All-trans retinoic acid inhibits epithelial-to-mesenchymal transition (EMT) through the down-regulation of IL-6 in endometriosis
Introduction
Endometriosis (EMs) is a non-malignant, but potential metastatic, reproductive disorder that manifests as inflammatory endometriotic implants growing outside of the uterus, mainly occurring in women of childbearing age. The incidence of chronic pelvic pain and infertility in patients is as high as 45%, and around 10–12% of women worldwide suffer from this disease (1,2). However, its defined etiology and pathogenic mechanism remain obscure, and the current clinical therapeutic effect is dismal. Multiple hypotheses have been proposed to elucidate the initiation and development of EMs (3). Among, the most commonly accepted hypothesis is “Sampson’s theory”, postulating that endometrial cells are deposited into the peritoneal cavity via retrograde menstruation (4). However, nearly 90% of women experience retrograde menstruation while endometriotic lesions are observed in only 10%, suggesting that additional factors trigger the incidence (5). In the 1970s, Prianishnikov presented the concept of the “endometrial stem cell”, positing that a small amount of endometrial stem cells, which reside in the endometrial functional layer, possess the characteristics of mesenchymal stem cells (6). It has been suggested that endometrial stem cells participate in EMs pathogenesis due to their features of cell regeneration, proliferation, and high developmental plasticity (7-9).
Epithelial-to-mesenchymal transition (EMT) is an established mechanism that drives the progression of the in-situ tumor towards metastasis through affecting cell morphology and polarization, which causes a disruption of the integrity of the intercellular cohesion (10). Although EMs is acknowledged to be a benign gynecological disorder, it has similar characteristics to malignant tumors such as cell infiltration, migration, and invasion, thereby infiltrating and developing new ectopic lesions (11). Recently, it has been verified that EMT plays a critical role in the initial formation and evolution of EMs (12,13). In addition, genic transition changes shown in endometriotic cell phenotypes were involved in the processes of mesenchymal-epithelial metastasis (MET) and EMT, which also strengthened the association between EMs and EMT (14,15).
Besides, emerging evidence has demonstrated that the abnormal immune response in the peritoneal microenvironment emerges as a hinge for endometriotic cells which escape from the immunosurveillance of the peritoneal cavity (16). Endometriotic implant growth was accompanied by the recruitment of various immune cells and severe inflammation caused by increased pro-inflammatory cytokines, growth factors, and angiogenesis. There was solid evidence that the function of almost all types of immune cells was abnormal in women with EMs (17). Amongst all the inflammatory cytokines, interleukin-6 (IL-6), involved in many biological processes including immune processes (T- and B-cell activation and macrophage differentiation), bone metabolism, arthritis, tumors, and reproduction (18), has attracted significant attention in EMs research. Prior studies revealed that endometriotic cells were resistant to IL-6 (19-21), and its secretion was significantly up-regulated (22,23). Additional reports have also described a significant increase in IL-6 levels both in the peritoneal fluid and serum of EMs patients (23,24). Furthermore, a previous study uncovered that the increased expression of IL-6 enhanced the migration and invasion abilities of endometriotic stromal cells (25). Considering that an increased level of IL-6 is associated with tumor development and progression via prompting EMT in a variety of cancers, we propose a hypothesis that abnormal IL-6 stimulates EMs progression by improving the EMT process.
Additionally, a link between abnormal retinol metabolism and EMs has recently been reported. Some researchers pointed out that abnormal retinoic acid (RA) signal transduction pathways had a certain effect on endometriotic stromal cell survival, and there was growing evidence that treatments for the path of retinal metabolism could be therapeutic for EMs (26-28). As a small-molecule derivative of vitamin A, all-trans retinoic acid (ATRA), a well-known anti-leukemic drug, has been clinically used in the treatment of certain tumor types. For the past few years, its importance in EMs studies has amplified (29,30). IL-6 level has been found to be inhibited after RA treatment (31). Consequently, we assume that ATRA inhibits the EMT capability of endometriotic cells. The current study attempts to explore whether ATRA inhibits the EMT of endometriotic stromal stem cells by targeting IL-6.
We present the following article in accordance with the MDAR reporting checklist (available at https://dx.doi.org/10.21037/apm-21-2175).
Methods
Cell isolation and culture
Endometriotic samples were obtained from the ovarian endometrioma of patients who underwent laparoscopic surgery for EMs, while control endometrial samples were obtained from the endometrium of patients who underwent laparoscopic surgery without EMs. Only patients who had not taken exogenous hormones for 3 months before surgery were included in the research. All procedures performed in this study involving human participants were in accordance with the Declaration of Helsinki (as revised in 2013). Informed written consent was acquired from each patient, and the Second Affiliated Hospital of Wenzhou Medical University provided ethical approval (No. 2014-28).
Hepes-buffered Dulbecco’s modified Eagle’s medium (DMEM)/Ham’s F-12 (DMEM-F12) (Gibco, USA) containing 5% fetal bovine serum (FBS) (Gibco, USA) and 1% antibiotics (Gibco, USA) (32) was used to collect endometrial and endometriotic samples. After dissociation, 300 µg/mL of collagenase type 3 (Worthington Biochemical Corporation, USA) and 40 µg/mL of deoxyribonuclease type I (Roche Diagnostics, Germany) were added to the dissociated tissue to digest. After digestion and filtration, purified stromal cell suspensions were acquired and subsequently cultured in DMEM-F12 medium supplemented with 10% FBS and 1% antibiotics. The culture medium was replaced every 3–4 days.
Low-density cell clonogenicity
Low-density endometrial and endometriotic stromal cells were inoculated onto fibronectin (10 mg/mL)-coated plates (Thermo Fisher Scientific, USA) to test cell clonogenicity. Briefly, cells at the 3rd passage were treated with 0.05% trypsin/0.2% EDTA (Gibco, USA) to obtain single-cell suspensions, adjusted to a clonal density of 500 cells/cm2, and seeded in triplicate in plates (32). Then, these cultures were incubated for 15 days and the cells were microscopically controlled daily to ensure the single-cell origin of the clusters. The clusters were then rinsed 3 times using phosphate buffered saline (PBS, pH =7.2) and stained with Giemsa solution at the end of the incubation period. Clusters of cells which contained more than 50 cells were considered as clones or colony forming units (CFUs). To determine cloning efficiency (CE), the following formula was applied: cloning efficiency (CE%) = (number of clones/number of cells seeded) × 100.
Self-renewal of stromal cells was assessed by serial cloning of individual large and small clones. Cells from individual clones were reseeded at clonal density to generate secondary clones. Two types of stromal clones were harvested per primary clone. Each clone was sub-cloned into duplicate culture plates until the 5th passage if more than 600 cells could be harvested.
Immunocytochemistry (ICC)
Endometrial and endometriotic clonogenic stromal cells generated from the large stromal CFUs were inoculated at 5,000–10,000 cells/cm2 onto 25-mm Thermanox coverslips (Nalge Nunc International, USA) in 6-well plates. After culturing for nearly 3 days, these coverslips were rinsed with PBS and then fixed with 10% formalin (Sigma-Aldrich, USA) before being treated with 0.3% hydrogen peroxide (Merck, Germany) to quench the endogenous peroxidase, followed by 10% blocking serum (Sigma-Aldrich, USA). Then, the primary antibodies were diluted with 10% serum and incubated overnight at 4 °C. The primary antibodies were vimentin (Abcam, USA), CD90 (Abcam, USA), and CD146 (Abcam, USA). Subsequently, the coverslips were rinsed and incubated with the corresponding secondary antibody for 1 hour, followed by incubation with the ABC regent (Vector Laboratories, USA) for 30 min. Then, positive immunoreactivities were visualized after incubation with DAB chromogen (Sigma-Aldrich, USA).
In vitro differentiation
To assess multipotency, the cells of large endometriotic stromal CFUs were induced to undergo osteogenic, chondrogenic, and adipogenic differentiation. When cells reached more than 80% confluence, large endometriotic stromal CFUs were isolated, expanded in culture medium, and seeded at 5,000 cells/cm2. Subsequently, they were cultured in differentiation induction medium for 4 weeks using standard methods. Meanwhile, undifferentiated control cells were concurrently cultured in low serum medium (DMEM-F12/1%FBS/1% antibiotics). Osteogenic differentiation was detected using Alizarin Red, chondrogenic differentiation was detected using Alcian Blue, and adipogenic differentiation was detected using Oil Red O.
Transwell assay
Upon reaching 80–90% confluence with treatment for 48 hours, the cells of large endometriotic stromal CFUs were digested and assessed for migration and invasion abilities by the transwell assay, which are the main manifestations of EMT as previously described (33). For the migration and invasion assay, cells were seeded into wells averagely and incubated for 24 h. Then, these cells were randomly counted in 5 fields of each chamber. Then the average cell numbers in 3 duplicate wells for each patient sample were calculated statistically.
Quantitative real-time polymerase chain reaction (RT-qPCR)
Total RNA was extracted from each treatment group of large endometriotic stromal CFUs with TRIzol reagent (Invitrogen, USA). A spectrophotometer (Thermo Fisher Scientific, USA) was used to assess the quality of the RNA. SuperScript First-Strand Synthesis System (Invitrogen, USA) was used to convert RNA into cDNA. RT-qPCR was performed in triplicate using the FastStart Universal SYBR Green Master (Roche, Germany). The gene-specific primers used for the present study were as follows: PCNA: sense 5'-GGCCGAAGATAACGCGGATAC-3', antisense 5'-GGCATATACGTGCAAATTCACCAG-3'; Caspase-3: sense 5'-CGTCGATTTTTGTGATGCTCGTCAG-3', antisense 5'-GAAGCATTTATCAGGGTTATTGTCTCATG-3'; IL-6: sense 5'-GGACAUGACAACUCAUCUCTT-3', antisense 5'-GAGAUGAGUUGUCAUGUCCTG-3'; Vimentin: sense 5'-CCAGGCAAAGCAGGAGTC-3', antisense 5'-GGGTATCAACCAGAGGGAGT-3'; N-cadherin: sense 5'-ATGTGCCGGATAGCGGGA-3', antisense 5'-TCAGTCATCACCACCACCAT-3'; Snail: Sense 5'-GCCUUCAACUGCAAAUACUTT-3', antisense 5'-AGUAUUUGCAGUUGAAGGCTT-3'; Slug: sense 5'-CAAGGACACATTAGAACTCACAC-3', antisense 5'-CTACACAGCAGCCAGATTC-3'; Twist: sense 5'-TCCTCTATGGGGTCGT-3', antisense 5'-ACCACCGCATCTCTAC-3'.
The transcript level for each specific gene was normalized to the level of the β-actin gene, and the comparative threshold cycle (Ct) method (2–ΔΔCt) was used to calculate.
Western blot
Total protein was extracted from each treatment group of large endometriotic stromal CFUs for the western blot, as previously described (33). The protein samples were loaded on 8–12% sodium dodecyl sulfate polyacrylamide gels equally to carry out electrophoresis. The primary antibodies were anti-IL-6 (Abcam, USA), anti-N-cadherin (Abcam, USA), anti-Vimentin (Abcam, USA), anti-Snail (Abcam, USA), anti-Slug (Abcam, USA), anti-Twist (Abcam, USA), anti-PCNA (Abcam, USA), and anti-Caspase-3 (Abcam, USA). GAPDH was used as the loading control. The results were quantified by densitometry by using ImageJ software (NIH, USA).
ELISA assay
According to the manufacturer’s instructions, the Human IL-6 Quantikine ELISA Kit (R&D Systems, USA) was used to measure the IL-6 level in the liquid culture supernatants. For each treatment group, the cell number was basically the same in each well, and culture medium was collected.
Statistical analysis
GraphPad Prism Version 7 was applied for statistical analysis. The data are shown as the mean of mean ± standard error. The differences between groups and among groups were analyzed by student t-test and one-way analysis of variance respectively. P value <0.05 was considered significant statistically.
Results
Primary culture of endometrial and endometriotic stromal cells and cell clonogenicity
Both endometrial stromal cells from the endometrium and endometriotic stromal cells from ovarian endometrioma were characteristically flat and spindle shaped. The low-density clonogenicity assay was conducted under microscopy during the whole culture process. The purified individual endometrial and endometriotic stromal cells both attached within 24 hours, and small clusters of 3 to 4 flattened fibroblasts began to form on the 4th day. During the first week of culture, 2 clone types appeared in different sizes. Large stromal clone was defined as comprising more than 4,000 cells with a dense center of tightly-packed cells, while small stromal clone comprised less than 4,000 loosely-packed cells (32). Around day 11 to day 12, small clones ceased proliferation and maintained their sizes, while large clones kept increasing dramatically and contained as many as 15,000 cells which could form CFUs until day 15 (Figure 1A). Then, the CE between endometrial and endometriotic stromal clones showed no statistically significant difference regardless of the large or small size (Figure 1B).
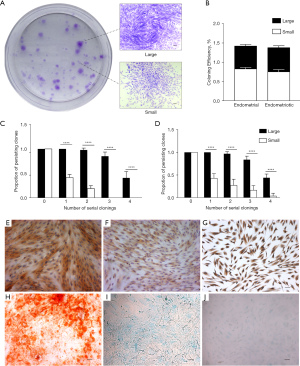
Next, the serial clonogenicity assay was performed to evaluate the self-renewal capacity of stromal cells. The numbers for serial cloning of large and small clones or CFUs were shown as serial cultivation. Large stromal clones could produce large and small clones until passage 4, while small clones at all times produced no large clones and eventually failed to form clones, with loosely dispersed cells attached on the culture plate (Figure 1C,1D). These results suggested that the large stromal clones or CFUs, not the small stromal clones, were endowed with a high capacity for self-renewal.
Expression of stem cell markers of large endometriotic stromal CFUs
Heretofore, no specific marker for endometrial/endometriotic stem/progenitor cells has been identified. In prior studies, cultured endometrial cells, which expressed the typical markers previously used to label bone marrow stem cells (34,35), were considered as endometrial stem cells. In our assay, large endometriotic stromal CFUs were not only positive for the stromal marker vimentin (Figure 1E), but also positive for the stem cell markers CD90 (Figure 1F) and CD146 (Figure 1G), confirming that large endometriotic stromal CFUs were endometriotic stem cell enrichment groups, which was in accordance with previous experiment (36).
Differentiation of large endometriotic stromal CFUs
The mesenchymal features of endometrial stem/progenitor cells have been clearly demonstrated through the detection of in-vitro chondrogenic, osteogenic, and adipogenic differentiation (34,36-38). By cultivating large endometriotic stromal CFUs in osteogenic, chondrogenic, and adipogenic inducing medium for 4 weeks, the potential of large endometriotic stromal CFUs to differentiate toward mesenchymal cell lineages was examined in our study. For osteogenic differentiation, cells cultured in osteogenic inducing medium showed positive staining for Alizarin Red (Figure 1H). For chondrogenic differentiation, cells cultured in chondrogenic inducing medium showed positive staining for Alcian Blue (Figure 1I). For adipogenic differentiation, cells cultured in adipogenic inducing medium showed positive staining for Oil Red O (Figure 1J). Considering these findings, we could reasonably regard the large endometriotic stromal CFUs as a set of endometriotic stem cells.
Expression and effect of IL-6 in endometriotic stem cells
We aimed at the large endometriotic stromal CFUs in the next step of preliminarily discussing the relationship between endometriotic stem cells and EMs pathogenesis. Herein, we attempted to examine the role of IL-6 in the induction of the EMT process in the large endometriotic stromal CFUs. The large endometrial and endometriotic stromal CFUs were selected to examine the IL-6 level and EMT status. Compared with the large endometrial stromal CFUs, the large endometriotic stromal CFUs maintained a higher expression level of IL-6 (Figure 2A), along with a greater ability to invade in the transwell assay (Figure 2B,2C). Further, the large endometriotic stromal CFUs possessed higher levels of IL-6, PCNA, and the mesenchymal-related markers N-cadherin, snail, slug, and twist, showing a much stronger tendency of EMT and proliferation (Figure 2D-2F), indicating a relevant link between the upregulation of IL-6 and the invasive growth of endometriotic stromal stem cells. Considering that IL-6 may act as a functional regulator in EMT-like endometriotic stem cells, we presumed that IL-6 was a potential therapeutic target for EMs as aiming to reverse the EMT state in the large endometriotic stromal CFUs through inhibiting IL-6.
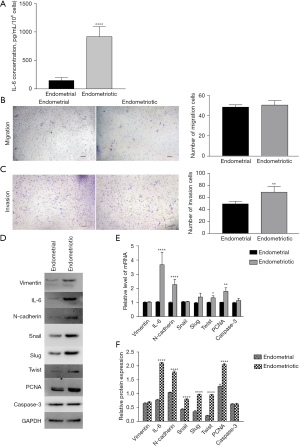
ATRA inhibited the EMT of endometriotic stem cells and IL-6 expression
Given that endometriotic stromal stem cells maintain a stronger ability for EMT, and RA has been reported to inhibit EMT in tumor development (39,40), we chose ATRA and AM580 [a selective retinoic acid receptor alpha (RARα) agonist] to examine the inhibitory effect of ATRA on IL-6 and EMT ability in the large endometriotic stromal CFUs. Our results revealed that ATRA inhibited the IL-6 level of the large endometriotic stromal CFUs in a dose-dependent manner after 48-hour treatment, with significant inhibition at 10−6 M (Figure 3A,3B). Then, we examined the effect of ATRA on the migration and invasion abilities of the large endometriotic stromal CFUs. As expected, the migration and invasion abilities of the large endometriotic stromal CFUs were both significantly decreased after treatment with ATRA for 48 h (Figure 3C,3D).
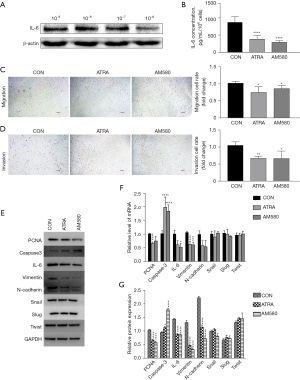
To explore whether ATRA reduced the migration and invasion abilities by preventing EMT, we measured the expression of EMT markers in the large endometriotic stromal CFUs. The proliferation ability, vimentin, and N-cadherin were decreased significantly, accompanied by decreased IL-6 expression after treatment with ATRA or AM580 for 48 h (Figure 3E-3G). Therefore, we confirmed the efficient inhibitory effect of ATRA on the EMT of the large endometriotic stromal CFUs.
ATRA inhibited the EMT of endometriotic stem cells by targeting IL-6
To investigate the role of IL-6 in the EMT status of the large endometriotic stromal CFUs and ATRA inhibition, additional IL-6 was added. After being cultured in IL-6-supplemented ATRA-conditioned medium, IL-6 expression of the large endometriotic stromal CFUs increased, and the migration and invasion abilities were also increased reversely (Figure 4A-4C). In addition, compared with ATRA treatment, the levels of vimentin and N-cadherin were increased after exposure to exogenous IL-6 (Figure 4D-4F). These data confirmed that ATRA inhibited EMT-related processes by effectively decreasing IL-6.
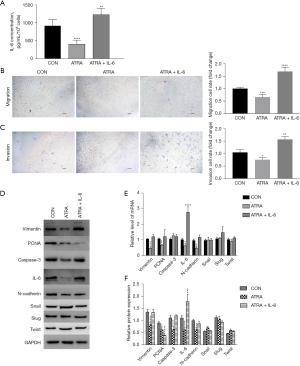
Overall, our findings suggested that IL-6 mediated the EMT of large endometriotic stromal CFUs to promote EMs progression, while ATRA could inhibit this process specifically.
Discussion
This study revealed a correlation between IL-6 and EMT in EMs, which demonstrated that elevated IL-6 stimulated the EMT process in endometriotic stem cell enrichment sets, whereas ATRA specifically inhibited this process.
In 2004, Chan et al. demonstrated that human endometrial epithelial and stromal cell suspensions could form CFUs (32). Nearly 15 years later, several different types of stem/progenitor cells have been gradually detected in the human endometrium. Stem/progenitor cells have also been identified in the ectopic endometria and have manifested a similar ability to form colonies (41). Incumbent data linked the observation of endometriotic stem cells to Sampson’MAOs theory (42). Once these cells from the menstrual debris reached the pelvic cavity or other ectopic sites, their stem-cell characteristics, which included high self-renewal ability, resistance to hormonal therapy, and regeneration, promoted the formation of endometriotic lesions. This is able to account for the disparity between the EMs morbidity and retrograde menstruation rate. Furthermore, researchers proposed that the severity of EMs depended on the “stemness” degree of the endometriotic cells. In addition, clonogenic epithelial cells and stromal cells from ectopic endometria in EMs patients had higher proliferative and invasive potential (8,43). Consequently, evidence supporting the endometrial stem cell theory is growing (44,45). In our study, the large stromal CFUs from the endometrium and ovarian endometrioma both possessed higher renewal and clonogenic capacity. Then, the large endometriotic stromal CFUs were confirmed to be representative of endometriotic stromal stem cells, which could differentiate into osteogenesis, chondrogenesis, and adipogenesis, and expressed the stem cell markers CD90 and CD146.
A prior study demonstrated an increased level of IL-6 in endometriotic cells, revealing that endometriotic cells with immune dysfunction played a part in the pathogenesis of EMs (46). The promotion of mammosphere formation, stem cell self-renewal, cancer cell stemness, and stem cell recruitment (47,48) by IL-6 has also been shown, supporting the view that IL-6 could directly act on stem cell function. IL-6 has been proven to be associated with tumor angiogenesis, proliferation, migration, metastasis, and chemotherapy resistance in many types of cancers (49-52). Subsequent publications suggested that IL-6 could promote the EMT process (48,53,54) by inhibiting the expression of E-cadherin and inducing vimentin, N-cadherin, snail, and twist expression in breast adenocarcinoma cells (53), in conjunction with epidermal growth factor (EGF) through activating JNK2/STAT3 in ovarian cancer (55), and inducing hypoxia associated with the EMT of small cell lung cancer (56), thereby improving the migration and invasion abilities of cells. Additionally, IL-6 has been shown to promote the migration and invasion capabilities of endometriotic cells (57,58). Notably, our data further demonstrated that an enrichment set of endometriotic stem cells manifested a stronger EMT potency, accompanied by higher IL-6 expression, providing powerful evidence that EMs originated from endometriotic stem cells which were stimulated by abnormal IL-6.
Consistently, it has been proven that targeting IL-6 could decrease the invasion and metastasis abilities of pancreatic cancer-associated fibroblast cells and lung cancer cells via repressing the EMT process (31,59). Next, our study elucidated the therapeutic effect of ATRA on EMs by revealing that ATRA inhibited EMT through decreasing IL-6 in endometriotic stem cells. In the EMs research field, many studies have found that retinol metabolism related to abnormal immunological changes in inflammatory processes could contribute to EMs development and/or progression (60,61). RA has been found to have potential in suppressing EMs via diverse pathways, such up-regulating gap junctional intercellular communication and connexin-43 (62) and suppressing IL-6 (63) in human endometrial stromal cells. Besides, a transcriptome analysis performed in ATRA-treated endometriotic stromal cells showed many influenced genes associated with the negative regulation in cellular proliferation were up-regulated, and the 17-beta-dehydrogenase 2 gene, which converts estradiol into estrone, was decreased (64). In an experimental mouse model, ATRA treatment reduced the endometriotic implant growth caused by decreased IL-6 and MCP-1 production, and enhanced peritoneal macrophage differentiation, showing an inhibitory effect on EMs development (65). In addition, fenretinide (a synthetic retinoid analogue) treatment induced endometriotic stromal cell apoptosis and increased STRA6 expression, thereby potentially reversing the retinoid metabolism dysfunction in EMs (66). Lu et al. further demonstrated that RA could up-regulate beclin1, a fundamental protein involved in autophagy, to suppress endometriotic stromal cell growth (67). As well, RA displayed reproductive protective capacity in a rat EMs model (68). In our in-vitro study, down-regulation of IL-6 by ATRA resulted in decreased endometriotic stem cell proliferation and EMT tendency. Nevertheless, the role of IL-6 in the microenvironment of EMs needs further in-depth study to fully evaluate the therapeutic potential.
Our present study provides a new strategy for the use of ATRA in the non-hormonal treatment of EMs, especially aimed at endometriotic stem cells. New combinatorial therapeutic approaches may explore the possibility of harnessing the anti-invasive properties of ATRA while blocking stemness-associated characteristics using a second drug. Nevertheless, due to the small clinical sample size, this study is limited. Studies with a larger sample size and in vivo studies are required to validate our results, and more investigations are also needed to probe into the exact molecular mechanisms involved.
Acknowledgments
Funding: The research was supported by grants from the Natural Science Foundation of China (No. 81401184), the scientific research of Wenzhou (No. 2018Y0759), the Natural Science Foundation of Zhejiang Province (No. LY20H040005), the Medical and Health Research Project of Zhejiang Province of China (No. 2017KY479).
Footnote
Reporting Checklist: The authors have completed the MDAR reporting checklist. Available at https://dx.doi.org/10.21037/apm-21-2175
Data Sharing Statement: Available at https://dx.doi.org/10.21037/apm-21-2175
Conflicts of Interest: All authors have completed the ICMJE uniform disclosure form (available at https://dx.doi.org/10.21037/apm-21-2175). The authors have no conflicts of interest to declare.
Ethical Statement: The authors are accountable for all aspects of the work in ensuring that questions related to the accuracy or integrity of any part of the work are appropriately investigated and resolved. All procedures performed in this study involving human participants were in accordance with the Declaration of Helsinki (as revised in 2013). Written informed consent was obtained from each patient and the Second Affiliated Hospital of Wenzhou Medical University provided ethical approval (No. 2014-28).
Open Access Statement: This is an Open Access article distributed in accordance with the Creative Commons Attribution-NonCommercial-NoDerivs 4.0 International License (CC BY-NC-ND 4.0), which permits the non-commercial replication and distribution of the article with the strict proviso that no changes or edits are made and the original work is properly cited (including links to both the formal publication through the relevant DOI and the license). See: https://creativecommons.org/licenses/by-nc-nd/4.0/.
References
- Wang CY. Coping with endometriosis. Lancet 2004;364:1800. [Crossref] [PubMed]
- Brunty S, Mitchell B, Bou-Zgheib N, et al. Endometriosis and ovarian cancer risk, an epigenetic connection. Ann Transl Med 2020;8:1715. [Crossref] [PubMed]
- Wang Y, Nicholes K, Shih IM. The Origin and Pathogenesis of Endometriosis. Annu Rev Pathol 2020;15:71-95. [Crossref] [PubMed]
- Sampson JA. Peritoneal endometriosis due to the menstrual dissemination of endometrial tissue into the peritoneal cavity. Am J Obstet Gynecol 1927;14:422-69. [Crossref]
- Halme J, Hammond MG, Hulka JF, et al. Retrograde menstruation in healthy women and in patients with endometriosis. Obstet Gynecol 1984;64:151-4. [PubMed]
- Cousins FL, Xiao L, Gargett CE. Adult Stem Cells in the Pathogenesis and Treatment of Endometriosis. Journal of Endometriosis and Pelvic Pain Disorders 2018;9:223-31. [Crossref]
- Gargett CE. Uterine stem cells: what is the evidence? Hum Reprod Update 2007;13:87-101. [Crossref] [PubMed]
- Gargett CE, Schwab KE, Brosens JJ, et al. Potential role of endometrial stem/progenitor cells in the pathogenesis of early-onset endometriosis. Mol Hum Reprod 2014;20:591-8. [Crossref] [PubMed]
- Götte M, Wolf M, Staebler A, et al. Increased expression of the adult stem cell marker Musashi-1 in endometriosis and endometrial carcinoma. J Pathol 2008;215:317-29. [Crossref] [PubMed]
- Voulgari A, Pintzas A. Epithelial-mesenchymal transition in cancer metastasis: mechanisms, markers and strategies to overcome drug resistance in the clinic. Biochim Biophys Acta 2009;1796:75-90. [PubMed]
- Starzinski-Powitz A, Handrow-Metzmacher H, Kotzian S. The putative role of cell adhesion molecules in endometriosis: can we learn from tumour metastasis? Mol Med Today 1999;5:304-9. [Crossref] [PubMed]
- Konrad L, Gronbach J, Horné F, et al. Similar Characteristics of Endometrial and Endometriotic Epithelial Cells. Reprod Sci 2019;26:49-59. [Crossref] [PubMed]
- Bilyk O, Coatham M, Jewer M, et al. Epithelial-to-Mesenchymal Transition in the Female Reproductive Tract: From Normal Functioning to Disease Pathology. Front Oncol 2017;7:145. [Crossref] [PubMed]
- Bartley J, Jülicher A, Hotz B, et al. Epithelial to mesenchymal transition (EMT) seems to be regulated differently in endometriosis and the endometrium. Arch Gynecol Obstet 2014;289:871-81. [Crossref] [PubMed]
- Proestling K, Birner P, Gamperl S, et al. Enhanced epithelial to mesenchymal transition (EMT) and upregulated MYC in ectopic lesions contribute independently to endometriosis. Reprod Biol Endocrinol 2015;13:75. [Crossref] [PubMed]
- Podgaec S, Abrao MS, Dias JA Jr, et al. Endometriosis: an inflammatory disease with a Th2 immune response component. Hum Reprod 2007;22:1373-9. [Crossref] [PubMed]
- Paul Dmowski W, Braun DP. Immunology of endometriosis. Best Pract Res Clin Obstet Gynaecol 2004;18:245-63. [Crossref] [PubMed]
- Akira S, Taga T, Kishimoto T. Interleukin-6 in biology and medicine. Adv Immunol 1993;54:1-78. [Crossref] [PubMed]
- Rier SE, Zarmakoupis PN, Hu X, et al. Dysregulation of interleukin-6 responses in ectopic endometrial stromal cells: correlation with decreased soluble receptor levels in peritoneal fluid of women with endometriosis. J Clin Endocrinol Metab 1995;80:1431-7. [PubMed]
- Tabibzadeh SS, Santhanam U, Sehgal PB, et al. Cytokine-induced production of IFN-beta 2/IL-6 by freshly explanted human endometrial stromal cells. Modulation by estradiol-17 beta. J Immunol 1989;142:3134-9. [PubMed]
- Eisermann J, Gast MJ, Pineda J, et al. Tumor necrosis factor in peritoneal fluid of women undergoing laparoscopic surgery. Fertil Steril 1988;50:573-9. [Crossref] [PubMed]
- Tseng JF, Ryan IP, Milam TD, et al. Interleukin-6 secretion in vitro is up-regulated in ectopic and eutopic endometrial stromal cells from women with endometriosis. J Clin Endocrinol Metab 1996;81:1118-22. [PubMed]
- Punnonen J, Teisala K, Ranta H, et al. Increased levels of interleukin-6 and interleukin-10 in the peritoneal fluid of patients with endometriosis. Am J Obstet Gynecol 1996;174:1522-6. [Crossref] [PubMed]
- Harada T, Yoshioka H, Yoshida S, et al. Increased interleukin-6 levels in peritoneal fluid of infertile patients with active endometriosis. Am J Obstet Gynecol 1997;176:593-7. [Crossref] [PubMed]
- Delbandi AA, Mahmoudi M, Shervin A, et al. Eutopic and ectopic stromal cells from patients with endometriosis exhibit differential invasive, adhesive, and proliferative behavior. Fertil Steril 2013;100:761-9. [Crossref] [PubMed]
- Bruner-Tran KL, Osteen KG, Duleba AJ. Simvastatin protects against the development of endometriosis in a nude mouse model. J Clin Endocrinol Metab 2009;94:2489-94. [Crossref] [PubMed]
- Oktem M, Esinler I, Eroglu D, et al. High-dose atorvastatin causes regression of endometriotic implants: a rat model. Hum Reprod 2007;22:1474-80. [Crossref] [PubMed]
- Yilmaz B, Ozat M, Kilic S, et al. Atorvastatin causes regression of endometriotic implants in a rat model. Reprod Biomed Online 2010;20:291-9. [Crossref] [PubMed]
- Alizadeh F, Bolhassani A, Khavari A, et al. Retinoids and their biological effects against cancer. Int Immunopharmacol 2014;18:43-9. [Crossref] [PubMed]
- Peinemann F, van Dalen EC, Tushabe DA, et al. Retinoic acid post consolidation therapy for high-risk neuroblastoma patients treated with autologous hematopoietic stem cell transplantation. Cochrane Database Syst Rev 2015;1:CD010685 [PubMed]
- Guan J, Zhang H, Wen Z, et al. Retinoic acid inhibits pancreatic cancer cell migration and EMT through the downregulation of IL-6 in cancer associated fibroblast cells. Cancer Lett 2014;345:132-9. [Crossref] [PubMed]
- Chan RW, Schwab KE, Gargett CE. Clonogenicity of human endometrial epithelial and stromal cells. Biol Reprod 2004;70:1738-50. [Crossref] [PubMed]
- Xu X, Zheng Q, Zhang Z, et al. Periostin Enhances Migration, Invasion, and Adhesion of Human Endometrial Stromal Cells Through Integrin-Linked Kinase 1/Akt Signaling Pathway. Reprod Sci 2015;22:1098-106. [Crossref] [PubMed]
- Dominici M, Le Blanc K, Mueller I, et al. Minimal criteria for defining multipotent mesenchymal stromal cells. The International Society for Cellular Therapy position statement. Cytotherapy 2006;8:315-7. [Crossref] [PubMed]
- Schwab KE, Gargett CE. Co-expression of two perivascular cell markers isolates mesenchymal stem-like cells from human endometrium. Hum Reprod 2007;22:2903-11. [Crossref] [PubMed]
- Chan RW, Ng EH, Yeung WS. Identification of cells with colony-forming activity, self-renewal capacity, and multipotency in ovarian endometriosis. Am J Pathol 2011;178:2832-44. [Crossref] [PubMed]
- Wolff EF, Wolff AB, Du Hongling, et al. Demonstration of multipotent stem cells in the adult human endometrium by in vitro chondrogenesis. Reprod Sci 2007;14:524-33. [Crossref] [PubMed]
- Masuda H, Anwar SS, Bühring HJ, et al. A novel marker of human endometrial mesenchymal stem-like cells. Cell Transplant 2012;21:2201-14. [Crossref] [PubMed]
- Luesink M, Pennings JL, Wissink WM, et al. Chemokine induction by all-trans retinoic acid and arsenic trioxide in acute promyelocytic leukemia: triggering the differentiation syndrome. Blood 2009;114:5512-21. [Crossref] [PubMed]
- Pettersson F, Dalgleish AG, Bissonnette RP, et al. Retinoids cause apoptosis in pancreatic cancer cells via activation of RAR-gamma and altered expression of Bcl-2/Bax. Br J Cancer 2002;87:555-61. [Crossref] [PubMed]
- Gargett CE, Schwab KE, Deane JA. Endometrial stem/progenitor cells: the first 10 years. Hum Reprod Update 2016;22:137-63. [PubMed]
- Pittatore G, Moggio A, Benedetto C, et al. Endometrial adult/progenitor stem cells: pathogenetic theory and new antiangiogenic approach for endometriosis therapy. Reprod Sci 2014;21:296-304. [Crossref] [PubMed]
- Li T, He H, Liu R, et al. Isolation and identification of epithelial and stromal stem cells from eutopic endometrium of women with endometriosis. Eur J Obstet Gynecol Reprod Biol 2014;178:89-94. [Crossref] [PubMed]
- Maruyama T, Masuda H, Ono M, et al. Human uterine stem/progenitor cells: their possible role in uterine physiology and pathology. Reproduction 2010;140:11-22. [Crossref] [PubMed]
- Gargett CE, Masuda H. Adult stem cells in the endometrium. Mol Hum Reprod 2010;16:818-34. [Crossref] [PubMed]
- Koippallil Gopalakrishnan Nair AR, Pandit H, Warty N, et al. Endometriotic mesenchymal stem cells exhibit a distinct immune phenotype. Int Immunol 2015;27:195-204. [Crossref] [PubMed]
- Bharti R, Dey G, Mandal M. Cancer development, chemoresistance, epithelial to mesenchymal transition and stem cells: A snapshot of IL-6 mediated involvement. Cancer Lett 2016;375:51-61. [Crossref] [PubMed]
- So KA, Min KJ, Hong JH, et al. Interleukin-6 expression by interactions between gynecologic cancer cells and human mesenchymal stem cells promotes epithelial-mesenchymal transition. Int J Oncol 2015;47:1451-9. [Crossref] [PubMed]
- Lin JT, Wang JY, Chen MK, et al. Colon cancer mesenchymal stem cells modulate the tumorigenicity of colon cancer through interleukin 6. Exp Cell Res 2013;319:2216-29. [Crossref] [PubMed]
- Endler A, Chen L, Li Q, et al. Int6/eIF3e silenced HIF2α stabilization enhances migration and tube formation of HUVECs via IL-6 and IL-8 signaling. Cytokine 2013;62:115-22. [Crossref] [PubMed]
- Hassan H, Greve B, Pavao MS, et al. Syndecan-1 modulates β-integrin-dependent and interleukin-6-dependent functions in breast cancer cell adhesion, migration, and resistance to irradiation. FEBS J 2013;280:2216-27. [Crossref] [PubMed]
- Mano Y, Aishima S, Fujita N, et al. Tumor-associated macrophage promotes tumor progression via STAT3 signaling in hepatocellular carcinoma. Pathobiology 2013;80:146-54. [Crossref] [PubMed]
- Sullivan NJ, Sasser AK, Axel AE, et al. Interleukin-6 induces an epithelial-mesenchymal transition phenotype in human breast cancer cells. Oncogene 2009;28:2940-7. [Crossref] [PubMed]
- Xie G, Yao Q, Liu Y, et al. IL-6-induced epithelial-mesenchymal transition promotes the generation of breast cancer stem-like cells analogous to mammosphere cultures. Int J Oncol 2012;40:1171-9. [PubMed]
- Colomiere M, Ward AC, Riley C, et al. Cross talk of signals between EGFR and IL-6R through JAK2/STAT3 mediate epithelial-mesenchymal transition in ovarian carcinomas. Br J Cancer 2009;100:134-44. [Crossref] [PubMed]
- Wan J, Ma J, Mei J, et al. The effects of HIF-1alpha on gene expression profiles of NCI-H446 human small cell lung cancer cells. J Exp Clin Cancer Res 2009;28:150. [Crossref] [PubMed]
- Woo JH, Yang YI, Ahn JH, et al. Interleukin 6 secretion from alternatively activated macrophages promotes the migration of endometriotic epithelial cells. Biol Reprod 2017;97:660-70. [Crossref] [PubMed]
- Ren S, Zhou Y, Fang X, et al. PRL-3 Is Involved in Estrogen- and IL-6-Induced Migration of Endometrial Stromal Cells From Ectopic Endometrium. Reprod Sci 2017;24:124-32. [Crossref] [PubMed]
- Li L, Han R, Xiao H, et al. Metformin sensitizes EGFR-TKI-resistant human lung cancer cells in vitro and in vivo through inhibition of IL-6 signaling and EMT reversal. Clin Cancer Res 2014;20:2714-26. [Crossref] [PubMed]
- Taylor RN, Kane MA, Sidell N. Pathogenesis of Endometriosis: Roles of Retinoids and Inflammatory Pathways. Semin Reprod Med 2015;33:246-56. [Crossref] [PubMed]
- Patel BG, Lenk EE, Lebovic DI, et al. Pathogenesis of endometriosis: Interaction between Endocrine and inflammatory pathways. Best Pract Res Clin Obstet Gynaecol 2018;50:50-60. [Crossref] [PubMed]
- Tanmahasamut P, Sidell N. Up-regulation of gap junctional intercellular communication and connexin43 expression by retinoic acid in human endometrial stromal cells. J Clin Endocrinol Metab 2005;90:4151-6. [Crossref] [PubMed]
- Sawatsri S, Desai N, Rock JA, et al. Retinoic acid suppresses interleukin-6 production in human endometrial cells. Fertil Steril 2000;73:1012-9. [Crossref] [PubMed]
- Yamagata Y, Takaki E, Shinagawa M, et al. Retinoic acid has the potential to suppress endometriosis development. J Ovarian Res 2015;8:49. [Crossref] [PubMed]
- Wieser F, Wu J, Shen Z, et al. Retinoic acid suppresses growth of lesions, inhibits peritoneal cytokine secretion, and promotes macrophage differentiation in an immunocompetent mouse model of endometriosis. Fertil Steril 2012;97:1430-7. [Crossref] [PubMed]
- Pavone ME, Malpani SS, Dyson M, et al. Fenretinide: A Potential Treatment for Endometriosis. Reprod Sci 2016;23:1139-47. [Crossref] [PubMed]
- Lu H, Li S, Wu Q. Retinoic acid regulates endometriotic stromal cell growth through upregulation of Beclin1. Arch Gynecol Obstet 2018;297:93-9. [Crossref] [PubMed]
- Ozer H, Boztosun A, Açmaz G, et al. The efficacy of bevacizumab, sorafenib, and retinoic acid on rat endometriosis model. Reprod Sci 2013;20:26-32. [Crossref] [PubMed]