Predictive value of change in effective arterial elastance in norepinephrine weaning: a retrospective study
Introduction
The hemodynamic profile of septic shock is characterized by low systemic vascular resistance (SVR), high cardiac output (CO), and presents persistent low blood pressure as a clinical feature (1). Fluid therapy and vasoactive support are the cornerstones of septic shock resuscitation (2). These two main components are used to achieve target pressure and to provide adequate tissue perfusion. Meanwhile, norepinephrine is the first-choice vasopressor recommended to correct refractory hypotension due to depressed vascular tone (3). However, exposure to high dose of norepinephrine over one hour might lead to adverse events, such as myocardial cell injury, tissue ischemia, and alteration of sepsis-associated immunomodulation (4,5). Once the patient is stable and has received adequate fluid resuscitation, great effort should be directed towards decreasing the norepinephrine requirements (2,3). However, norepinephrine weaning is usually an empirical choice made by clinicians. There is insignificant published evidence on how to assess the optimal time to initiate norepinephrine weaning (6-8).
A complete description of the cardiovascular system should be considered in optimizing the norepinephrine weaning process. In this regard, the assessment of the effective arterial elastance (Ea) helps clinicians better understand the cardiovascular performance in circulatory failure and hemodynamic therapy (9-11). Ea is the net afterload, which can be acquired from the left ventricular pressure-volume loop. It integrates steady and pulsatile components of arterial load (12-14) and comprehensively reflects the left ventricular afterload better than the SVR or arterial pressure (12,15). Ea also has been regarded as the mechanical energetic aspect of heart-arterial interaction (1,16-18). The arterial system does not only act as the conduit for blood flow to provide tissue perfusion but also modulates ventricular ejection and maintains blood pressure. Analytical work revealed that the heart delivers maximal stroke work when Ea and left ventricular end-systolic elastance match (17-19).
In the past decades, a robust Ea estimate by 90% of systolic arterial pressure (SAP) over stroke volume (SV) ratio has been clinically used. Validation studies have proven that the Ea reliability acquired at bedside represented the arterial load (13,14,20). However, Ea itself has not been widely applied in clinical practice. The major reason might be the Ea’s misunderstanding as a surrogate of vascular resistance, because the algorithm of Ea is similar to SVR. Meanwhile, most literature has been focused on exploring Ea to predict pressure responsiveness (8,21). Therefore, this study design was to observe Ea’s ability and change according to a fluid challenge in the prediction of early norepinephrine weaning among critically septic patients. We present the following article in accordance with the STROBE reporting checklist (available at http://dx.doi.org/10.21037/apm-21-482).
Methods
Setting
This was a retrospective analysis of prospectively collected data from septic shock patients admitted to the Medical Intensive Care Unit (MICU) of Peking Union Medical College Hospital. The study was conducted in accordance with the Declaration of Helsinki (as revised in 2013). This study was approved by the Ethical Committee of Peking Union Medical College Hospital (ZS1085) and informed consent was waived because of the retrospective study design.
Patients, hemodynamic monitoring
We screened patients who were diagnosed with septic shock from October 2015 to August 2020. Septic shock was defined according to international criteria (3). Included were patients with persistent hypotension who needed vasopressors to maintain SAP no less than 90 mmHg or mean arterial pressure (MAP) higher than 65 mmHg after initial fluid resuscitation of 30 mL/kg in accordance with sepsis campaign bundle. All patients received a fluid challenge. For the fluid challenge, 500 mL of 4% gelatin or normal saline (NS) was given over 5–10 min using a bag pressurized to 300 mmHg. The exclusion criteria included other types of diagnosed shock, the receipt of other vasopressor more than norepinephrine, the receipt of other CO monitoring tools utilizing pulse-contour analysis, such as pulse index continuous cardiac output (PiCCO), patients younger than 18, pregnancy, patients with “do-not-resuscitate” orders, and patients with a survival time of less than 24 hours after fluid challenge.
The arterial blood pressure was monitored from an arterial line (Becton Dickinson Infusion Therapy Systems Inc., Utah, USA) placed in radial artery. All patients were indwelled with a pulmonary artery catheter (PAC) and received continuous CO monitoring by thermodilution (Swan-Ganz CCOmbo CCO/SvO2, Vigilance II™ monitor, Edwards Lifesciences, Irvine, CA, USA). Hemodynamic variables were formulated and presented by use of the IntelliVue Patient Monitor MP70 (Philips Medical System, Boeblingen, Germany).
Data collected
Hemodynamic variables before and after fluid challenge were recorded, including heart rate (HR), SAP, MAP, central venous pressure (CVP), CO, SV and SVR. Measures of arterial load were calculated at bedside, as Ea =90% of SAP/SV (1,14), net arterial compliance (C) = SV/arterial pulse pressure. Time 0 (T0) was defined as the time point before fluid challenge, Time 1 (T1) was immediately after fluid challenge, six hours (T6) and 24 hours (T24) were identified. We collected norepinephrine doses at T0, T6, T24, T48 and T72 and cumulative fluid intake, fluid output and diuresis at T6, T24, T48 and T72. There was no missing data for norepinephrine dose during the first 3 days, because it was the used crucial information in the critical care databases. Missing data for cumulative fluid output were obtained from relevant information in the medical records or calculated from the daily nursing record sheets.
Definitions
- NE weaning was defined as the dose of norepinephrine reduced or maintained stable at T6 [NE (T6) – NE (T0), ΔNE ≤0 µg/min];
- NE worsening was defined as the dose of norepinephrine increased at T6: [NE (T6) – NE (T0), ΔNE ≥1 µg/min];
- ΔEa% was defined as the percentage of Ea reduction after fluid challenge: [Ea (T0) – Ea (T1)]/Ea (T0);
- Preload responder was defined as an increase in CO greater than 10% after fluid challenge; meanwhile, a 10% increase in MAP was defined as pressure responder (1,22);
- The discontinuation of norepinephrine was defined as a norepinephrine-free period of at least 24 h.
Outcomes
The primary outcome was to assess the viability of Ea and other hemodynamic variables to detect norepinephrine weaning at T6. Secondary outcomes were the following: the cumulative duration of norepinephrine treatment after inclusion, the fluid intake, diuresis and fluid balance (expressed in mL/kg) in first 6 hours until 3 days from inclusion.
Statistical analysis
Categorical data were expressed as number (percentage). Continuous variables were expressed as the means ± standard deviation (SD) or median with interquartile ranges (IQR) as appropriate. The Student’s t or Mann-Whitney U test for the comparison of continuous data and Chi-square test for categorical data were used. The receiver operator characteristic (ROC) curves generated to describe ΔEa% to predict norepinephrine weaning. The area under the ROC curves (AUCs) were calculated as well. DeLong test was used to compare the AUCs for ΔEa%, a combination of ΔEa% with other parameters in predicting norepinephrine weaning. Potential related factors added into the model included demographics (age and gender) and past-medical history (hypertension, diabetes mellitus, and chronic kidney disease), which might change the arterial system and affect norepinephrine weaning (23). The associations between norepinephrine weaning and potential predictive factors were initially assessed using univariate analyses. The variables associated with norepinephrine weaning in the univariate analysis with a P value <0.2 in at least one comparison were included in the multivariate analysis. Multivariate analysis was performed using binary logistic regression to explore the ability of ΔEa% and other indicators to predict norepinephrine weaning in sepsis resuscitation. The odds ratios (OR) was displayed with a 95% confidence interval (CI). The probability for remaining under norepinephrine was analyzed with the Kaplan-Meier method using the Gehan-Breslow-Wilcoxon test to compare between groups.
Statistical analyses were performed with SPSS 25.0 software (SPSS, Inc., Chicago, IL), MedCalc (statistical software e version 15.6.1 for Windows) and GraphPad Prism 7. A value of P<0.05 was considered to be statistically significant.
Results
One hundred and eight patients were retrospectively screened and analyzed. All patients received norepinephrine support with a median dose of 0.56 (0.32–1.13) µg∙kg−1∙min−1 at T0. The most common sources of septic shock were pulmonary (67, 62.0%) and abdomen (22, 20.4%). There were 37 (34.3%), 24 (22.2%) and 18 (16.7%) patients who had a prior medical history of hypertension, diabetic mellitus, and chronic kidney disease, respectively. The baseline demographic data and clinical characteristics are shown in Table 1.
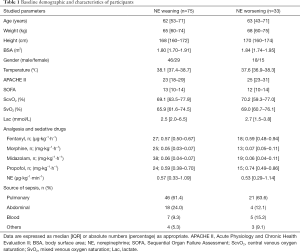
Full table
ΔEa% predicts early initiation of norepinephrine weaning
Patients were allocated into two groups according to NE weaning (ΔNE ≤0 µg/min, n=75) and NE worsening (ΔNE ≥1 µg/min, n=33). The hemodynamic variables at T0 and T1 were assessed (Table 2). In the univariate analysis, Ea at T0 (OR =0.52; 95% CI: 0.29–0.93; P=0.029), ΔEa% (OR =0.98; 95% CI: 0.98–1.00; P=0.049), and ΔCO% (OR =0.96; 95% CI: 0.93–0.99; P=0.011) were associated with norepinephrine weaning, while ΔMAP% (OR =0.55; 95% CI: 0.23–1.32; P=0.183), ΔSVR% (OR =0.99; 95% CI: 0.97–1.02; P=0.188) were not associated with norepinephrine weaning.
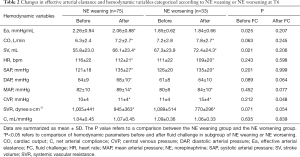
Full table
The multivariate analysis showed that ΔEa% (OR =0.95; 95% CI: 0.89-0.99; P=0.003), ΔCO% (OR =0.94; 95% CI: 0.91–0.99; P=0.015), ΔSVR% (OR =0.92; 95% CI: 0.87–0.98; P=0.013) and ΔMAP% (OR =0.94; 95% CI: 0.89–0.98; P=0.010) were independent predictive factors for norepinephrine weaning at T6. The AUCs of ΔEa% to predict norepinephrine weaning was at 0.64 (95% CI: 0.52–0.75; P=0.026). To predict norepinephrine weaning, the optimum thresholds for ΔEa% was 5.1% [sensitivity: 61%, specificity: 67%, positive likelihood ratio (+LR) 1.84, negative likelihood ratio (−LR) 0.58]. Notably, the combination of ΔEa%, ΔSVR% and ΔCO% was associated with norepinephrine weaning [AUC at 0.73 (95% CI: 0.64–0.83); P=0.001] whereas the combination of ΔEa% and ΔMAP% was at 0.71 (95% CI: 0.61–0.81); P<0.001 (Figure 1). In the pairwise comparison of ROC curves, the AUC of ΔEa% + ΔCO% + ΔSVR% was higher than that of ΔEa% along (Z=1.968; P=0.049), whereas no difference was observed when comparison was made between ΔEa% and ΔEa% + ΔMAP%, or between ΔEa% + ΔMAP% and ΔEa% + ΔCO% + ΔSVR% (Z=1.519, P=0.129 and Z=0.896, P=0.371, respectively).
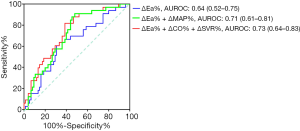
Norepinephrine weaning and cumulative use of norepinephrine
The norepinephrine dose at T0 was similar between the two groups: 0.57 (0.33–1.09) vs. 0.53 (0.29–1.14) µg∙kg−1∙min−1, P=0.971. In groups of NE weaning, norepinephrine dose decreased to 0.47 (0.29–0.82) µg∙kg−1∙min−1 at T6, whereas a value of 0.65 (0.40–1.30) µg∙kg−1∙min−1 was shown in the NE worsening group at T6, P=0.013. The tendency of norepinephrine weaning during the first 72 hours from inclusion is displayed in Figure 2A. A discontinuation of norepinephrine was achieved in 50 (66.7%) of the 75 NE weaning group and in 18 (54.5%) of 33 NE worsening group. Forty patients did not achieve discontinuation of norepinephrine due to they died before norepinephrine weaning (no significant difference between groups). Median (IQR) duration (in hours) of norepinephrine was significantly shorter in the NE weaning group: 48 [34–89] vs. 72 [54–90] hours, P=0.048. The median time of cumulative probability remaining under norepinephrine of the two groups is shown by the Kaplan-Meier plot in Figure 2B.
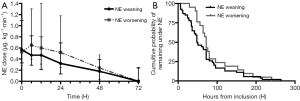
Fluid administration and fluid balance in both groups
The data regarding fluid administration and fluid balance are displayed in Figure 3 and Table 3. The fluid intake and fluid balance reduced day-by-day in both groups from inclusion to 72 h, and there was no significantly difference between groups. Meanwhile, the daily administered fluid amounts were higher in the NE weaning group (Figure 3A); on the contrary, the daily amounts of diuresis were lower in the NE weaning group (Figure 3B), but no statistical difference was observed between groups. As a result, the fluid balance in the NE weaning group was insignificantly higher than that of the NE worsening group, but there was no significant difference between two groups at each time points (P>0.05, Figure 3C).
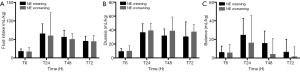
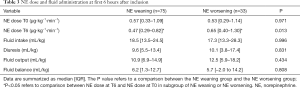
Full table
Effect of fluid type: gelatin vs. normal saline
Except ΔCO%, there was no significant difference in the blood pressure and arterial load changes after fluid challenge between the gelatin group and the normal saline group (Table 4).
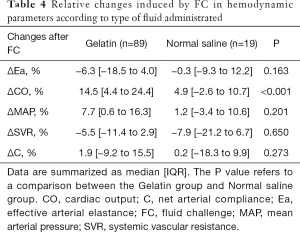
Full table
Effective arterial elastance in fluid challenge
Of the 108 patients, 62 (57.4%) were preload responders (R) to the fluid challenge, whereas 46 (42.6%) were pressure responders (NR). Only 28 (25.9%) patients were both preload responders and pressure responders. Changes in hemodynamic variables before and after fluid challenge in R and NR categorized according to CO are shown in Table 5. Fluid responsive individuals had expected changes in CO, SV, and SVR over the study interval. Fluid infusion decreased Ea from 2.36±0.94 to 2.03±0.89 mmHg/mL for preload-R, whereas Ea of patients with negative preload response increased from 1.84±0.67 to 1.94±0.72 mmHg/mL.
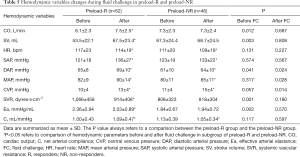
Full table
Discussion
Our study explored that ΔEa% induced by fluid challenge was able to predict the early initiation of norepinephrine weaning. A combination of ΔEa%, ΔSVR% and ΔCO% provided an increased level of prediction. In addition, a significant reduction in Ea after fluid challenge was a predictive factor to norepinephrine weaning, although this reduction has also been used to explain why preload responders without increasing blood pressure. We highlighted that timely application of Ea strengthened the clinical decision to promote norepinephrine weaning as early as possible, particularly at bedside in septic shock patients.
Once the patient is hemodynamically stable following a thorough assessment of perfusion status, vasopressor weaning should commence. The early goal-directed therapy (EGDT) takes account of the infusion vasopressor’s early initiation to achieve a predefined hemodynamic goal; subsequent hemodynamic management is left to the clinician’s discretion (8). The optimum method for weaning vasopressors is subject to much controversy, although the classical method recommends titration of the vasopressors by monitoring the MAP (3,6,7). Ea in some way explains the arterial system’s performance in hemodynamic resuscitation. In the NE weaning group, early initiation of norepinephrine weaning was associated with a shorter duration of vasopressor exposure, while a similar fluid intake and balance were applied. This study was the first to evaluate Ea as a means to assess the early initiation possibilities of norepinephrine weaning. Further studies are needed to validate the clinical value of Ea, while an individualized approach could be applied in order to improve patient’s management.
It is generally believed that patients with both preload and pressure positive response might have prospects of a better outcome. There can be no argument that the reserve of cardiac function, expressed as fluid infusion leading to a greater increase in CO, is a protective factor of norepinephrine weaning, but the change in Ea (ΔEa%) demonstrates an attractive result. Previous studies have reported that maintaining Ea stability was associated with positive pressure responsiveness during fluid challenge, hypothesizing that the loss of arterial load is the primary reason for an exhibited a CO increase in septic patients after fluid administration without an improvement in blood pressure (6,19,24,25). Therefore, one can hypothesize that an unchanged Ea, performed in fluid administration, was a predictive factor of norepinephrine weaning. Meanwhile, according to the concept of ventriculoarterial coupling (VAC), Ea was assumed to concomitantly contribute to a preload increase in order to match a maximal stroke work (13,17,26). However, other studies found that fluid administration induced an Ea reduction. There are several possible explanations. First, the sympathetic nervous system contributes to vasoconstriction in response to hypovolemia and hypotension. After fluid infusion, the baroreflex changes, and a Ea decrease is the response in order to adapt the modulation of blood volume, arterial tone, and ventricular work. Sakamoto et al. has demonstrated that the vascular properties predominantly contribute to baroreflex regulation of arterial pressure, rather than ventricular properties (26,27). Second, fluid loading during a short-time period may stimulate the production of nitric oxide and endothelial shear stress, leading to a reduction of arterial tone (28). Third (and a related possibility) is that the increased resistance and impedance were triggered by intravenous fluid replacement. In Sagawa’s analytical model, the ratio of Ea and end-systolic elastance of 50% was thought to reach the maximal mechanical efficiency of ventricular contraction (16). The following considerations need further study: (I) determining whether an appropriate therapeutic approach is the restoration of the vascular system’s autoregulation and (II) whether a decrease in Ea induced by fluid loading indicates VAC optimization (18).
We used a combination of these markers to provide a better level of prediction. The combination of ΔEa%, ΔSVR% and ΔCO%, which include the factors of the cardiac system, arterial load, and the major vascular system, demonstrated better prediction ability. A significant reduction in Ea and an increase in CO and SVR following fluid challenge predicted a higher chance of norepinephrine weaning. It is worth noting that although Ea and SVR included the component of vascular resistance, the small and large vessels presented different responses to increased flow (29). An opposite trend in changes of Ea and SVR has therefore, been established in this predictive equation. Moreover, the baseline Ea, was able to predict NE weaning, but in critically ill patients with septic shock, a norepinephrine infusion increases arterial pressure through vasoconstrictive effects (30-32). Thus, some patients presented normal SVR and higher Ea, especially in severe septic shock. It is troublesome to use a normal range of SVR and Ea to predict hemodynamic performance at bedside. Therefore, we selected the changes in Ea and SVR in this prediction model. Actually, they were found to be more reliable and useful as predictors due to their adjustment by baseline value. Additionally, considering that demographics and characteristics might affect the efficiency and therapy outcome, we selected data for analysis based on the patients’ age and gender, having received concurrent doses of norepinephrine infusion, along with their medical history including prior hypertensive disease, chronic kidney disease and diabetes mellitus. The clinical improvement was independent of concurrent norepinephrine and other factors mentioned above.
Ea shares the same units of ventricle end-systolic elastance (Ees). Ea therefore, has been most often applied to assess cardiovascular efficiency. Ea, taken in isolation, is commonly considered as an index of arterial load (12,33), which compromises different arterial properties and the effects of arterial wave reflections. Although Ea is a complex lumped parameter, Ea plays an important role in physiological regulation of the heart and arterial system. Since the estimation of Ea by 90% of SAP over SV ratio has been clinically used, the Ea calculation leads to confusion when trying to understand changes in Ea. Mathematically, Ea is calculated from arterial pressure and stroke volume; from a physiological point, Ea integrates arterial properties both in steady and pulsatile components. The Ea, arterial pressure, and blood flow value are actually the independent variables (three components) of cardiovascular system. Therefore, Ea, along with the Ea changes, should not be considered as a spurious mathematical coupling effect (24). We should keep this in mind when analyzing Ea during hemodynamic therapy.
To enhance the Ea’s understanding in clinical practice, we analyzed the data before and after fluid infusion. Generalized vasodilation is the septic shock’s typical hemodynamic profile, leading to refractory hypotension. In our study, the vascular system’s manifestation was an increase in Ea but a decrease in SVR. Previous experimental studies suggested that aortic wall edema caused by sepsis could contribute to a persistent elevation in aortic characteristic impedance, whereas the pharmacological vasoconstriction induced by the fluid infusion or exogenous vasoactive drug might also be a major factor in inducing an Ea increase (21,26,33,34). Ea has been regarded as a product of the vascular system’s response to an SV increase (1). Still, caution should be taken to ensure that a higher pre-infusion Ea did not instigate an improved pressure response to fluid challenge—no significant difference in pre-infusion Ea was observed between pressure-R and pressure-NR. In this respect, our findings displayed similarities to previous studies (24,25). Simultaneously, we found that prior to fluid challenge, Ea was higher in preload-R than in preload-NR, and a baseline Ea greater than 1.97 mmHg/mL can predict preload responsiveness. However, the baseline value of Ea differed in previous studies due to the different measurements given (19,24,25). Moreover, recent studies reported that a higher Eadyn might indicate greater efficiency of the cardiovascular system in delivering energy to the arterial system for sustaining blood flow (35,36). Based on this, we were only able to suggest that a higher pre-infusion Ea might indicate a positive preload response to fluid challenge, without using an accurate predictive value.
Several limitations of this study require discussion. First, it is a retrospective analysis of previously collected data, although we documented the data before and after fluid challenge and also evaluated the added value of Ea in assessing fluid challenge and further therapy. The most important hemodynamic data, including Ea and CO, were not completely recorded at T6 and T24. Therefore, even though the sample size was not small, it was hard to continuously trace Ea changes across 24 h and provide more valuable clinical evidence. Second, the blood pressure was obtained from the radial artery. Although arterial load indices derived from the radial artery have been verified as similar to aortic (20,37), Jozwiak demonstrated that a femoral estimate of Ea was supposed to be more accurate and precise than the radial estimate (38). However, our study used the changes in Ea and cardiac vascular variables induced by fluid in the prediction model; the dynamic changes in hemodynamic indicators were more meaningful than the baseline value itself. Third, we did not validate the potential use of Ea to titrate norepinephrine weaning at bedside, which was the major reason why the long-term prognosis of the two group patients were similar in this study. Fourth, this study excluded patients who received vasopressors other than norepinephrine - the different receptors of dobutamine or epinephrine would affect the heart pump and arterial load. Further validation studies are needed to confirm the value of Ea in constituting an approach to infusion and weaning off norepinephrine and other vasopressors.
Conclusions
The assessment of arterial load by effective arterial elastance in septic shock patients may assist clinicians in detecting patients with whom it is possible to initiate norepinephrine weaning at the early phase of resuscitation. Early initiation of norepinephrine weaning was associated with shorter duration of norepinephrine exposure. A combination of Ea, CO and SVR according to fluid challenge provided an improved prediction ability. Further studies are needed to confirm the results and to optimize the hemodynamic treatment of patients with septic shock and acute circulatory failure.
Acknowledgments
We appreciate Yibing Zhu, from Medical Research and Biometrics Center, National Center for Cardiovascular Diseases, Fuwai Hospital, Chinese Academy of Medical Sciences and Peking Union Medical College for her kind suggestions on the statistical issue. The authors thank AiMi Academic Services (
Funding: This study has been supported by the Ministry of Science and Technology of People’s Republic of China (2020YFC0841300).
Footnote
Reporting Checklist: The authors have completed the STROBE reporting checklist. Available at http://dx.doi.org/10.21037/apm-21-482
Data Sharing Statement: Available at http://dx.doi.org/10.21037/apm-21-482
Conflicts of Interest: All authors have completed the ICMJE uniform disclosure form (available at http://dx.doi.org/10.21037/apm-21-482). The authors have no conflicts of interest to declare.
Ethical Statement: The authors are accountable for all aspects of the work in ensuring that questions related to the accuracy or integrity of any part of the work are appropriately investigated and resolved. The study was conducted in accordance with the Declaration of Helsinki (as revised in 2013). Ethical approval for the study was provided by the Ethical Committee of Peking Union Medical College Hospital (ZS-1085). Informed consent was waived because of the retrospective study design.
Open Access Statement: This is an Open Access article distributed in accordance with the Creative Commons Attribution-NonCommercial-NoDerivs 4.0 International License (CC BY-NC-ND 4.0), which permits the non-commercial replication and distribution of the article with the strict proviso that no changes or edits are made and the original work is properly cited (including links to both the formal publication through the relevant DOI and the license). See: https://creativecommons.org/licenses/by-nc-nd/4.0/.
References
- Guarracino F, Baldassarri R, Pinsky MR. Ventriculo-arterial decoupling in acutely altered hemodynamic states. Critical Care 2013;17:213. [Crossref] [PubMed]
- Levy MM, Evans LE, Rhodes A. The Surviving Sepsis Campaign Bundle: 2018 update. Intensive Care Med 2018;44:925-8. [Crossref] [PubMed]
- Dellinger RP, Levy MM, Rhodes A, et al. Surviving Sepsis Campaign: international guidelines for management of severe sepsis and septic shock, 2012. Intensive Care Med 2013;39:165-228. [Crossref] [PubMed]
- Stolk RF, van der Poll T, Angus DC, et al. Potentially inadvertent immunomodulation: norepinephrine use in sepsis. Am J Respir Crit Care Med 2016;194:550-8. [Crossref] [PubMed]
- Anantasit N, Boyd JH, Walley KR, et al. Serious adverse events associated with vasopressin and norepinephrine infusion in septic shock. Crit Care Med 2014;42:1812-20. [Crossref] [PubMed]
- Arellano DL, Hanneman SK. Vasopressor weaning in patients with septic shock. Crit Care Nurs Clin North Am 2014;26:413-25. [Crossref] [PubMed]
- Merouani M, Guignard B, Vincent F, et al. Norepinephrine weaning in septic shock patients by closed loop control based on fuzzy logic. Crit Care 2008;12:R155. [Crossref] [PubMed]
- Guinot PG, Abou-Arab O, Guilbart M, et al. Monitoring dynamic arterial elastance as a means of decreasing the duration of norepinephrine treatment in vasoplegic syndrome following cardiac surgery: a prospective, randomized trial. Intensive Care Med 2017;43:643-51. [Crossref] [PubMed]
- Monnet X, Letierce A, Hamzaoui O, et al. Arterial pressure allows monitoring the changes in cardiac output induced by volume expansion but not by norepinephrine. Crit Care Med 2011;39:1394-9. [Crossref] [PubMed]
- Cecconi M, Hofer C, Teboul JL, et al. Fluid challenges in intensive care: the FENICE study: A global inception cohort study. Intensive Care Med 2015;41:1529-37. Erratum in: Intensive Care Med 2015;41:1737-8. [Crossref] [PubMed]
- Boulain T, Boisrame-Helms J, Ehrmann S, et al. Volume expansion in the first 4 days of shock: a prospective multicentre study in 19 French intensive care units. Intensive Care Med 2015;41:248-56. [Crossref] [PubMed]
- Monge García MI, Saludes Orduna P, Cecconi M. Understanding arterial load. Intensive Care Med 2016;42:1625-7. [Crossref] [PubMed]
- Sunagawa K, Maughan WL, Burkhoff D, et al. Left ventricular interaction with arterial load studied in isolated canine ventricle. Am J Physiol 1983;245:H773-80. [PubMed]
- Kelly RP, Ting CT, Yang TM, et al. Effective arterial elastance as index of arterial vascular load in humans. Circulation 1992;86:513-21. [Crossref] [PubMed]
- Segers P, Stergiopulos N, Westerhof N. Relation of effective arterial elastance to arterial system properties. Am J Physiol Heart Circ Physiol 2002;282:H1041-6. [Crossref] [PubMed]
- Burkhoff D, Sagawa K. Ventricular efficiency predicted by an analytical model. Am J Physiol 1986;250:R1021-7. [PubMed]
- Asanoi H, Sasayama S, Kameyama T. Ventriculoarterial coupling in normal and failing heart in humans. Circulation Research 1989;65:483-93. [Crossref] [PubMed]
- Guarracino F, Ferro B, Morelli A, et al. Ventriculoarterial decoupling in human septic shock. Critical Care 2014;18:R80. [Crossref] [PubMed]
- Li S, Wan X, Laudanski K, et al. Left-Sided Ventricular-arterial coupling and volume responsiveness in septic shock patients. Shock 2019;52:577-82. [Crossref] [PubMed]
- Hatib F, Jansen JR, Pinsky MR. Peripheral vascular decoupling in porcine endotoxic shock. J Appl Physiol (1985) 2011;111:853-60. [Crossref] [PubMed]
- Guarracino F, Bertini P, Pinsky MR. Cardiovascular determinants of resuscitation from sepsis and septic shock. Crit Care 2019;23:118. [Crossref] [PubMed]
- Sunagawa K, Maughan WL, Sagawa K. Optimal arterial resistance for the maximal stroke work studied in isolated canine left ventricle. Circulation Research 1985;56:586-95. [Crossref] [PubMed]
- McEniery CM, Wilkinson IB, Avolio AP. Age, hypertension and arterial function. Clin Exp Pharmacol Physiol 2007;34:665-71. [Crossref] [PubMed]
- Monge García MI, Guijo Gonzalez P, Gracia Romero M, et al. Effects of fluid administration on arterial load in septic shock patients. Intensive Care Med 2015;41:1247-55. [Crossref] [PubMed]
- Monge García MI, Gil Cano A, Gracia Romero M. Dynamic arterial elastance to predict arterial pressure response to volume loading in preload-dependent patients. Crit Care 2011;15:R15. [Crossref] [PubMed]
- Cholley BP, Lang RM, Berger DS, et al. Alterations in systemic arterial mechanical properties during septic shock: role of fluid resuscitation. Am J Physiol 1995;269:H375-84. [PubMed]
- Sakamoto T, Kakino T, Sakamoto K, et al. Changes in vascular properties, not ventricular properties, predominantly contribute to baroreflex regulation of arterial pressure. Am J Physiol Heart Circ Physiol 2015;308:H49-58. [Crossref] [PubMed]
- Losser MR, Forget AP, Payen D. Nitric oxide involvement in the hemodynamic response to fluid resuscitation in endotoxic shock in rats. Crit Care Med 2006;34:2426-31. [Crossref] [PubMed]
- Cryer HM, Garrison RN, Harris PD. Role of muscle microvasculature during hyperdynamic and hypodynamic phases of endotoxin shock in decerebrate rats. J Trauma 1988;28:312-8. [Crossref] [PubMed]
- Persichini R, Silva S, Teboul JL, et al. Effects of norepinephrine on mean systemic pressure and venous return in human septic shock. Crit Care Med 2012;40:3146-53. [Crossref] [PubMed]
- Levy B, Perez P, Perny J, et al. Comparison of norepinephrine-dobutamine to epinephrine for hemodynamics, lactate metabolism, and organ function variables in cardiogenic shock. A prospective, randomized pilot study. Crit Care Med 2011;39:450-5. [Crossref] [PubMed]
- De Backer D, Biston P, Devriendt J, et al. Comparison of dopamine and norepinephrine in the treatment of shock. N Engl J Med 2010;362:779-89. [Crossref] [PubMed]
- Pinsky MR, Guarracino F. How to assess ventriculoarterial coupling in sepsis. Curr Opin Crit Care 2020;26:313-8. [Crossref] [PubMed]
- Murphy L, Davidson S, Knopp JL, et al. State analysis of total stressed blood volume and arterial elastance during induced sepsis. Annu Int Conf IEEE Eng Med Biol Soc 2019;2951-4.
- Monge Garcia MI, Guijo Gonzalez P, Saludes Orduna P, et al. Dynamic arterial elastance during experimental endotoxic septic shock: a potential marker of cardiovascular efficiency. Front Physiol 2020;11:562824 [Crossref] [PubMed]
- Mannozzi J, Kaur J, Spranger MD, et al. Muscle metaboreflex-induced increases in effective arterial elastance: effect of heart failure. Am J Physiol Regul Integr Comp Physiol 2020;319:R1-R10. [Crossref] [PubMed]
- Pauca AL, Wallenhaupt SL, Kon ND, et al. Does radial artery pressure accurately reflect aortic pressure? Chest 1992;102:1193-8. [Crossref] [PubMed]
- Jozwiak M, Millasseau S, Richard C, et al. Validation and critical evaluation of the effective arterial elastance in critically ill patients. Crit Care Med 2019;47:e317-e324. [Crossref] [PubMed]