The combined use of gamma-aminobutyric acid and walnut peptide enhances sleep in mice
Introduction
Sleep is a complex behavior affected by both physical and psychological influences. Adequate sleep is important for health and sleep deprivation can lead to multiple functional problems (1). Animals deprived of sleep typically show poorer neurobiological performance. In addition, sleep loss increases blood-brain barrier permeability and alters fertility concomitantly with the disruption of the blood-tissue barriers at the reproductive tract (2). Increases in negative mood states (including anxiety, depression, and suicidal tendency) and amplification of basic emotional responses are strongly associated with sleep loss (3). Studies have shown a link between sleep restriction and the loss of muscle mass in both human and animal models (4).
A cross-sectional survey across 15 provinces showed that the sleep insufficiency rate in people aged 18–64 years was 9.8% and the proportion of elderly with insufficient sleep was 14.2% (5). The use of hypnotic drugs, such as benzodiazepines and benzodiazepine‐related drugs for the clinical treatment of sleep disorders can be complicated by excessive use and dependence issues. There are approximately 342 accidental deaths due to sedative/hypnotic drug overdose per 100,000 persons in the United States (6). A meta‐analysis showed that benzodiazepine‐related drugs users had a 1.25‐fold increased risk of pneumonia compared to patients who did not use such drugs (7). The demand for medication with naturally sourced materials has increased in recent years. Thus, it is important to identify novel ingredients from natural sources that have high efficacy for sleep disorders but limited does-related toxicity.
Exogenous γ-aminobutyric acid (GABA) plays an important role in the regulation of the mammalian central nervous system (CNS). Studies have shown that 100 mg GABA greatly shortened sleep latency and non-rapid eye movement (non-REM) sleep latency, thereby enabling patients to fall asleep quickly and easily (8). In 2001, Japan added GABA to the food line and in 2008, the United States listed GABA as a recognized safe substance (No. 000257) permitted in food and drinks. In 2009, China’s Ministry of Health approved GABA as a new resource food. As a biologically active substance with various physiological functions, GABA can be used in beverages, cocoa products, chocolate products, confectionery, baked goods, and puffed foods. Glutamine is the raw material for synthesis of GABA. It plays an important role in the glutamate/GABA-glutamine cycle (GGC) as the key amino acid in the CNS. In the GGC, glutamine is transferred from astrocytes to neurons by the members of the Sodium-coupled neutral amino acid transporter (SNAT), linker for the activation of T cell (LAT), y+LAT, and apoptosis-associated speck-like protein (ASC) families of transporters, where it acts as an inhibitory or excitatory neurotransmitter (9,10). Walnut peptide (WP), which contains high levels of arginine, aspartic acid, and glutamate, is a good source of GABA supplementation through the glutamate/GABA-glutamine (GGG) cycle (11). Studies have shown that food-derived glutamate increases GABA and glutamate concentrations in the serum and brain tissue (12). Furthermore, walnut protein hydrolysates have been reported to exhibit free radical scavenging and lipid peroxidation activity (13), as well as improving cognitive deficits and memory impairment in mice, while restoring antioxidant enzyme levels and reducing brain inflammatory mediators (14).
In this study, GABA and WP were administered to mice to explore their combined effects on sleep quality. Sleep quality was assessed by measuring sleep latency time, sleep duration and subthreshold dose-fall rate, which are three indicators reflecting the sleep process. Furthermore, the levels of neurotransmitters in the mouse brain were analyzed by ultra-high performance liquid chromatography tandem mass spectrometry analysis (UPLC-MS/MS). We present the following article in accordance with the ARRIVE reporting checklist (available at https://dx.doi.org/10.21037/apm-21-2798).
Methods
Materials
GABA (20%) and walnut oligopeptide Prodemax TM (peptide >60%, protein hydrolysate (<2,000 dalton; >80%) was supplied by Toong Yeuan Food Technology Ltd (Shanghai, China) and Sinphar Tianli Pharmaceutical Co. Ltd. (Hangzhou, China), respectively. Pentobarbital sodium, barbital sodium, GABA, 5-hydroxytryptamine (5-TH), norepinephrine (NeNE), and acetylcholine (Ach) standards were purchased from Sigma-Aldrich (St. Louis, MO, USA). All other reagents were HPLC grade and obtained from Fisher (USA).
Experimental animals
A total of 252 male specific pathogens free (SPF) Bagg’s albino (Balb/c) mice (18–20 g, 8 weeks old) were provided by SiPeiFu Biotechnology Co., Ltd (Beijing). Animals were housed at a temperature of 22±2 °C, humidity of 55%±5%, and a 12-hour light-dark cycle. The mice were given free access to water and food. A protocol was prepared before the study without registration. All animal procedures and protocols were approved by the Fifth Medical Center, Chinese People’s Liberation Army General Hospital (PLA) Animal Care Committee (a professional third party animal agency, IACUC-2019-0016, Beijing, China) and conducted in accordance with the “Guiding Principles in the Care and Use of Animals” published by the American Physiological Society.
Intervention
The mice were randomly divided into three groups (n=84) for the pentobarbital-prolonged sleep test, the pentobarbital-threshold sleep test, and the barbital-induced sleep test. For each type of sleep test, the animals were randomly divided into 6 subgroups and treated by gavage (volume 20 mL/kg) for 30 days as follows: control group (saline), GABA group (102.25 mg/kg GABA), WP group (102.25 mg/kg WP), GABA/WP mixture dose 1 group (33.95 mg/kg GABA,102.25 mg/kg WP), GABA/WP mixture dose 2 group (GABA, 102.25 mg/kg GABA, 102.25 mg/kg WP), and GABA/WP mixture dose 3 group (GABA, 306.75 mg/kg GABA, 102.25 mg/kg WP). Sleep status was assessed within 1 hour after oral administration.
Direct sleep experiment
The direct sleep experiments were designed to exclude the direct hypnotic effects of GABA and WP. After gavage on the 30th day of the experiment, mice were observed for 1 hour. Sleep was assessed based on the disappearance of the righting reflex. If the mouse cannot turn upright within 1 minute, this is determined as the absence of the turn right reflex and the mouse is considered to be sleeping. If the righting reflex is detected, the animal is considered to be awake. The period between the loss of the righting reflex to recovery of the righting reflex recovery is determined to be the total sleep time. The number of sleeping animals and the sleep time of the animals were recorded.
Pentobarbital-prolonged sleep test
Preliminary tests confirmed that 50 mg/kg of pentobarbital sodium leads to a certain sleep time in mice. To determine if GABA/WP can prolong sleep, mice were given GABA, WP, or the combination of GABA/WP orally. After 30 minutes, mice were treated with sodium pentobarbital intraperitoneally (50 mg/kg) and the sleep time of mice was assessed. Sleep-deprived mice were eliminated from the experiment after injection of pentobarbital sodium.
Pentobarbital-threshold sleep test
Preliminary tests confirmed that a sub-threshold dose of sodium pentobarbital (25 mg/kg) can induce sleep in 10–20% of mice. To determine whether GABA or WP could promote sleep, mice were administered oral GABA, WP, or a combination of GABA/WP. After 220 minutes, mice were given intraperitoneal injections of a sub-threshold amount of sodium pentobarbital (25 mg/kg). The total number of mice sleeping within 30 minutes was recorded and the rate was calculated.
Barbital-induced sleep test
We investigated whether GABA could shorten the time between sodium barbiturate intervention and falling asleep, that is, the sleep latency. Mice were administered GABA, WP, or both GABA and WP orally. After 30 minutes, sodium barbital (260 mg/kg) was administered intraperitoneally in each mouse. The time taken from sodium barbital injection to the loss of righting reflex (sleep latency) was determined. Mice with righting reflex after the injection were excluded from the data. All mice were sacrificed and the brain tissue was collected and stored at −80 °C further experiments.
Sample processing
A 1:1 methanol/water solution was added to the mice brain tissue. After centrifugation for 5 minutes at 13,000 rpm, 150 µL precipitant (Methanol/Acetonitrile =1:1) was added to 50 µL of homogenate supernatant and centrifugation for 10 minutes. The levels of GABA, Ach, 5-HT, and NE were detected with LC-MS.
LC-MS/MS chromatography
LC-MS/MS analysis was performed with Water Acquity ultra-high performance liquid chromatography (UPLC) system coupled to a quadrupole mass spectrometer, equipped with a positive working mode electrospray ionization (ESI) source and controlled by the Masslynx software. The separation of Hypersil Gold C-18 (3 microlites, 2.1×100 mm) column (Themo) was as follows: A =0.1% HCOOH, 2.5 mM NFPA in water, and B= ACN. The injection volume with a linear gradient (5–95% B in 5 minutes) was set to 5 µL and the flow rate was set to 0.3 mL/min. The ESI source spray voltage was set to 3.50 kV; capillary temperature was set to 350 °C; sheath gas flow rate was set to 40 arbitrary units; auxiliary gas flow rate was set to 10 arbitrary units; sweep gas flow rate was set to 0 arbitrary units; S lens RF level was set to 50 arbitrary units.
Statistical analysis
Mice which died during the intervention were excluded from the final data analysis. All data was managed in Excel software. All statistical analyses were performed using the SPSS software version 18.0 followed by Analysis of Variance and Chi-square tests and independent t-test was used for comparison between groups.
Results
The effects of GABA and WP on sleep status in mice
The direct effects of GABA or WP on sleep status in mice were assessed. No loss of righting reflex occurred 1 hour after oral administration of GABA, WP, or the GABA/WP mixture, suggesting that these compounds did not directly induce sleep in the mice. The fur and movements of the mice were within the norm during the intervention.
The effects of GABA and WP on prolonged sleep in mice treated with pentobarbital
Mice were treated with GABA, WP, or the combination of the 2, and sleep extension following pentobarbital-induction was assessed (Figure 1). Treatment with GABA or WP alone (102.25 mg/kg) did not significantly affect sleep time compared to control mice (P>0.05). However, a significant difference was observed between mice treated with combined GABA and WP and control mice (P<0.05). Compared with mice treated with GABA alone, mice treated with combined GABA and WP showed increased sleep time (13.54% increase). When increasing doses of GABA was added to a fixed dose of WP, sleep duration in mice showed a dose-dependent increase (21.74%, 20.23%, and 28.10%, respectively). When 33.95 and 306.75 mg/kg GABA was added in combination with WP, the sleep duration was significantly longer than that observed in mice treated with WP alone (P<0.05). When 102.25 mg/kg GABA added in combination with WP, sleep duration was significantly longer than that observed in mice treated with GABA alone (P<0.05). These results demonstrated that the combined administration of GABA and WP exerted a synergistic effect on prolonging sleep time.
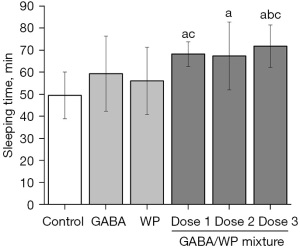
The ability of GABA and WP to promote sleep in mice treated with sub-threshold levels of pentobarbital
The threshold dose of pentobarbital was set to 25 mg/kg which caused 21.42% (3/11) mice to lose the righting reflex. This test was designed to assess the strengthening effect of GABA or WP by comparing the sleep rate of different groups (Figure 2). The sleep rate of the GABA group or the WP group was comparable to the control group. However, with increasing doses of GABA in mice treated with GABA and WP, the number of sleeping mice increased gradually and the sleep rate increased significantly compared to control mice (P<0.05). Increasing doses of GABA in mice treated with combined GABA and WP elevated the sleep rate to 50.00%, 64.28%, and 64.28%, respectively, compared to mice treated with GABA alone (35.71%) or mice treated with WP alone (28.57%) (Figure 2). These results demonstrated that oral administration of combined GABA and WP improved sleep rate when sub-threshold levels of pentobarbital were used in mice, while treatment with GABA alone or WP alone could not enhance the sleep rate.
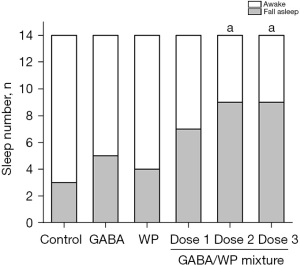
The effects of combined GABA and WP on sleep latency in mice
The barbital-induced sleep test was designed to verify whether GABA, WP, or combined GABA and WP could shorten the time from injection to the loss of righting reflex. Administration of WP alone (102.25 mg/kg) significantly decreased sleep latency time compared with control mice (P<0.05), however, no significant differences were observed in mice treated with GABA alone (Figure 3). Latency time was significantly decreased in mice treated with a combination of GABA and WP compared with control mice (P<0.05). However, combined treatment with GABA and WP did not significantly shorten the sleep latency time compared to animals treated with GABA alone or WP alone. These results suggested that WP plays a major role in shortening the sleep latency time.
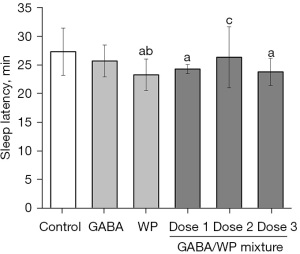
The levels of neurotransmitters in mice brain tissue
The content of neurotransmitters in the brain is closely related to the status of sleep. The levels of GABA in the brain increased with increasing doses of GABA in mice treated with combined GABA and WP. Administration of GABA alone, WP alone, or a combination of GABA and WP significantly increased the content of GABA in mice brain compared with control mice (P<0.05; Figure 4A). The brain GABA contents of mice treated with combined GABA and WP (33.95 and 306.75 mg/kg GABA) were significantly higher than that observed in mice treated with GABA alone or WP alone (P<0.05). Furthermore, in mice treated with the highest dose of GABA and WP (102.25 mg/kg GABA), brain GABA levels were higher than that observed in mice treated with GABA alone.
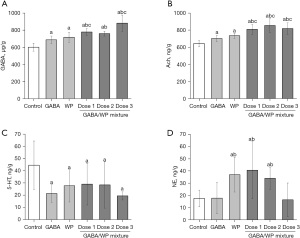
Mice administered GABA alone, WP alone, or the combination of GABA and WP showed significantly increased levels of brain acetylcholine (Ach) compared with control mice (P<0.05; Figure 4B), and the levels observed in mice in the GABA/WP group were higher than that observed in the GABA alone group or the WP alone group (P<0.05).
After oral administration of GABA, WP, or the combination of GABA and WP for 30 days, the levels of serotonin (5-HT) in the brain were significantly decreased compared to that in control mice (P<0.05; Figure 4C). However, there were no significant differences among the treatment groups.
The norepinephrine (NE) levels in the brains of mice treated with WP or the combination of GABA and WP (33.95/102.25 mg/kg and 102.25/102.25 mg/kg) were increased significantly compared with control mice (P<0.05; Figure 4D). However, there was no difference in NE levels between mice in the GABA alone group and mice in the GABA/WP mixture group (306.75/102.25 mg/kg).
Discussion
This study used the pentobarbital and barbital intervention mouse model and the pentobarbital-prolonged sleep test, the pentobarbital-threshold sleep test, and the barbital-induced sleep test to identify the optimal dosing ratio for GABA and WP. The combination of GABA and WP (102.25 mg/kg) reduced sleep latency and prolonged sleep duration in the mouse pentobarbital-induced sleep model. Moreover, the levels of brain GABA and Ach in mice treated with combined GABA and WP were higher than that in the control group and the single intervention groups. This suggested a synergistic relationship between GABA and WP on sleep behavior (P<0.01, compared to control), resulting in significantly reduced sleep latency and increased sleep duration.
Cardiovascular diseases, diabetes, and cancer are closely linked to sleep deprivation (15). Previous studies using the pentobarbital-induced sleep model have confirmed the effects of GABA on sleep enhancement. Additionally, studies have confirmed that WP contains rich bioactive substances and essential amino-acid, among which glutamate and aspartic acid are indispensable excitatory neurotransmitters that are closely related to the formation of learning and memory in mammals (16,17). Metabolomic analyses have revealed that the levels of serum norepinephrine (NE) increased significantly in response to WP intervention and ameliorated cognitive impairments in mice (18).
In this current report, observations for 1 hour after gavage showed that GABA, WP, and the GABA/WP mixture did not directly induce sleep in mice. Both GABA and WP are less effective sleep agents compared with other drugs and are thus suitable as drug replacements in patients with mild symptoms of sleep disorders and in patients who are in remission. The pentobarbital-prolonged sleep model was used to assess whether GABA or WP could prolong pentobarbital-induced sleep in mice. The GABA/WP mixture significantly extended the sleep time compared to control mice, while GABA alone or WP alone had no significant effect. The optimal dose of GABA and WP for prolonging sleep time was determined to be 306.75 mg/kg GABA and 102.25 mg/kg WP. Pentobarbital sodium is metabolized by the liver and excreted from the body. The components with inhibitory effects on liver metabolic enzymes could also achieve the purpose of prolonging sleep time. The pentobarbital threshold sleep model was conducted to rule out the inhibitory effect of the GABA or WP on liver enzymes. In this study, the sleep rate of the GABA/WP mixture (102.25/102.25 mg/kg and 306.75/102.25 mg/kg) was significantly higher than that of control mice and mice treated with GABA alone or WP alone. The barbital-induced sleep model was used to identify the promoting effects of GABA/WP. This study identified WP as the most effective component for shortening sleep latency in mice, with GABA playing an auxiliary role.
The levels of the brain neurotransmitters GABA and Ach showed a dose-dependent elevation with increasing doses of GABA in mice treated with combined GABA and WP (Figure 4A,4B). GABA plays a significant role in the nervous system as a primary mediator of inhibitory neurotransmission and at least 20% of brain neurons are GABAergic (19). In fact, the main clinical insomnia drugs target the GABAA receptor (20). Through the effect of hyperpolarization or depolarization, GABAA receptor activation increases chloride conductance and inhibits neuronal activity. Normal and pathological brain functions including sleep, epilepsy, and various emotions are regulated by GABAA receptors (21). Acetylcholine (Ach) is synthesized and released by cholinergic neurons, and the cholinergic neurons in the brain stem can project to the thalamus, neostriatum, basal forebrain, and other parts. The fibrous tissue and other ascending fibers constitute the uplink reticular activation system, which can regulate respiration and sleep (22). Physiological research has shown that Ach suppresses the spread of excitation in vivo (23) and reduces evoked responses (24), while Ach activation of muscarinic receptors suppresses both intracortical and thalamocortical excitatory connections (25). GABA and Ach were increased after administration of GABA and WP in mice, indicating that the composition could promote the secretion of sleep-promoting neurotransmitters, and the results were consistent with behavioral observation.
Serotonin [5-hydroxytryptamine (5-HT)] alleviates the adverse effects of stress on emotion and behavior via the hippocampus and is thought to be critical for neuroadaptation. Much evidence has demonstrated that the dysfunction of 5-HT neurotransmission in the CNS can cause mood disorders (26). Increasing 5-HT release or inhibiting 5-HT reuptake and increasing 5-HT concentration in the synaptic cleft can lead to anxiety. Conversely, depletion of 5-HT or blocking of 5-HT receptors in the brain can reduce anxiety (27). The decrease of 5-HT in the brain has a calming effect and can promote sleep. As shown in Figure 4C, compared to control mice, the levels of 5-HT in the brain tissue of mice decreased after treatment with GABA, WP, or a combination of the two compounds.
Norepinephrine (NE) is a neurotransmitter that has the chemical structure of catecholamines. NE is synthesized and secreted mainly by post-sympathetic ganglion neurons and adrenergic nerve endings in the brain. The NE content in the brain tissue of mice was increased after treatment with WP. However, the brain NE content did not alter with increasing concentrations of GABA. Thus, the synergistic effect of GABA and WP on NE indexes could not be determined.
This investigation demonstrated that the combined administration of GABA and WP could more efficiently prolong sleep duration, increase sleep rate, and shorten sleep latency compared to administration of either agent alone. Moreover, there was a linear relationship between the levels of GABA and Ach in brain tissues and increasing doses of GABA and WP. The mechanisms of improving sleep status may be related to the regulation of neurotransmitters in brain tissue by the combination of GABA and WP. The factor that affects sleep quality including physiological, psychological, drug, diet and other aspects. As this study was a basic animal research project, the effective dose of GABA and WP in humans requires further investigation. In conclusion, this report suggested that GABA and WP may be used in combination to enhance sleep and may be added in foods as a form of nonpharmacological management in patients with sleep disorders.
Acknowledgments
Funding: None.
Footnote
Reporting Checklist: The authors have completed the ARRIVE reporting checklist. Available at https://dx.doi.org/10.21037/apm-21-2798
Data Sharing Statement: Available at https://dx.doi.org/10.21037/apm-21-2798
Conflicts of Interest: All authors have completed the ICMJE uniform disclosure form (available at https://dx.doi.org/10.21037/apm-21-2798). XC reported he is an employee of Bonnysci International Science & Technology Co., Ltd. XJ, ZZ, JH, HL, JD and WZ reported they are employees of Inner Mongolia Diary Technology Research Institute Co., Ltd. IS, WZ, QC, YM and HF reported they are employees of Yili Innovation Center, Inner Mongolia Yili Industrial Group Co., Ltd. The other authors have no conflicts of interest to declare.
Ethical Statement: The authors are accountable for all aspects of the work and in ensuring that questions related to the accuracy or integrity of any part of the work are appropriately investigated and resolved. A protocol was prepared before the study without registration. The procedure and protocols were approved by the Fifth Medical Center, Chinese People’s Liberation Army General Hospital (PLA) Animal Care Committee (a professional third party animal agency, IACUC-2019-0016, Beijing, China) and conducted in accordance with the “Guiding Principles in the Care and Use of Animals” published by the American Physiological Society.
Open Access Statement: This is an Open Access article distributed in accordance with the Creative Commons Attribution-NonCommercial-NoDerivs 4.0 International License (CC BY-NC-ND 4.0), which permits the non-commercial replication and distribution of the article with the strict proviso that no changes or edits are made and the original work is properly cited (including links to both the formal publication through the relevant DOI and the license). See: https://creativecommons.org/licenses/by-nc-nd/4.0/.
References
- Hodinka BL, Ashley NT. Effect of sleep loss on executive function and plasma corticosterone levels in an arctic-breeding songbird, the Lapland longspur (Calcarius lapponicus). Horm Behav 2020;122:104764 [Crossref] [PubMed]
- Domínguez-Salazar E, Hurtado-Alvarado G, Medina-Flores F, et al. Chronic sleep loss disrupts blood-testis and blood-epididymis barriers, and reduces male fertility. J Sleep Res 2020;29:e12907 [Crossref] [PubMed]
- Ben Simon E, Vallat R, Barnes CM, et al. Sleep Loss and the Socio-Emotional Brain. Trends Cogn Sci 2020;24:435-50. [Crossref] [PubMed]
- Saner NJ, Lee MJ, Pitchford NW, et al. The effect of sleep restriction, with or without high-intensity interval exercise, on myofibrillar protein synthesis in healthy young men. J Physiol 2020;598:1523-36. [Crossref] [PubMed]
- Chang S, Huijun W, Bing Z. Sleep status of adults aged 18-64 in 15 provinces (autonomous regions and municipalities directly under the Central Government) in 2015. Journal of Hygiene Research 2020;49:498-501.
- Goldman-Mellor S, Olfson M, Lidon-Moyano C, et al. Mortality Following Nonfatal Opioid and Sedative/Hypnotic Drug Overdose. Am J Prev Med 2020;59:59-67. [Crossref] [PubMed]
- Sun GQ, Zhang L, Zhang LN, et al. Benzodiazepines or related drugs and risk of pneumonia: A systematic review and meta-analysis. Int J Geriatr Psychiatry 2019;34:513-21. [Crossref] [PubMed]
- Yamatsu A, Yamashita Y, Maru I, et al. The Improvement of Sleep by Oral Intake of GABA and Apocynum venetum Leaf Extract. J Nutr Sci Vitaminol (Tokyo) 2015;61:182-7. [Crossref] [PubMed]
- Qureshi T, Bjørkmo M, Nordengen K, et al. Slc38a1 Conveys Astroglia-Derived Glutamine into GABAergic Interneurons for Neurotransmitter GABA Synthesis. Cells 2020;9:1686. [Crossref] [PubMed]
- Leke R, Schousboe A. The Glutamine Transporters and Their Role in the Glutamate/GABA-Glutamine Cycle. Adv Neurobiol 2016;13:223-57. [Crossref] [PubMed]
- Lee H, Chang MJ, Kim SH. Effects of poly-gamma-glutamic acid on serum and brain concentrations of glutamate and GABA in diet-induced obese rats. Nutr Res Pract 2010;4:23-9. [Crossref] [PubMed]
- Jahanbani R, Ghaffari SM, Salami M, et al. Antioxidant and Anticancer Activities of Walnut (Juglans regia L.) Protein Hydrolysates Using Different Proteases. Plant Foods Hum Nutr 2016;71:402-9. [Crossref] [PubMed]
- Chen N, Yang H, Sun Y, et al. Purification and identification of antioxidant peptides from walnut (Juglans regia L.) protein hydrolysates. Peptides 2012;38:344-9. [Crossref] [PubMed]
- Wang LM, Yi Y, Yao YL, et al. Walnut oil improves spatial memory in rats and increases the expression of acid-sensing ion channel genes Asic2a and Asic4. Food Sci Nutr 2019;7:293-301. [Crossref] [PubMed]
- Baron KG, Reid KJ. Circadian misalignment and health. Int Rev Psychiatry 2014;26:139-54. [Crossref] [PubMed]
- Monti JM, Jantos H. The effects of local microinjection of selective dopamine D1 and D2 receptor agonists and antagonists into the dorsal raphe nucleus on sleep and wakefulness in the rat. Behav Brain Res 2018;339:11-8. [Crossref] [PubMed]
- Topo E, Soricelli A, Di Maio A, et al. Evidence for the involvement of D-aspartic acid in learning and memory of rat. Amino Acids 2010;38:1561-9. [Crossref] [PubMed]
- Wang M, Amakye WK, Guo L, et al. Walnut-Derived Peptide PW5 Ameliorates Cognitive Impairments and Alters Gut Microbiota in APP/PS1 Transgenic Mice. Mol Nutr Food Res 2019;63:e1900326 [Crossref] [PubMed]
- Paredes RG, Agmo A. GABA and behavior: the role of receptor subtypes. Neurosci Biobehav Rev 1992;16:145-70. [Crossref] [PubMed]
- Gottesmann C. GABA mechanisms and sleep. Neuroscience 2002;111:231-9. [Crossref] [PubMed]
- Kalueff A, Nutt DJ. Role of GABA in memory and anxiety. Depress Anxiety 1996-1997;4:100-10. [Crossref] [PubMed]
- Sokhadze G, Campbell PW, Guido W. Postnatal development of cholinergic input to the thalamic reticular nucleus of the mouse. Eur J Neurosci 2019;49:978-89. [Crossref] [PubMed]
- Kitamura A, Marszalec W, Yeh JZ, et al. Effects of halothane and propofol on excitatory and inhibitory synaptic transmission in rat cortical neurons. J Pharmacol Exp Ther 2003;304:162-71. [Crossref] [PubMed]
- Chauvette S, Seigneur J, Timofeev I. Sleep oscillations in the thalamocortical system induce long-term neuronal plasticity. Neuron 2012;75:1105-13. [Crossref] [PubMed]
- Krishnan GP, Chauvette S, Shamie I, et al. Cellular and neurochemical basis of sleep stages in the thalamocortical network. Elife 2016;5:18607. [Crossref] [PubMed]
- Lesch KP, Araragi N, Waider J, et al. Targeting brain serotonin synthesis: insights into neurodevelopmental disorders with long-term outcomes related to negative emotionality, aggression and antisocial behaviour. Philos Trans R Soc Lond B Biol Sci 2012;367:2426-43. [Crossref] [PubMed]
- Haleem DJ. Behavioral deficits and exaggerated feedback control over raphe-hippocampal serotonin neurotransmission in restrained rats. Pharmacol Rep 2011;63:888-97. [Crossref] [PubMed]
(English Language Editor: J. Teoh)