Considerations and perspectives on digestive diseases during the COVID-19 pandemic: a narrative review
Introduction
Since its initial outbreak in December 2019, coronavirus disease 2019 (COVID-19) has become a pandemic, with a rate of infection surpassing that of severe acute respiratory syndrome (SARS) (1). The growing numbers of confirmed cases and suspected cases, which are associated with complex clinical manifestations, have posed significant challenges for disease diagnosis. Due to atypical symptoms, differential diagnosis of COVID-19 is challenging. If suspected patients have been admitted to the digestive ward instead of the quarantine ward, widespread viral transmission may occur, which might expose the medical staff and other patients to this deadly disease given the high infectivity of severe acute respiratory syndrome coronavirus 2 (SARS-CoV-2). Therefore, it is critical to pay close attention to patients with gastrointestinal symptoms, especially those with fever. To date, there has yet to be a systematic review of COVID-19-related digestive symptoms and underlying mechanisms. To address this gap in the literature, this review summarizes the digestive system symptoms of patients with COVID-19. The review is highly relevant in today’s scenario given the current global pandemic and has implications for how clinical professionals treat COVID-19 patients. We searched PubMed using the search terms “COVID-19,” “digestive diseases,” “ACE2,” “intestinal microecology,” and “gut-lung axis” for studies published from January 1, 2004, to July 1, 2020. Further, we manually searched the references of selected articles for additional relevant articles. We selected articles that were relevant to a general medicine readership.
We present the following article in accordance with the Narrative Review reporting checklist (available at http://dx.doi.org/10.21037/apm-20-2124).
COVID-19 pandemic
COVID-19 is caused by SARS-CoV-2 infection and is characterized by fatigue, fever, muscle pain, and dry cough. These main clinical symptoms are often accompanied by digestive system-related symptoms such as diarrhoea (2). The spread of the COVID-19 pandemic has caused major challenges in clinical gastroenterology. Laboratory tests of patients with COVID-19 indicate reduced lymphocyte counts, and severe cases can rapidly progress to acute respiratory distress syndrome, septic shock, multiple-organ failure, and even death (3). The sources of infection predominantly comprise individuals clearly infected with SARS-CoV-2, although asymptomatic patients may also be a source of infection via respiratory droplets and contact transmission (4). The majority of the population are susceptible to COVID-19, which is a highly infectious disease. Indeed, there is clear evidence that it has affected medical workers and spread in specific communities (5).
Understanding coronaviruses
Coronaviruses are single-stranded RNA viruses that can be divided into four genera (α, β, γ, and δ) based on serotype and genomic characteristics. COVID-19 is caused by a novel coronavirus of the β genus. The average incubation period of SARS-CoV-2 is 1–14 days but typically ranges from 3–7 days, although longer incubation periods of 24 days or more have been reported (6). Although other regions of China have also been affected, Hubei is the most highly affected province. After the outbreak, China actively responded to contain the epidemic, on January 12, 2020, the National Center for Disease Control and Prevention added COVID-2019 to the Class B infectious diseases stipulated in the laws of the People’s Republic of China on the Prevention and Control of Infectious Diseases and adopted measures of prevention and control management of Class A infectious diseases.
Reconsidering diseases of the digestive system during the COVID-19 pandemic
Gastrointestinal symptoms such as nausea, vomiting, abdominal pain, or diarrhoea are frequently observed in patients with COVID-19 (7). One reason for early delays in diagnosis and inappropriate treatment of infected patients is that gastrointestinal symptoms may precede the respiratory presentation of SARS-COV-2 infection (8). Recent, detection of SARS-CoV-2 in stools suggested the possibility of fecal-oral transmission, which was subsequently confirmed by reports that SARS-CoV-2 can multiply in both the respiratory and digestive tracts (9). In addition, COVID-19 patients with gastrointestinal symptoms experience more severe respiratory disorders than those without gastrointestinal symptoms (10).
Angiotensin converting enzyme-2 (ACE2) hypothesis
ACE2 is a functional receptor of SARS-COV-2 (11). ACE2 is highly expressed on the brush-like edge and deep within the intestinal wall, especially in smooth muscle cells of the intestinal muscular layer, vascular smooth muscle cells, and endothelial cells. Studies of ACE2 expression in lung and bronchial branch cells have indicated that higher ACE2 expression is associated with greater ease of SARS-CoV-2 entry into cells (12). These findings have led to a hypothesis about the role of ACE2 in viral transmission. As a functional receptor of SARS-COV-2, ACE2 plays a crucial role in the pathogenesis of COVID-19 and promotes virus entry into human cells (13). SARS-CoV-2 initially enters the respiratory tract via the oral and nasopharyngeal epithelial mucosa or directly into the lower respiratory tract, subsequently infecting bronchial and alveolar epithelial cells. At this initial stage, the virus may enter the surrounding blood circulation through the lungs and cause sepsis. Patients at increased risk of developing severe disease may experience severe lung involvement, leading to systemic inflammation. Large-scale inflammatory processes may cause severe cytokine storms and affect other organs of the body (14-16). This presentation is consistent with other blood-related sources of viral entry into organs via activated ACE2 on endothelial cells, resulting in cardiovascular, kidney, or gastrointestinal symptoms. SARS-CoV-2 can directly invade the gastrointestinal epithelium via ACE2. A single-cell transcriptome study revealed that ACE2 was highly expressed in alveolar epithelial cells, esophageal epithelium, and absorptive intestinal epithelium of the ileum and colon, and co-expressed with Transmembrane Protease Serine 2 (TMPRSS2) protein (17-19).
Notably, SARS-COV-2 recognizes human ACE2 more effectively than SARS-COV, thus increasing the person-to-person transmission capacity of SARS-COV-2 (20). The SARS-COV-2 spike protein has been speculated to have a strong binding affinity for human ACE2. This leads to a 10-20-fold increase in the binding affinity between SARS-COV-2 and ACE2 by and, consequently, its pathogenicity, especially in the small intestine, which may be vulnerable to SARS-COV-2 infection (17). These findings underscore the possibility that SARS-CoV-2 may also cause gastrointestinal infection. The virus may target different levels of the small intestine, including the stomach, resulting in upper gastrointestinal symptoms. In addition, viral RNA has been detected in stool samples and negative oral swab, leading to the hypothesis that viral replication and activity occur in the intestine and that the virus may be more prevalent in this region (21). The gastrointestinal manifestations of SARS-CoV-2 infection indicate the possibility of viral fecal-oral transmission (22). Consistent with the possible transmission route of SARS-CoV-2, the respiratory and gastrointestinal systems share an interface with the external environment. The ACE2 receptor is widely distributed in the human body and is abundantly expressed in almost every organ of the gastrointestinal tract. In this regard, organs comprising ACE2-expressing cells may act as potential infection sites and transmission routes of SARS-COV-2 (Figure 1). Therefore, these organs provide potential targets for combating SARS-CoV-2 infection.
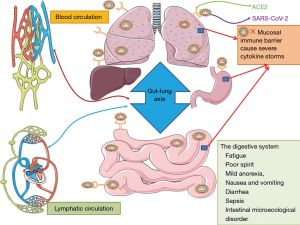
There is compelling evidence that ACE2 receptors provide entry points for the virus. However, high expression of ACE2 may pose benefits. For instance, circulating ACE2 may bind SARS-CoV-2 and remove it from the circulation. Additionally, increased ACE2 expression may promote self-dimerization in the cell membrane, reducing viral affinity, internalization, and propagation. Finally, increasing ACE2 levels may shift the Renin-Angiotensin System (RAS) towards vasoprotective functions (23). These findings collectively highlight the potential of targeting ACE2 as a treatment for COVID-19, and future research in this area is warranted.
Intestinal microecology in patients with COVID-19
The human gastrointestinal tract hosts over 1,014 cells comprising 500 to 1,000 bacterial species, which are referred to as the gut microbiota (24). A well-balanced bidirectional interaction exists between gut microbiota and the immune system. For instance, microbiota plays a fundamental role in the development and maturation of the immune system; conversely, the immune system shapes microbiota composition and functions. Notably, disruption of this balance may lead to human diseases (25).
Patients with COVID-19 may present with isolated symptoms of the digestive system, such as mild anorexia, fatigue, nausea, vomiting, and diarrhea, as initial manifestations in the absence of respiratory symptoms (26). It is believed that a dynamic balance exists between microorganisms entering and exiting the lungs. Lung lesions are associated with changes in the types and numbers of bacteria, and the proliferation rate of these microorganisms substantially exceeds the ability of the respiratory tract to remove pathogenic microorganisms. In this process, pulmonary flora and pathogenic microorganisms may not be the sole microorganisms infecting the lungs. Indeed, intestinal flora may also modulate pulmonary diseases (27,28).
Intestinal flora is involved in various physiological processes, including metabolism and nutrient synthesis (29). They degrade complex polysaccharides such as cellulose and hemicellulose, thus providing energy for the body. Further, intestinal flora synthesize certain essential nutrients, such as short-chain fatty acids and vitamin K. Intestinal flora also participate in the metabolism of exogenous substance, thereby regulating their absorption and utilization (30). Furthermore, intestinal flora are involved in the regulation of both innate and acquired immunity. For instance, the mucosal immunity of sterile mice is rapidly restored using microbial flora in fecal supernatants (31). Moreover, intestinal flora co-participates in resistance to pathogens and microbial infections (32). Upon invasion by pathogenic microorganisms, gut microbes “dilute” the pathogens and protect the body by competing with them. In addition, intestinal flora protects the body by producing antibacterial compounds. Intestinal flora are also strongly implicated in lung health (33). The rapid developments in genome sequencing technology have facilitated the elucidation of intestinal microecological differences between diseases and healthy conditions, which may reveal the pathophysiological mechanisms of digestive tract symptoms in patients with COVID-19, mechanisms underscoring disease occurrence and development, and potential novel treatment approaches (34). In the early stages of infection, limited numbers of microorganisms colonize the lungs, predominantly by migrating from pharyngeal secretions or gastric juices to the lungs via microaspiration. These microorganisms are removed via phagocytosis by alveolar macrophages and transport of mucosal cilia, thus ensuring balance and stability of the pulmonary microecology (35,36). Pulmonary microorganisms thereby promote the maturation of the immune system.
Respiratory influenza infection causes intestinal injury, which is not due to direct intestinal viral infection but is induced by alterations in intestinal microbiota composition mediated by lung-derived CCR9+CD4+ T cells (37). Additionally, respiratory diseases following viral lung infection alter the murine gut microbiota, and similar mechanisms may underpin the gastrointestinal symptoms associated with COVID-19 (38). Furthermore, acute respiratory disease syndrome (ARDS) is characterized as a partial cause of death in patients with COVID-19. In this regard, the lung microbiome of patients with ARDS is enriched with gut bacteria. The interaction between the lungs and intestine during inflammatory responses may induce a vicious cycle of pulmonary and intestinal inflammation (39,40). Therefore, intestinal symptoms may be a predictor of COVID-19 and reflect dyshomeostasis of the intestinal microbiota and immune system. It is thus recommended that the lungs and gut be treated as a whole in the diagnosis and treatment of COVID-19.
A meta-analysis of 4,243 patients reported that the pooled prevalence of gastrointestinal symptoms was 17.6% (41). Given that the intestinal tract absorbs approximately 95% of nutrients, excretes approximately 80% of toxins, and regulates approximately 70% of immunity, it is clear that the intestinal tract is a critical organ involved in digestion, metabolism, and immunity (42). Indeed, the gastrointestinal system hosts the largest microecological niche in the human body, and its health thus determines the health of the entire organism (43). The pathogenesis of SARS-CoV-2 infection strongly resembles that of immune-mediated diseases, which has led to the hypothesis that targeted therapies used for the treatment of immune-mediated diseases may be effective for the treatment or prevention of COVID-19-related complications. The National Health Commission of China proposed the involvement of intestinal microecological regulators in the diagnosis and treatment of COVID-19 (44). This is because critically ill patients are in an acute stress state and are prone to stress ulcers. The extensive use of multiple antibiotics increases the risk of intestinal flora disorders and can cause damage to the body’s largest immune organ, the intestinal mucosal immune barrier, which can result in secondary bacterial infections (45). The effect of intestinal flora on mucosal immunity are not limited to the gastrointestinal tract; indeed, they also affect the immune response of the distal mucosa outside the intestinal tract, including the lungs, thus aggravating disease symptoms. Therefore, microecological therapy should be actively applied in the treatment of COVID-19 in order to maintain intestinal microecological balance and prevent secondary bacterial infections.
The “gut-lung” axis theory in COVID-19
The ensemble of relevant interactions between the intestine and lungs, including gut and lung microbiota, their intercompartmental crosstalk, and the interplay of gut and lung immune systems with local or distant interactions, has been termed the “gut-lung” axis (46). Growing evidence indicates the occurrence of crosstalk between gut microbiota and the lungs, which maintains host homeostasis and disease development via interactions with the immune system (47). These gut-lung interactions may influence COVID-19 severity in patients with extrapulmonary conditions. The pulmonary tropism of SARS-CoV-2 and attending respiratory pathophysiology and inflammation may be intimately associated with the gut tropism of SARS-CoV-2 and gastrointestinal events of COVID-19.
The alveolar, glandular, and mucosal epithelium of the lungs, trachea, and large intestine develop from the endoderm of the primary intestine and are embryologically homologous. The mucosal structure of the respiratory and gastrointestinal tracts is critical for survival of the microbial community and acts as an important barrier to protect the body against pathogen invasion via the mucosal immune system. The physiological conditions of the mucosal surface, such as temperature, humidity, pH, and secretions, affect microbial growth and migration (48). Secretory IgA, an immunoglobulin secreted by the mucosa, also exerts selective effects on microorganisms on the mucosal surface. Innate immune cells or epithelial cells recognise the existence of microorganisms and release antimicrobial peptides and inflammatory factors, which activate lymphocytes and induce an immune response (49). For example, more than 50% of patients with inflammatory bowel disease (IBD) experience respiratory dysfunction following disease onset (50). Research has indicated that dynamic crosstalk occurs between microbes of the “gut-lung” axis. Interactions of the gut and lung niche are mediated via this axis, which provides a route for the passage of hormones, microbial metabolites, cytokines, and endotoxins into the bloodstream. A balanced gut community is of vital importance for pulmonary immunity. The “gut-lung” axis is assumed to be bidirectional, suggesting that infection with SARS-CoV-2 in the lungs may trigger an immune response in the gastrointestinal tract. Infection of the lungs with SARS-CoV-2 causes epithelial disruption in gas exchange areas and associated airways (51). Patients with COVID-19-induced infectious pneumonia often present with diarrhea, vomiting, and other gastrointestinal symptoms. Thus, it is evident that the balance and stability of the lung and intestinal microecology are bidirectionally regulated.
Microbiota that colonizes the respiratory and gastrointestinal mucosa interact to regulate local tissues, thereby acting as a substrate connecting the lungs and intestines (52). Developments in microbiome research in recent years have led to the concept of the “gut-lung” axis, which refers to the common regulation of immunity and inflammation between the gastrointestinal tract and lungs (53). The stability of intestinal microecology, which is underscored by the health and integrity of intestinal flora, plays an important role in maintaining lung health.
Clinically, intestinal flora participates in the regulation of various pulmonary diseases including viral pneumonia, asthma, tuberculosis, and chronic obstructive pulmonary disease via the “gut-lung” axis (53). This shows that intestinal flora influences the occurrence and development of pulmonary diseases. Conversely, perturbations in intestinal flora caused by pulmonary diseases, especially viral invasion, and infectious diseases, may affect the digestive system via immune regulation (54).
An increase in dietary fibre intake may lead to similar changes in the microbiome of the lungs and intestines, and intestinal microorganisms may be transferred to the lungs (55). For example, destruction of the intestinal mucosal integrity and displacement of intestinal flora into the blood and lungs may result in sepsis and ARDS (56). Microbes in the lungs and intestine influence each other by modulating the immune system. In recent years, specific lung and intestinal flora have been demonstrated to affect the immune system, and beneficial flora have been successfully harnessed to treat acute or chronic lung diseases (57). Therefore, it can be inferred that the immunomodulatory signals produced by various microbes in the lungs and intestines play an important role in maintaining health, and further research on this topic is warranted. Further, immune cells travel via blood circulating in the lung and intestine, thereby providing another substrate for the “gut-lung” axis. Perturbations in intestinal flora result in increased production of inflammatory mediators. Excessive inflammatory mediators enter the lungs through the blood circulation, thereby affecting the microecological environment of the lungs and the types and intensity of immune responses (58-60).
“Gut-lung” communication is thought to be mediated by several substrates. Short-chain fatty acids such as butyric acid, acetic acid, and propionic acid, which are produced by the fermentation of starch by intestinal microorganisms, enter the lung tissues through blood circulation (61). Unmetabolized short-chain fatty acids enter the peripheral blood circulatory system and bone marrow to affect immune cell development. Further, bone marrow-derived immune cells trigger an immune response in distal body parts such as lung tissue (31). Intestinal immune cells also move directly from the intestinal tract to the respiratory tract via the blood circulation to regulate immune activity of the respiratory system (62).
Liver injury in COVID-19
Novel coronaviruses can invade the liver, resulting in abnormal hepatic function (63). COVID-19-associated abnormalities in liver biochemistry underpin the occurrence, development, and treatment of liver-related symptoms in patients with COVID-19. Pathological findings in liver biopsy specimens of patients with COVID-19 include moderate microvascular fatty degeneration and active inflammation in hepatic lobule portal regions, suggesting that the pathology may be caused by novel coronavirus infection (64). COVID-19 patients with digestive illness symptoms more commonly present with hepatic injury than those without the symptoms (65). It is speculated that patients with COVID-19 are affected by liver pathogenesis in several ways, for instance, direct toxic effects of coronavirus may mediate these symptoms. Histopathologic examinations of liver biopsies of patients with COVID-19 displayed increased liver volume, hepatocellular degeneration, and necrosis with neutrophil infiltration, liver blood sinus congestion, accumulation of visible lymphocytes, mononuclear cell infiltration, and microthrombosis (66). Further, the bile duct epithelium expresses ACE2 and bile duct cells may undergo compensatory hyperplasia of liver parenchyma cells. The general expression of ACE2 in liver tissue reflects bile duct injury, however, no significant elevations in alkaline phosphatase and glutamine transferase, a marker of bile duct injury, have been reported (67). In addition, novel coronaviruses may invade other tissues and organs, such as the heart, kidney, and muscle, resulting in elevated serum transaminases and other enzymes in the myocardium or skeletal muscle (68). Further, coronavirus infection may activate immune cells, resulting in excessive immune cell aggregation and release of proinflammatory cytokines, such as tumour necrosis factor interleukin (IL)-6, and IL-18, which are associated with systemic inflammatory response syndrome and ARDS (69). These events may induce hypoxia, leading to more cell damage, necrosis, and a vicious cycle resulting in lung injury and damage to the liver, heart, kidney, and other organs (70). The resulting cytokine storm induced by COVID-19 infection is an important cause of liver damage in patients with COVID-19 (71). Moreover, hypoxia can induce oxidative stress response in respiratory distress syndrome and concomitantly augment reactive oxygen species production, resulting in the further release of proinflammatory factors that induce liver damage (72). Hypoxic hepatitis, also known as ischemic hepatitis or “shock liver” is common in severe heart failure, respiratory failure, surgery, trauma, and other causes of hypotensive shock or severe hypoxemia in patients (73). Clinical features include a rapid increase in transaminases, often accompanied by an increase in lactate dehydrogenase (74). Abnormalities in liver biochemistry can be ameliorated by improving circulatory and respiratory functions (73). Therefore, ischemia and hypoxia may be the main mechanisms underscoring liver injury in severe and critically ill patients with COVID-19. Further, drug-induced liver injury in such patients may also contribute to abnormal liver function. Patients with COVID-19, especially severe and critically ill patients, are often treated with multiple medications. Indeed, more than 50% of patients with COVID-19 receive antibiotics intravenously, while 45% of patients receive more than two types of combination therapy with antibiotics, with a drug duration of 3–17 days (75). A study of elderly patients with liver disease, including chronic hepatitis B virus (HBV) and hepatitis C virus infection, alcoholic liver disease, non-alcoholic fatty liver disease (NAFLD), and other basic liver disease, revealed that HBV patients who received antiviral therapy may relapse if they discontinue anti-HBV drugs while infected with COVID-19. Moreover, patients who did not receive anti-HBV therapy may have received high doses of hormone therapy, resulting in the activated (or reactivated) of HBV. Further, patients with NAFLD presented with persistent or fluctuating liver biochemical abnormalities over a protracted period (75).
Conclusions
Our present understanding of COVID-19 remains limited, and the pandemic has become a major challenge for the global health system. There is still a lack of perfect design and laboratory research implementation to support, and further studies are warranted. For medical professionals, deeper understanding of COVID-19 symptoms is critical, and the identification and protection of asymptomatic patients should be improved. Temporary precautionary measures include hand-washing, the use of masks and gloves, and isolation. Patients in the incubation period may be asymptomatic but remain infectious; these patients are a critical source of hidden infections and should be isolated. For asymptomatic cases, routine blood biochemical examinations, respiratory pathogen detection, and chest computed tomography examination are warranted. Diagnosis and treatment programs for COVID-19 should be dynamically adjusted based on concurrent developments in clinical interventions and our understanding of this disease in order to ensure consistency with the clinical situation, improve clinical outcomes, and minimize missed diagnosis or misdiagnoses.
Acknowledgments
Funding: This research was supported by the Science-Technology Foundation for Young Scientists of the Gansu Province of China (grant No. 18JR3RA059).
Footnote
Reporting Checklist: The authors have completed the Narrative Review reporting checklist. Available at http://dx.doi.org/10.21037/apm-20-2124
Conflicts of Interest: All authors have completed the ICMJE uniform disclosure form (available at http://dx.doi.org/10.21037/apm-20-2124). The authors have no conflicts of interest to declare.
Ethical Statement: The authors are accountable for all aspects of the work in ensuring that questions related to the accuracy or integrity of any part of the work are appropriately investigated and resolved.
Open Access Statement: This is an Open Access article distributed in accordance with the Creative Commons Attribution-NonCommercial-NoDerivs 4.0 International License (CC BY-NC-ND 4.0), which permits the non-commercial replication and distribution of the article with the strict proviso that no changes or edits are made and the original work is properly cited (including links to both the formal publication through the relevant DOI and the license). See: https://creativecommons.org/licenses/by-nc-nd/4.0/.
References
- Chu H, Chan JF, Yuen TT, et al. Comparative tropism, replication kinetics, and cell damage profiling of SARS-CoV-2 and SARS-CoV with implications for clinical manifestations, transmissibility, and laboratory studies of COVID-19: an observational study. Lancet Microbe 2020;1:e14-e23. [Crossref] [PubMed]
- Huang C, Wang Y, Li X, et al. Clinical features of patients infected with 2019 novel coronavirus in Wuhan, China. Lancet 2020;395:497-506. [Crossref] [PubMed]
- Lake MA. What we know so far: COVID-19 current clinical knowledge and research. Clin Med (Lond) 2020;20:124-7. [Crossref] [PubMed]
- Franczuk M, Przybylowski T, Czajkowska-Malinowska M, et al. Spirometry during the SARS-CoV-2 pandemic. Guidelines and practical advice from the expert panel of Respiratory Physiopathology Assembly of Polish Respiratory Society. Adv Respir Med 2020;88:640-50. [Crossref] [PubMed]
- Dubey S, Biswas P, Ghosh R, et al. Psychosocial impact of COVID-19. Diabetes Metab Syndr 2020;14:779-88. [Crossref] [PubMed]
- Wang Y, Wang Y, Chen Y, et al. Unique epidemiological and clinical features of the emerging 2019 novel coronavirus pneumonia (COVID-19) implicate special control measures. J Med Virol 2020;92:568-76. [Crossref] [PubMed]
- Suresh Kumar VC, Mukherjee S, Harne PS, et al. Novelty in the gut: a systematic review and meta-analysis of the gastrointestinal manifestations of COVID-19. BMJ Open Gastroenterol 2020;7:e000417 [Crossref] [PubMed]
- Xiao F, Tang M, Zheng X, et al. Evidence for Gastrointestinal Infection of SARS-CoV-2. Gastroenterology 2020;158:1831-3.e3. [Crossref] [PubMed]
- Lamers MM, Beumer J, van der Vaart J, et al. SARS-CoV-2 productively infects human gut enterocytes. Science 2020;369:50-4. [Crossref] [PubMed]
- Olaimat AN, Aolymat I, Al-Holy M, et al. The potential application of probiotics and prebiotics for the prevention and treatment of COVID-19. NPJ Sci Food 2020;4:17. [Crossref] [PubMed]
- Hamming I, Timens W, Bulthuis ML, et al. Tissue distribution of ACE2 protein, the functional receptor for SARS coronavirus. A first step in understanding SARS pathogenesis. J Pathol 2004;203:631-7. [Crossref] [PubMed]
- Lukassen S, Chua RL, Trefzer T, et al. SARS-CoV-2 receptor ACE2 and TMPRSS2 are primarily expressed in bronchial transient secretory cells. EMBO J 2020;39:e105114 [Crossref] [PubMed]
- Ziegler CGK, Allon SJ, Nyquist SK, et al. SARS-CoV-2 Receptor ACE2 Is an Interferon-Stimulated Gene in Human Airway Epithelial Cells and Is Detected in Specific Cell Subsets across Tissues. Cell 2020;181:1016-35.e19. [Crossref] [PubMed]
- Zhou F, Yu T, Du R, et al. Clinical course and risk factors for mortality of adult inpatients with COVID-19 in Wuhan, China: a retrospective cohort study. Lancet 2020;395:1054-62. [Crossref] [PubMed]
- Lin L, Lu L, Cao W, et al. Hypothesis for potential pathogenesis of SARS-CoV-2 infection-a review of immune changes in patients with viral pneumonia. Emerg Microbes Infect 2020;9:727-32. [Crossref] [PubMed]
- Liu J, Li S, Liu J, et al. Longitudinal characteristics of lymphocyte responses and cytokine profiles in the peripheral blood of SARS-CoV-2 infected patients. EBioMedicine 2020;55:102763 [Crossref] [PubMed]
- Hoffmann M, Kleine-Weber H, Schroeder S, et al. SARS-CoV-2 Cell Entry Depends on ACE2 and TMPRSS2 and Is Blocked by a Clinically Proven Protease Inhibitor. Cell 2020;181:271-80.e8. [Crossref] [PubMed]
- Xu H, Zhong L, Deng J, et al. High expression of ACE2 receptor of 2019-nCoV on the epithelial cells of oral mucosa. Int J Oral Sci 2020;12:8. [Crossref] [PubMed]
- Zhang H, Penninger JM, Li Y, et al. Angiotensin-converting enzyme 2 (ACE2) as a SARS-CoV-2 receptor: molecular mechanisms and potential therapeutic target. Intensive Care Med 2020;46:586-90. [Crossref] [PubMed]
- Chen N, Zhou M, Dong X, et al. Epidemiological and clinical characteristics of 99 cases of 2019 novel coronavirus pneumonia in Wuhan, China: a descriptive study. Lancet 2020;395:507-13. [Crossref] [PubMed]
- Kopel J, Perisetti A, Gajendran M, et al. Clinical Insights into the Gastrointestinal Manifestations of COVID-19. Dig Dis Sci 2020;65:1932-9. [Crossref] [PubMed]
- Chen C, Gao G, Xu Y, et al. SARS-CoV-2-Positive Sputum and Feces After Conversion of Pharyngeal Samples in Patients With COVID-19. Ann Intern Med 2020;172:832-4. [Crossref] [PubMed]
- Cole-Jeffrey CT, Liu M, Katovich MJ, et al. ACE2 and Microbiota: Emerging Targets for Cardiopulmonary Disease Therapy. J Cardiovasc Pharmacol 2015;66:540-50. [Crossref] [PubMed]
- Zhang YJ, Li S, Gan RY, et al. Impacts of gut bacteria on human health and diseases. Int J Mol Sci 2015;16:7493-519. [Crossref] [PubMed]
- Ostaff MJ, Stange EF, Wehkamp J. Antimicrobial peptides and gut microbiota in homeostasis and pathology. EMBO Mol Med 2013;5:1465-83. [Crossref] [PubMed]
- Wong SH, Lui RN, Sung JJ. Covid-19 and the digestive system. J Gastroenterol Hepatol 2020;35:744-8. [Crossref] [PubMed]
- De Filippis F, Pellegrini N, Vannini L, et al. High-level adherence to a Mediterranean diet beneficially impacts the gut microbiota and associated metabolome. Gut 2016;65:1812-21. [Crossref] [PubMed]
- Subramanian S, Blanton LV, Frese SA, et al. Cultivating healthy growth and nutrition through the gut microbiota. Cell 2015;161:36-48. [Crossref] [PubMed]
- Mohr AE, Jager R, Carpenter KC, et al. The athletic gut microbiota. J Int Soc Sports Nutr 2020;17:24. [Crossref] [PubMed]
- Rowland I, Gibson G, Heinken A, et al. Gut microbiota functions: metabolism of nutrients and other food components. Eur J Nutr 2018;57:1-24. [Crossref] [PubMed]
- Ekmekciu I, von Klitzing E, Fiebiger U, et al. Immune Responses to Broad-Spectrum Antibiotic Treatment and Fecal Microbiota Transplantation in Mice. Front Immunol 2017;8:397. [Crossref] [PubMed]
- Dong D, Ni Q, Wang C, et al. Effects of intestinal colonization by Clostridium difficile and Staphylococcus aureus on microbiota diversity in healthy individuals in China. BMC Infect Dis 2018;18:207. [Crossref] [PubMed]
- Dumas A, Bernard L, Poquet Y, et al. The role of the lung microbiota and the gut-lung axis in respiratory infectious diseases. Cell Microbiol 2018;20:e12966 [Crossref] [PubMed]
- Galanopoulos M, Gkeros F, Doukatas A, et al. COVID-19 pandemic: Pathophysiology and manifestations from the gastrointestinal tract. World J Gastroenterol 2020;26:4579-88. [Crossref] [PubMed]
- von Mutius E. Intimate Crosstalk in Lower Airways at the Beginning of Life. Cell Host Microbe 2018;24:758-9. [Crossref] [PubMed]
- Gollwitzer ES, Marsland BJ. Impact of Early-Life Exposures on Immune Maturation and Susceptibility to Disease. Trends Immunol 2015;36:684-96. [Crossref] [PubMed]
- Zhang S, Wei T, Tianv H, et al. Small intestinal injury in mice infected with respiratory influenza A virus: evidence for virus induced gastroenteritis. Biotechnol Lett 2015;37:1585-92. [Crossref] [PubMed]
- Groves HT, Cuthbertson L, James P, et al. Respiratory Disease following Viral Lung Infection Alters the Murine Gut Microbiota. Front Immunol 2018;9:182. [Crossref] [PubMed]
- Marsland BJ, Trompette A, Gollwitzer ES. The Gut-Lung Axis in Respiratory Disease. Ann Am Thorac Soc 2015;12:S150-6. [PubMed]
- Fink MP, Delude RL. Epithelial barrier dysfunction: a unifying theme to explain the pathogenesis of multiple organ dysfunction at the cellular level. Crit Care Clin 2005;21:177-96. [Crossref] [PubMed]
- Cheung KS, Hung IFN, Chan PPY, et al. Gastrointestinal Manifestations of SARS-CoV-2 Infection and Virus Load in Fecal Samples From a Hong Kong Cohort: Systematic Review and Meta-analysis. Gastroenterology 2020;159:81-95. [Crossref] [PubMed]
- Thaiss CA, Zmora N, Levy M, et al. The microbiome and innate immunity. Nature 2016;535:65-74. [Crossref] [PubMed]
- Qin Z, Yang X, Chen G, et al. Crosstalks Between Gut Microbiota and Vibrio Cholerae. Front Cell Infect Microbiol 2020;10:582554 [Crossref] [PubMed]
- Xu K, Cai H, Shen Y, et al. Management of corona virus disease-19 (COVID-19): the Zhejiang experience. Zhejiang Da Xue Xue Bao Yi Xue Ban 2020;49:147-57. [PubMed]
- Lange K, Buerger M, Stallmach A, et al. Effects of Antibiotics on Gut Microbiota. Dig Dis 2016;34:260-8. [Crossref] [PubMed]
- Enaud R, Prevel R, Ciarlo E, et al. The Gut-Lung Axis in Health and Respiratory Diseases: A Place for Inter-Organ and Inter-Kingdom Crosstalks. Front Cell Infect Microbiol 2020;10:9. [Crossref] [PubMed]
- Aktas B, Aslim B. Gut-lung axis and dysbiosis in COVID-19. Turk J Biol 2020;44:265-72. [Crossref] [PubMed]
- Proctor DM, Relman DA. The Landscape Ecology and Microbiota of the Human Nose, Mouth, and Throat. Cell Host Microbe 2017;21:421-32. [Crossref] [PubMed]
- Mantis NJ, Rol N, Corthesy B. Secretory IgA’s complex roles in immunity and mucosal homeostasis in the gut. Mucosal Immunol 2011;4:603-11. [Crossref] [PubMed]
- Wang H, Liu JS, Peng SH, et al. Gut-lung crosstalk in pulmonary involvement with inflammatory bowel diseases. World J Gastroenterol 2013;19:6794-804. [Crossref] [PubMed]
- Wang J, Li F, Tian Z. Role of microbiota on lung homeostasis and diseases. Sci China Life Sci 2017;60:1407-15. [Crossref] [PubMed]
- Libertucci J, Young VB. The role of the microbiota in infectious diseases. Nat Microbiol 2019;4:35-45. [Crossref] [PubMed]
- Gupta A, Madhavan MV, Sehgal K, et al. Extrapulmonary manifestations of COVID-19. Nat Med 2020;26:1017-32. [Crossref] [PubMed]
- Vogel K, Blumer N, Korthals M, et al. Animal shed Bacillus licheniformis spores possess allergy-protective as well as inflammatory properties. J Allergy Clin Immunol 2008;122:307-12, 12.e1-8.
- Bandeira de Melo C, Zhang Z, Shi L, et al. Dietary Fiber Intake Regulates Intestinal Microflora and Inhibits Ovalbumin-Induced Allergic Airway Inflammation in a Mouse Model. PLoS One 2016;11:e0147778 [Crossref] [PubMed]
- Doucet D, Badami C, Palange D, et al. Estrogen receptor hormone agonists limit trauma hemorrhage shock-induced gut and lung injury in rats. PLoS One 2010;5:e9421 [Crossref] [PubMed]
- Dowman L, Hill CJ, Holland AE. Pulmonary rehabilitation for interstitial lung disease. Cochrane Database Syst Rev 2014;CD006322 [PubMed]
- Samuelson DR, Welsh DA, Shellito JE. Regulation of lung immunity and host defense by the intestinal microbiota. Front Microbiol 2015;6:1085. [Crossref] [PubMed]
- Fyhrquist N, Ruokolainen L, Suomalainen A, et al. Acinetobacter species in the skin microbiota protect against allergic sensitization and inflammation. J Allergy Clin Immunol 2014;134:1301-9.e11. [Crossref] [PubMed]
- Koch KN, Hartung ML, Urban S, et al. Helicobacter urease-induced activation of the TLR2/NLRP3/IL-18 axis protects against asthma. J Clin Invest 2015;125:3297-302. [Crossref] [PubMed]
- Gill SR, Pop M, Deboy RT, et al. Metagenomic analysis of the human distal gut microbiome. Science 2006;312:1355-9. [Crossref] [PubMed]
- Greenhalgh K, Meyer KM, Aagaard KM, et al. The human gut microbiome in health: establishment and resilience of microbiota over a lifetime. Environ Microbiol 2016;18:2103-16. [Crossref] [PubMed]
- El-Ghiaty MA, Shoieb SM, El-Kadi AOS. Cytochrome P450-mediated drug interactions in COVID-19 patients: Current findings and possible mechanisms. Med Hypotheses 2020;144:110033 [Crossref] [PubMed]
- Banales JM, Huebert RC, Karlsen T, et al. Cholangiocyte pathobiology. Nat Rev Gastroenterol Hepatol 2019;16:269-81. [Crossref] [PubMed]
- Pan L, Mu M, Yang P, et al. Clinical Characteristics of COVID-19 Patients With Digestive Symptoms in Hubei, China: A Descriptive, Cross-Sectional, Multicenter Study. Am J Gastroenterol 2020;115:766-73. [Crossref] [PubMed]
- Beigmohammadi MT, Jahanbin B, Safaei M, et al. Pathological Findings of Postmortem Biopsies From Lung, Heart, and Liver of 7 Deceased COVID-19 Patients. Int J Surg Pathol 2020; [Epub ahead of print]. [Crossref] [PubMed]
- Wu HT, Chuang YW, Huang CP, et al. Loss of angiotensin converting enzyme II (ACE2) accelerates the development of liver injury induced by thioacetamide. Exp Anim 2018;67:41-9. [Crossref] [PubMed]
- Alqahtani SA, Schattenberg JM. Liver injury in COVID-19: The current evidence. United European Gastroenterol J 2020;8:509-19. [Crossref] [PubMed]
- Liu B, Li M, Zhou Z, et al. Can we use interleukin-6 (IL-6) blockade for coronavirus disease 2019 (COVID-19)-induced cytokine release syndrome (CRS)? J Autoimmun 2020;111:102452 [Crossref] [PubMed]
- Cheng H, Wang Y, Wang GQ. Organ-protective effect of angiotensin-converting enzyme 2 and its effect on the prognosis of COVID-19. Journal of Medical Virology 2020;92:726-30. [Crossref] [PubMed]
- Ling Y, Xu SB, Lin YX, et al. Persistence and clearance of viral RNA in 2019 novel coronavirus disease rehabilitation patients. Chin Med J (Engl) 2020;133:1039-43. [Crossref] [PubMed]
- Zhong P, Xu J, Yang D, et al. COVID-19-associated gastrointestinal and liver injury: clinical features and potential mechanisms. Signal Transduct Target Ther 2020;5:256. [Crossref] [PubMed]
- Waseem N, Chen PH. Hypoxic Hepatitis: A Review and Clinical Update. J Clin Transl Hepatol 2016;4:263-8. [PubMed]
- Zhao D, Yao F, Wang L, et al. A Comparative Study on the Clinical Features of Coronavirus 2019 (COVID-19) Pneumonia With Other Pneumonias. Clin Infect Dis 2020;71:756-61. [Crossref] [PubMed]
- European Association for the Study of the Liver. EASL Clinical Practice Guidelines: Drug-induced liver injury. J Hepatol 2019;70:1222-61. [Crossref] [PubMed]