoporosis mouse model and found that FTZ reduced the risk of osteoporosis. Ishii et al. further suggested that the functional inhibition of S1PR2 by the antagonist JTE013 restricted the localization of osteoclast precursors and reduced the number of mature osteoclasts in an osteoporosis mouse model (47). Huang et al. investigated the effect of fingolimod (FTY720, a structural analogue of sphingosine) on the formation of bones in ovariectomized (OVX) rats and showed that FTY720 upregulated the expression levels of various transcription factors in OVX rats and prevented bone resorption in an animal model of post menopausal osteoporosis (52). Yu et al. reported that treatment with FTY720 inhibited osteoporosis in murine bone marrow cells by inhibiting proinflammatory cytokine production and suppressing osteoclastogenesis. FTY720 has been reported to prevent bone loss in animal models by inhibiting RANKL-induced osteoclastogenesis (24).
Bisphosphonates can be used for to treat osteoporosis by inhibiting osteoclast bone resorption and reducing fracture risk (44). Peltier et al. used the MG-63 cell line (osteoblast-like characteristics) to examine the mechanism by which iron impaired osteoblasts. The researchers found that excess iron could cause osteoporosis through the S1P pathway (53). In vitro and in vivo studies indicated that pharmacological intervention could direct in the development of novel therapeutic strategies. These studies suggested that S1PRs could be potential drug targets in the development of osteoporosis treatments (5,53,54). Zhang et al. (23) summarized the role of S1PRs in different bone diseases and how these receptors could be used as potential targets for the treatment of bone diseases, including osteoporosis, based on the biological functions of S1P in osteoclasts/osteoblasts, as well as immune cells and cancer cells.
In addition to inhibiting osteoclast-mediated bone resorption, anabolic targets in osteoblasts or their precursors have also been studied. Strontium ranelate and teriparatide exert anabolic effects through G protein-coupled receptor signalling (46). Calcitonin (CT) hormone acts as an inhibitor of bone resorption. Bone formation is also increased by blocking the murine CT receptor (CTR) (55,56).
Future research
Osteoporosis has become a major health burden with high morbidity and mortality rates. Dysregulation of S1P correlates with the progression of osteoporosis (30,57). In osteoporosis, increased levels of circulating S1P are responsible for increased bone resorption and reduced BMD values. The measurement of bone formation and bone resorption is important for clinical investigations of osteoporotic patients (29,58). Among the available biochemical markers, S1P can be used as an early marker in the diagnosis of osteoporosis. S1P-targeted therapy is an emerging and novel treatment strategy for osteoporosis that lacks serious side effects (59-61). Moreover, specific agonists and antagonists of S1P and the generation of targeted knockout models may provide exciting future avenues to reduce the risk of osteoporosis by limiting inflammation and preventing bone resorption (50,62,63). Another important point is that S1P plays a critical role in obesity and morbidity associated with age, sex and environmental factors (64), which is also an interesting area to explore. S1P might be a link between obesity and osteoporosis in elderly individuals.
Conclusions
Osteoporosis has become a major health problem worldwide; however, treatment strategies to induce osteogenesis in the context of osteoporosis are very limited. S1P has emerged as an important mediator of osteoporosis, and it may serve as a potential diagnostic marker for osteoporosis. S1P might also be regarded as an effective therapeutic target in osteoporosis. It is essential to know how the imbalance between bone formation and bone resorption contributes to osteoporosis. Improved understanding of the functional roles of S1P in bone turnover may lead to the discovery of novel targets for the treatment of osteoporosis. Moreover, S1P-targeting drugs might be a promising therapeutic option for osteoporosis treatment.
Acknowledgments
Funding: This review was supported by National Key R&D Program of China (2019YFA0111900), National Natural Science Foundation of China (No. 81874030,82072506), Provincial Natural Science Foundation of Hunan (No.2018JJ2636,2020JJ3060), Provincial Clinical Medical Technology Innovation Project of Hunan (No.2020SK53709), the Administration of Traditional Chinese Medicine of Hunan Province (No.2021075), Innovation-Driven Project of Central South university (No.2020CX045), Wu Jieping Medical Foundation (No.320.6750.2020-03-14), CMA·Young and Middle-aged Doctors Outstanding Development Program--Osteoporosis Specialized Scientific Research Fund Project (No.G-X-2019-1107-12), the Clinical and Rehabilitation Research Foundation of Xiangya Hospital and Weiming of Peking University (No.xywm2015II04), the Key Research and Development Program of Hunan Province (No.2018SK2076) and the Key program of Health Commission of Hunan Province (No.20201902).
Footnote
Reporting Checklist: The authors have completed the Narrative Review reporting checklist. Available at http://dx.doi.org/10.21037/apm-20-1255
Peer Review File: Available at http://dx.doi.org/10.21037/apm-20-1255.
Conflicts of Interest: All authors have completed the ICMJE uniform disclosure form (available at http://dx.doi.org/10.21037/apm-20-1255). The authors have no conflicts of interest to declare.
Ethical Statement: The authors are accountable for all aspects of the work and ensuring that questions related to the accuracy or integrity of any part of the work are appropriately investigated and resolved.
Open Access Statement: This is an Open Access article distributed in accordance with the Creative Commons Attribution-NonCommercial-NoDerivs 4.0 International License (CC BY-NC-ND 4.0), which permits the non-commercial replication and distribution of the article with the strict proviso that no changes or edits are made and the original work is properly cited (including links to both the formal publication through the relevant DOI and the license). See: https://creativecommons.org/licenses/by-nc-nd/4.0/.
References
- Chun J, Hla T, Lynch KR, et al. International Union of Basic and Clinical Pharmacology. LXXVIII. Lysophospholipid receptor nomenclature. Pharmacol Rev 2010;62:579-87. [Crossref] [PubMed]
- Maceyka M, Harikumar KB, Milstien S, Spiegel S. Sphingosine-1-phosphate signaling and its role in disease. Trends Cell Biol 2012;22:50-60. [Crossref] [PubMed]
- Grassi S, Mauri L, Prioni S, et al. Sphingosine 1-Phosphate Receptors and Metabolic Enzymes as Druggable Targets for Brain Diseases. Front Pharmacol 2019;10:807. [Crossref] [PubMed]
- Xia P, Wadham C. Sphingosine 1-phosphate, a key mediator of the cytokine network: juxtacrine signaling. Cytokine Growth Factor Rev 2011;22:45-53. [Crossref] [PubMed]
- Meshcheryakova A, Mechtcheriakova D, Pietschmann P. Sphingosine 1-phosphate signaling in bone remodeling: multifaceted roles and therapeutic potential. Expert Opin Ther Targets 2017;21:725-37. [Crossref] [PubMed]
- Czubowicz K, Jęśko H, Wencel P, et al. The Role of Ceramide and Sphingosine-1-Phosphate in Alzheimer's Disease and Other Neurodegenerative Disorders. Mol Neurobiol 2019;56:5436-55. [Crossref] [PubMed]
- Blaho VA, Hla T. Regulation of mammalian physiology, development, and disease by the sphingosine 1-phosphate and lysophosphatidic acid receptors. Chem Rev 2011;111:6299-320. [Crossref] [PubMed]
- Saba JD, Hla T. Point-counterpoint of sphingosine 1-phosphate metabolism. Circ Res 2004;94:724-34. [Crossref] [PubMed]
- Rosen H, Goetzl EJ. Sphingosine 1-phosphate and its receptors: an autocrine and paracrine network. Nat Rev Immunol 2005;5:560-70. [Crossref] [PubMed]
- Hsu LC, Reddy SV, Yilmaz Ö, et al. Sphingosine-1-Phosphate Receptor 2 Controls Podosome Components Induced by RANKL Affecting Osteoclastogenesis and Bone Resorption. Cells 2019;8:17. [Crossref] [PubMed]
- Ryu J, Kim HJ, Chang EJ, et al. Sphingosine 1-phosphate as a regulator of osteoclast differentiation and osteoclast-osteoblast coupling. EMBO J 2006;25:5840-51. [Crossref] [PubMed]
- Spiegel S, Milstien S. The outs and the ins of sphingosine-1-phosphate in immunity. Nat Rev Immunol 2011;11:403-15. [Crossref] [PubMed]
- Sims NA, Martin TJ. Coupling the activities of bone formation and resorption: a multitude of signals within the basic multicellular unit. Bonekey Rep 2014;3:481. [Crossref] [PubMed]
- Hla T, Lee MJ, Ancellin N, et al. Lysophospholipids—receptor revelations. Science 2001;294:1875-8. [Crossref] [PubMed]
- Lee SH, Lee SY, Lee YS, et al. Higher circulating sphingosine 1-phosphate levels are associated with lower bone mineral density and higher bone resorption marker in humans. J Clin Endocrinol Metab 2012;97:E1421-8. [Crossref] [PubMed]
- O'Sullivan S, Dev KK. Sphingosine-1-phosphate receptor therapies: Advances in clinical trials for CNS-related diseases. Neuropharmacology 2017;113:597-607. [Crossref] [PubMed]
- Xiao L, Zhou Y, Friis T, et al. S1P-S1PR1 Signaling: the "Sphinx" in Osteoimmunology. Front Immunol 2019;10:1409. [Crossref] [PubMed]
- Spiegel S, Milstien S. Sphingosine-1-phosphate: an enigmatic signalling lipid. Nat Rev Mol Cell Biol 2003;4:397-407. [Crossref] [PubMed]
- El Jamal A, Bougault C, Mebarek S, et al. The role of sphingosine 1-phosphate metabolism in bone and joint pathologies and ectopic calcification. Bone 2020;130:115087 [Crossref] [PubMed]
- Muruganandan S, Sinal CJ. The impact of bone marrow adipocytes on osteoblast and osteoclast differentiation. IUBMB Life 2014;66:147-55. [Crossref] [PubMed]
- During A, Penel G, Hardouin P. Understanding the local actions of lipids in bone physiology. Prog Lipid Res 2015;59:126-46. [Crossref] [PubMed]
- Ishii M, Kikuta J. Sphingosine-1-phosphate signaling controlling osteoclasts and bone homeostasis. Biochim Biophys Acta 2013;1831:223-7. [Crossref] [PubMed]
- Zhang L, Dong Y, Wang Y, et al. Sphingosine-1-phosphate (S1P) receptors: Promising drug targets for treating bone-related diseases. J Cell Mol Med 2020;24:4389-401. [Crossref] [PubMed]
- Yu H, Herbert BA, Valerio M, et al. FTY720 inhibited proinflammatory cytokine release and osteoclastogenesis induced by Aggregatibacter actinomycetemcomitans. Lipids Health Dis 2015;14:66. [Crossref] [PubMed]
- Ishii M, Egen JG, Klauschen F, et al. Sphingosine-1-phosphate mobilizes osteoclast precursors and regulates bone homeostasis. Nature 2009;458:524-8. [Crossref] [PubMed]
- Purdue PE, Crotti TN, Shen Z, et al. Comprehensive profiling analysis of actively resorbing osteoclasts identifies critical signaling pathways regulated by bone substrate. Sci Rep 2014;4:7595. [Crossref] [PubMed]
- Manolagas SC. Birth and death of bone cells: basic regulatory mechanisms and implications for the pathogenesis and treatment of osteoporosis. Endocr Rev 2000;21:115-37. [PubMed]
- Riggs BL, Parfitt AM. Drugs used to treat osteoporosis: the critical need for a uniform nomenclature based on their action on bone remodeling. J Bone Miner Res 2005;20:177-84. [Crossref] [PubMed]
- Garnero P, Delmas PD. Contribution of bone mineral density and bone turnover markers to the estimation of risk of osteoporotic fracture in postmenopausal women. J Musculoskelet Neuronal Interact 2004;4:50-63. [PubMed]
- Sattui SE, Saag KG. Fracture mortality: associations with epidemiology and osteoporosis treatment. Nat Rev Endocrinol 2014;10:592-602. [Crossref] [PubMed]
- Ardawi MS, Rouzi AA, Al-Sibiani SA, et al. High serum sclerostin predicts the occurrence of osteoporotic fractures in postmenopausal women: the Center of Excellence for Osteoporosis Research Study. J Bone Miner Res 2012;27:2592-602. [Crossref] [PubMed]
- Rouzi AA, Al-Sibiani SA, Al-Senani NS, et al. Independent predictors of all osteoporosis-related fractures among healthy Saudi postmenopausal women: the CEOR Study. Bone 2012;50:713-22. [Crossref] [PubMed]
- Raisz LG. Pathogenesis of osteoporosis: concepts, conflicts, and prospects. J Clin Invest 2005;115:3318-25. [Crossref] [PubMed]
- Armas LA, Recker RR. Pathophysiology of osteoporosis: new mechanistic insights. Endocrinol Metab Clin North Am 2012;41:475-86. [Crossref] [PubMed]
- Sipos W, Pietschmann P, Rauner M, et al. Pathophysiology of osteoporosis. Wien Med Wochenschr 2009;159:230-4. [Crossref] [PubMed]
- Pietschmann P, Rauner M, Sipos W, et al. Osteoporosis: an age-related and gender-specific disease--a mini-review. Gerontology 2009;55:3-12. [Crossref] [PubMed]
- Kanis JA, Cooper C, Rizzoli R, et al. European guidance for the diagnosis and management of osteoporosis in postmenopausal women. Osteoporos Int 2019;30:3-44. [Crossref] [PubMed]
- Hernlund E, Svedbom A, Ivergård M, et al. Osteoporosis in the European Union: medical management, epidemiology and economic burden. A report prepared in collaboration with the International Osteoporosis Foundation (IOF) and the European Federation of Pharmaceutical Industry Associations (EFPIA). Arch Osteoporos 2013;8:136. [Crossref] [PubMed]
- Chopin F, Biver E, Funck-Brentano T, et al. Prognostic interest of bone turnover markers in the management of postmenopausal osteoporosis. Joint Bone Spine 2012;79:26-31. [Crossref] [PubMed]
- Dobrosak C, Gooi JH. Increased sphingosine-1-phosphate production in response to osteocyte mechanotransduction. Bone Rep 2017;7:114-20. [Crossref] [PubMed]
- Bae SJ, Lee SH, Ahn SH, et al. The circulating sphingosine-1-phosphate level predicts incident fracture in postmenopausal women: a 3.5-year follow-up observation study. Osteoporos Int 2016;27:2533-41. [Crossref] [PubMed]
- Ardawi MM, Rouzi AA, Al-Senani NS, et al. High Plasma Sphingosine 1-phosphate Levels Predict Osteoporotic Fractures in Postmenopausal Women: The Center of Excellence for Osteoporosis Research Study. J Bone Metab 2018;25:87-98. [Crossref] [PubMed]
- Ota K, Quint P, Ruan M, et al. TGF-β induces Wnt10b in osteoclasts from female mice to enhance coupling to osteoblasts. Endocrinology 2013;154:3745-52. [Crossref] [PubMed]
- Walia B, Lingenheld E, Duong L, et al. A novel role for cathepsin K in periosteal osteoclast precursors during fracture repair. Ann N Y Acad Sci 2018;1415:57-68. [Crossref] [PubMed]
- Kikuta J, Kawamura S, Okiji F, et al. Sphingosine-1-phosphate-mediated osteoclast precursor monocyte migration is a critical point of control in antibone-resorptive action of active vitamin D. Proc Natl Acad Sci U S A 2013;110:7009-13. [Crossref] [PubMed]
- Diepenhorst N, Rueda P, Cook AE, et al. G protein-coupled receptors as anabolic drug targets in osteoporosis. Pharmacol Ther 2018;184:1-12. [Crossref] [PubMed]
- Ishii M, Kikuta J, Shimazu Y, et al. Chemorepulsion by blood S1P regulates osteoclast precursor mobilization and bone remodeling in vivo. J Exp Med 2010;207:2793-8. [Crossref] [PubMed]
- Weske S, Vaidya M, von Wnuck Lipinski K, et al. Agonist-induced activation of the S1P receptor 2 constitutes a novel osteoanabolic therapy for the treatment of osteoporosis in mice. Bone 2019;125:1-7. [Crossref] [PubMed]
- Heckt T, Brylka LJ, Neven M, et al. Deficiency of sphingosine-1-phosphate receptor 3 does not affect the skeletal phenotype of mice lacking sphingosine-1-phosphate lyase. PLoS One 2019;14:e0219734 [Crossref] [PubMed]
- Weske S, Vaidya M, Reese A, et al. Targeting sphingosine-1-phosphate lyase as an anabolic therapy for bone loss. Nat Med 2018;24:667-78. [Crossref] [PubMed]
- Luo D, Li J, Chen K, et al. Untargeted Metabolomics Reveals the Protective Effect of FufangZhenshuTiaozhi (FTZ) on Aging-Induced Osteoporosis in Mice. Front Pharmacol 2019;9:1483. [Crossref] [PubMed]
- Huang C, Ling R, Li FJ, et al. FTY720 enhances osteogenic differentiation of bone marrow mesenchymal stem cells in ovariectomized rats. Mol Med Rep 2016;14:927-35. [Crossref] [PubMed]
- Peltier L, Bendavid C, Cavey T, et al. Iron excess upregulates SPNS2 mRNA levels but reduces sphingosine-1-phosphate export in human osteoblastic MG-63 cells. Osteoporos Int 2018;29:1905-15. [Crossref] [PubMed]
- Sefcik LS, Aronin CE, Awojoodu AO, et al. Selective activation of sphingosine 1-phosphate receptors 1 and 3 promotes local microvascular network growth. Tissue Eng Part A 2011;17:617-29. [Crossref] [PubMed]
- Hoff AO, Catala-Lehnen P, Thomas PM, et al. Increased bone mass is an unexpected phenotype associated with deletion of the calcitonin gene. J Clin Invest 2002;110:1849-57. [Crossref] [PubMed]
- Keller J, Catala-Lehnen P, Huebner AK, et al. Calcitonin controls bone formation by inhibiting the release of sphingosine 1-phosphate from osteoclasts. Nat Commun 2014;5:5215. [Crossref] [PubMed]
- Kanis JA, Odén A, McCloskey EV, et al. A systematic review of hip fracture incidence and probability of fracture worldwide. Osteoporos Int 2012;23:2239-56. [Crossref] [PubMed]
- Garnero P. The Utility of Biomarkers in Osteoporosis Management. Mol Diagn Ther 2017;21:401-18. [Crossref] [PubMed]
- Garnero P. New developments in biological markers of bone metabolism in osteoporosis. Bone 2014;66:46-55. [Crossref] [PubMed]
- Chapurlat RD, Confavreux CB. Novel biological markers of bone: from bone metabolism to bone physiology. Rheumatology (Oxford) 2016;55:1714-25. [Crossref] [PubMed]
- Kim BJ, Koh JM. Coupling factors involved in preserving bone balance. Cell Mol Life Sci 2019;76:1243-53. [Crossref] [PubMed]
- Kikuta J, Kawamura S, Ishii M. Encounter of cancer cells with bone. In vivo imaging of osteoclasts and their precursors in intact bone tissues. Clin Calcium 2011;21:372-8. [PubMed]
- Bigaud M, Guerini D, Billich A, et al. Second generation S1P pathway modulators: research strategies and clinical developments. Biochim Biophys Acta 2014;1841:745-58. [Crossref] [PubMed]
- Torretta E, Barbacini P, Al-Daghri NM, et al. Sphingolipids in Obesity and Correlated Co-Morbidities: The Contribution of Gender, Age and Environment. Int J Mol Sci 2019;20:5901. [Crossref] [PubMed]