Network pharmacology analysis of the mechanism of our hospital’s experiential prescription in the treatment of Guillain Barré syndrome
Introduction
Guillain-Barré syndrome (GBS) is an immune-related peripheral neuroinflammatory disease (1-3). As the pathogenesis of GBS has not yet been fully clarified, the effects of GBS treatment are limited. Typically, modern medicine uses symptomatic treatment and supportive strategies (e.g., neurotrophic therapy), and the overall outcome is not satisfactory. In order to enhance the effect of GBS treatment, our department developed a hospital-specific experiential traditional Chinese medicine (TCM) prescription for the treatment of GBS. The composition of this prescription is as follows: astragalus 60 g, raw licorice 12 g, atractylodes 10 g, cork 10 g, jobstears seed 10 g, salvia 10 g, red peony 10 g, and Poria cocos 12 g. We previously applied this hospital GBS-experiential prescription (GBS-EP) for 10 years and found that it (alone or in combination with other treatments) can effectively benefit the treatment of GBS. In the follow-up observation, we noticed that the application of GBS-EP can improve the daily living ability score, Hughes limb function score, and help cure sensory dysfunction in patients.
Clinically, the manifestations of GBS are similar to “failure disease” and “heat evil” in TCM theory. The main TCM syndrome differentiation characteristics include Qi-and-Blood deficiency and pathogenic heat. Accordingly, based on TCM theory, the treatment strategy should be strengthening Qi, clearing damp-heat, cooling, and activating blood. However, the main active ingredients, molecular targets/mechanisms of GBS-EP remain largely unclear. In recent years, increasing studies have established the disease/gene-target/drug interaction network based on the analysis of network pharmacology to explore the therapeutic mechanism of TCM prescriptions (4-10). We conducted the present study using network pharmacology to determine the mechanism underlying the effects of GBS-EP at the molecular level, and our findings may provide references for discovering the etiology of GBS and further developing GBS treatment. We present the following article in accordance with the MDAR reporting checklist (available at https://dx.doi.org/10.21037/apm-21-1743).
Methods
The key active ingredients and targets
The traditional Chinese medicine system pharmacology database and analysis platform (TCMSP, http://lsp.nwu.edu.cn/tcmsp.php) was employed, and the following TCM herbs were used to search for active ingredients: astragalus, raw licorice, atractylodes, cork, jobstears seed, salvia, red peony, and Poria cocos. The screening criteria for key active ingredients were set as follows: oral bioavailability (OB) ≥40%; and drug similarity (DL) ≥0.2. The corresponding gene targets for all key active ingredients were then acquired through the CTD database (http://ctdbase.org), and only the Homo sapiens target data were selected.
GBS associated targets
The Online Mendelian Inheritance in Man (OMIM) (https://www.omim.org), Genecards (http://www.genecards.org), and Malacards (https://www.malacards.org) databases were used to search for GBS-associated targets. In the OMIM and Genecards databases, “Guillain-Barre syndrome” was used as the keyword, and in Malacards, the classic GBS subtype “acute inflammatory demyelinating polyneuropathy” was used as the keyword to obtain the targets.
Screening of common targets between GBS-EP and GBS
The targets of GBS-EP and the GBS-associated targets were listed respectively, and their intersection was drawn using a Venn diagram.
Protein-protein interaction (PPI) network
The above common targets were imported into the STRING database (http://string-db.org/cgi/input.pl), and the protein type was set as Homo sapiens. The interaction threshold value was set at 0.7, and only experimentally verified types of PPI were selected. The lonely island nodes were not displayed. The PPI network was generated, and the core targets were selected and paid special attention.
Enrichment analysis
Based on the common targets, the R package clusterProfiler was used to perform Gene oncology (GO) function and Kyoto Encyclopedia of Genes and Genomes (KEGG) pathway enrichment analyses. Here, Gene Ratio was used to indicate the proportion of genes enriched to a specific GO or KEGG term in all targets, and multiple hypothesis tests were performed to adjust the P value calculation. The enrichment results were presented as bubble figures and detailed tables.
The study was conducted in accordance with the Declaration of Helsinki (as revised in 2013).
Statistical analysis
Statistical analyses were performed using R or the corresponding online tools as mentioned above. In enrichment analysis, multiple hypothesis tests were performed to adjust the P value calculation. The P values <0.05 were considered statistically significant.
Results
The active ingredients and corresponding targets of GBS-EP
Using the TCMSP database, we screened the active ingredients of astragalus, licorice, atractylodes, phellodendron, coix seed, salvia, red peony, and poria. A total of 160 key active ingredients were found under the criteria of OB ≥40% and DL ≥0.2; six repeated active ingredients were shared by multiple herbs and removed, and a total of 154 active ingredients were acquired. The specific key ingredients of each medicinal flavor are presented in Table 1. Next, using these 154 key active ingredients, a total of 8,102 targets were obtained via CTD database analysis. After de-duplication, 4,270 non-repetitive targets were obtained.
Table 1
Herb | Number of key ingredients |
---|---|
Astragalus | 12 |
Raw licorice | 65 |
Atractylodes | 3 |
Cork | 15 |
Jobstears seed | 2 |
Salvia | 43 |
Red peony | 17 |
Poria cocos | 3 |
GBS-EP, GBS experiential formula.
GBS associated targets
Using “Guillain-Barre Syndrome” as a keyword, we searched the OMIM and GeneCards databases, respectively. There were eight targets in the Genecards database and six targets in the OMIM database. Also, using the most classic GBS subtype, “acute inflammatory demyelinating polyneuropathy” as the keyword, 69 targets were found on the GeneCards database, and 109 targets were found on the OMIM database. Meanwhile, using Guillain-Barre Syndrome entry (MCID: GLL022) in the MalaCards database, 24 targets were found. After removing duplicates, there were a total of 158 targets.
Common targets
Among the 4,270 unique GBS-EP targets and 158 GBS associated targets, 70 common drug/disease targets were identified, and a Venn diagram was drawn to show the intersection (Figure 1A).
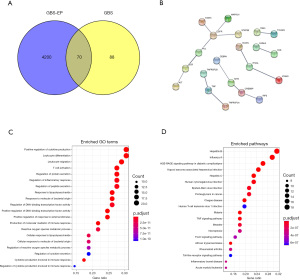
PPI network analysis
Using the STRING database, the PPI network of the above 70 common targets was established (Figure 1B), with 21 nodes and 15 edges. Among them, the target proteins EGFR (Epidermal Growth Factor Receptor), TNF (Tumor Necrosis Factor), ITGAL (Integrin Subunit Alpha L), and CREBBP (CREB Binding Protein) were in the core nodes of the PPI network, which may be highly significant for clarifying the treatment mechanism of GBS-EP.
Pathway enrichment analysis results
Furthermore, the 70 common targets were analyzed by GO and KEGG pathway enrichment, and the results are as follows. In the GO enrichment, a total of 116 enriched terms were found. As shown in Table 2, the enrichment of terms such as inflammatory response, extracellular space, positive regulation of gene expression, extrinsic apoptotic signaling pathway, and positive regulation of transcription from RNA polymerase II promoter may be the crucial mechanism of the therapeutic effects of GBS-EP, and the top 20 terms were presented using the bubble chart in Figure 1C. Meanwhile, a total of 61 KEGG pathways were enriched in the 70 common targets (Table 3). The top 20 pathways are shown in the bubble chart (Figure 1D), and the key KEGG pathways included influenza A, hepatitis B, malaria, etc.
Table 2
Category | Term | Count | % | Fold | FDR |
---|---|---|---|---|---|
BP | GO:0006954~inflammatory response | 20 | 28.57 | 12.66 | 6.55E-13 |
CC | GO:0005615~extracellular space | 29 | 41.43 | 5.61 | 2.15E-12 |
BP | GO:0010628~positive regulation of gene expression | 16 | 22.86 | 14.65 | 8.92E-11 |
BP | GO:0097191~extrinsic apoptotic signaling pathway | 9 | 12.86 | 51.40 | 2.37E-09 |
BP | GO:0045944~positive regulation of transcription from RNA polymerase II promoter | 22 | 31.43 | 5.38 | 5.89E-08 |
CC | GO:0045121~membrane raft | 11 | 15.71 | 13.90 | 3.69E-07 |
CC | GO:0009897~external side of plasma membrane | 11 | 15.71 | 13.44 | 3.69E-07 |
BP | GO:0031663~lipopolysaccharide-mediated signaling pathway | 7 | 10.00 | 52.48 | 6.72E-07 |
BP | GO:0051092~positive regulation of NF-kappaB transcription factor activity | 10 | 14.29 | 18.04 | 6.72E-07 |
BP | GO:0043066~negative regulation of apoptotic process | 15 | 21.43 | 7.91 | 6.72E-07 |
BP | GO:0006955~immune response | 14 | 20.00 | 7.98 | 2.19E-06 |
BP | GO:0071222~cellular response to lipopolysaccharide | 9 | 12.86 | 19.11 | 2.55E-06 |
BP | GO:0045429~positive regulation of nitric oxide biosynthetic process | 7 | 10.00 | 39.05 | 2.55E-06 |
BP | GO:0032496~response to lipopolysaccharide | 10 | 14.29 | 14.63 | 2.55E-06 |
CC | GO:0009986~cell surface | 14 | 20.00 | 6.72 | 4.00E-06 |
BP | GO:0006919~activation of cysteine-type endopeptidase activity involved in apoptotic process | 8 | 11.43 | 23.12 | 4.93E-06 |
BP | GO:0008285~negative regulation of cell proliferation | 13 | 18.57 | 7.88 | 6.37E-06 |
BP | GO:0007165~signal transduction | 20 | 28.57 | 4.13 | 1.17E-05 |
BP | GO:0043406~positive regulation of MAP kinase activity | 7 | 10.00 | 28.46 | 1.23E-05 |
BP | GO:0097296~activation of cysteine-type endopeptidase activity involved in apoptotic signaling pathway | 5 | 7.14 | 92.26 | 1.43E-05 |
MF | GO:0005515~protein binding | 58 | 82.86 | 1.59 | 2.59E-05 |
BP | GO:0050900~leukocyte migration | 8 | 11.43 | 15.73 | 4.95E-05 |
MF | GO:0005125~cytokine activity | 9 | 12.86 | 12.33 | 6.98E-05 |
BP | GO:0019221~cytokine-mediated signaling pathway | 8 | 11.43 | 14.65 | 7.21E-05 |
BP | GO:0032755~positive regulation of interleukin-6 production | 6 | 8.57 | 31.98 | 7.21E-05 |
CC | GO:0005576~extracellular region | 20 | 28.57 | 3.23 | 1.65E-04 |
BP | GO:0008284~positive regulation of cell proliferation | 12 | 17.14 | 6.18 | 1.80E-04 |
BP | GO:0007159~leukocyte cell-cell adhesion | 5 | 7.14 | 47.98 | 1.86E-04 |
BP | GO:0045930~negative regulation of mitotic cell cycle | 5 | 7.14 | 46.13 | 2.09E-04 |
BP | GO:0007568~aging | 8 | 11.43 | 11.63 | 2.64E-04 |
BP | GO:0051607~defense response to virus | 8 | 11.43 | 11.63 | 2.64E-04 |
BP | GO:0070374~positive regulation of ERK1 and ERK2 cascade | 8 | 11.43 | 10.97 | 3.74E-04 |
BP | GO:0033209~tumor necrosis factor-mediated signaling pathway | 7 | 10.00 | 14.23 | 4.29E-04 |
BP | GO:0050729~positive regulation of inflammatory response | 6 | 8.57 | 19.72 | 5.71E-04 |
BP | GO:0030198~extracellular matrix organization | 8 | 11.43 | 9.79 | 6.98E-04 |
MF | GO:0042802~identical protein binding | 14 | 20.00 | 4.51 | 7.24E-04 |
BP | GO:0008625~extrinsic apoptotic signaling pathway via death domain receptors | 5 | 7.14 | 31.56 | 7.28E-04 |
BP | GO:0042517~positive regulation of tyrosine phosphorylation of Stat3 protein | 5 | 7.14 | 31.56 | 7.28E-04 |
BP | GO:0042060~wound healing | 6 | 8.57 | 17.99 | 7.59E-04 |
BP | GO:0006953~acute-phase response | 5 | 7.14 | 30.75 | 7.59E-04 |
BP | GO:0051897~positive regulation of protein kinase B signaling | 6 | 8.57 | 17.13 | 9.27E-04 |
BP | GO:1904707~positive regulation of vascular smooth muscle cell proliferation | 4 | 5.71 | 63.97 | 0.001081 |
CC | GO:0031093~platelet alpha granule lumen | 5 | 7.14 | 23.67 | 0.001424 |
BP | GO:0008630~intrinsic apoptotic signaling pathway in response to DNA damage | 5 | 7.14 | 25.52 | 0.001467 |
BP | GO:0045893~positive regulation of transcription, DNA-templated | 11 | 15.71 | 5.12 | 0.001581 |
BP | GO:0043123~positive regulation of I-kappaB kinase/NF-kappaB signaling | 7 | 10.00 | 10.43 | 0.001754 |
BP | GO:0043536~positive regulation of blood vessel endothelial cell migration | 4 | 5.71 | 50.50 | 0.002035 |
CC | GO:0005886~plasma membrane | 31 | 44.29 | 1.96 | 0.002038 |
BP | GO:0002576~platelet degranulation | 6 | 8.57 | 13.97 | 0.002094 |
BP | GO:0051091~positive regulation of sequence-specific DNA binding transcription factor activity | 6 | 8.57 | 13.71 | 0.002213 |
BP | GO:1902895~positive regulation of pri-miRNA transcription from RNA polymerase II promoter | 4 | 5.71 | 47.98 | 0.002213 |
BP | GO:0001666~response to hypoxia | 7 | 10.00 | 9.76 | 0.002231 |
BP | GO:0050776~regulation of immune response | 7 | 10.00 | 9.43 | 0.002634 |
BP | GO:0031334~positive regulation of protein complex assembly | 4 | 5.71 | 43.62 | 0.0027 |
BP | GO:0000165~MAPK cascade | 8 | 11.43 | 7.32 | 0.0027 |
BP | GO:0071407~cellular response to organic cyclic compound | 5 | 7.14 | 20.33 | 0.0027 |
BP | GO:0006915~apoptotic process | 11 | 15.71 | 4.65 | 0.0027 |
BP | GO:0030335~positive regulation of cell migration | 7 | 10.00 | 9.13 | 0.002832 |
BP | GO:0042127~regulation of cell proliferation | 7 | 10.00 | 9.08 | 0.002858 |
MF | GO:0008201~heparin binding | 7 | 10.00 | 10.55 | 0.002912 |
CC | GO:0070062~extracellular exosome | 24 | 34.29 | 2.22 | 0.003261 |
BP | GO:0050715~positive regulation of cytokine secretion | 4 | 5.71 | 38.38 | 0.003546 |
BP | GO:0060326~cell chemotaxis | 5 | 7.14 | 18.45 | 0.003546 |
BP | GO:0051384~response to glucocorticoid | 5 | 7.14 | 18.45 | 0.003546 |
BP | GO:0001934~positive regulation of protein phosphorylation | 6 | 8.57 | 11.33 | 0.004148 |
BP | GO:0032728~positive regulation of interferon-beta production | 4 | 5.71 | 35.54 | 0.004223 |
BP | GO:0033138~positive regulation of peptidyl-serine phosphorylation | 5 | 7.14 | 17.13 | 0.004471 |
BP | GO:0097190~apoptotic signaling pathway | 5 | 7.14 | 16.89 | 0.004557 |
BP | GO:0060333~interferon-gamma-mediated signaling pathway | 5 | 7.14 | 16.89 | 0.004557 |
CC | GO:0072562~blood microparticle | 6 | 8.57 | 10.28 | 0.004729 |
BP | GO:0043065~positive regulation of apoptotic process | 8 | 11.43 | 6.40 | 0.004922 |
BP | GO:0042981~regulation of apoptotic process | 7 | 10.00 | 7.88 | 0.005101 |
BP | GO:2000553~positive regulation of T-helper 2 cell cytokine production | 3 | 4.29 | 119.94 | 0.005101 |
BP | GO:0097527~necroptotic signaling pathway | 3 | 4.29 | 119.94 | 0.005101 |
BP | GO:0008360~regulation of cell shape | 6 | 8.57 | 10.28 | 0.005581 |
BP | GO:0050728~negative regulation of inflammatory response | 5 | 7.14 | 15.18 | 0.006221 |
BP | GO:0050731~positive regulation of peptidyl-tyrosine phosphorylation | 5 | 7.14 | 14.63 | 0.007063 |
BP | GO:0050830~defense response to Gram-positive bacterium | 5 | 7.14 | 14.11 | 0.007892 |
BP | GO:0018108~peptidyl-tyrosine phosphorylation | 6 | 8.57 | 9.41 | 0.007892 |
BP | GO:0006928~movement of cell or subcellular component | 5 | 7.14 | 13.95 | 0.00809 |
MF | GO:0042803~protein homodimerization activity | 12 | 17.14 | 3.96 | 0.008157 |
BP | GO:0035234~ectopic germ cell programmed cell death | 3 | 4.29 | 89.96 | 0.008374 |
BP | GO:0010888~negative regulation of lipid storage | 3 | 4.29 | 89.96 | 0.008374 |
MF | GO:0004713~protein tyrosine kinase activity | 6 | 8.57 | 10.88 | 0.008484 |
BP | GO:0032930~positive regulation of superoxide anion generation | 3 | 4.29 | 79.96 | 0.010584 |
BP | GO:0001541~ovarian follicle development | 4 | 5.71 | 22.85 | 0.011894 |
BP | GO:0045599~negative regulation of fat cell differentiation | 4 | 5.71 | 22.85 | 0.011894 |
BP | GO:0032760~positive regulation of tumor necrosis factor production | 4 | 5.71 | 20.42 | 0.016107 |
BP | GO:0051781~positive regulation of cell division | 4 | 5.71 | 20.42 | 0.016107 |
CC | GO:0016020~membrane | 19 | 27.14 | 2.25 | 0.016874 |
BP | GO:0032727~positive regulation of interferon-alpha production | 3 | 4.29 | 59.97 | 0.017967 |
BP | GO:0071356~cellular response to tumor necrosis factor | 5 | 7.14 | 10.90 | 0.01801 |
BP | GO:0007157~heterophilic cell-cell adhesion via plasma membrane cell adhesion molecules | 4 | 5.71 | 19.19 | 0.018547 |
BP | GO:0050901~leukocyte tethering or rolling | 3 | 4.29 | 55.36 | 0.020363 |
MF | GO:0046982~protein heterodimerization activity | 9 | 12.86 | 4.67 | 0.020606 |
BP | GO:0048146~positive regulation of fibroblast proliferation | 4 | 5.71 | 17.77 | 0.022342 |
BP | GO:0016032~viral process | 7 | 10.00 | 5.62 | 0.022342 |
BP | GO:0006935~chemotaxis | 5 | 7.14 | 9.83 | 0.024779 |
BP | GO:0008631~intrinsic apoptotic signaling pathway in response to oxidative stress | 3 | 4.29 | 47.98 | 0.025624 |
BP | GO:0060397~JAK-STAT cascade involved in growth hormone signaling pathway | 3 | 4.29 | 47.98 | 0.025624 |
CC | GO:0005829~cytosol | 24 | 34.29 | 1.88 | 0.025833 |
MF | GO:0005178~integrin binding | 5 | 7.14 | 11.48 | 0.026985 |
BP | GO:0007249~I-kappaB kinase/NF-kappaB signaling | 4 | 5.71 | 15.99 | 0.027547 |
BP | GO:0050679~positive regulation of epithelial cell proliferation | 4 | 5.71 | 15.99 | 0.027547 |
BP | GO:0042102~positive regulation of T cell proliferation | 4 | 5.71 | 15.99 | 0.027547 |
BP | GO:0048661~positive regulation of smooth muscle cell proliferation | 4 | 5.71 | 15.99 | 0.027547 |
BP | GO:0044130~negative regulation of growth of symbiont in host | 3 | 4.29 | 44.98 | 0.027547 |
BP | GO:0032722~positive regulation of chemokine production | 3 | 4.29 | 42.33 | 0.030446 |
BP | GO:0042523~positive regulation of tyrosine phosphorylation of Stat5 protein | 3 | 4.29 | 42.33 | 0.030446 |
BP | GO:0010629~negative regulation of gene expression | 5 | 7.14 | 8.75 | 0.033418 |
BP | GO:0030225~macrophage differentiation | 3 | 4.29 | 39.98 | 0.033418 |
BP | GO:0000122~negative regulation of transcription from RNA polymerase II promoter | 10 | 14.29 | 3.33 | 0.035781 |
BP | GO:0007155~cell adhesion | 8 | 11.43 | 4.18 | 0.036558 |
BP | GO:0071260~cellular response to mechanical stimulus | 4 | 5.71 | 13.51 | 0.041224 |
BP | GO:0046427~positive regulation of JAK-STAT cascade | 3 | 4.29 | 32.71 | 0.047843 |
BP | GO:0030324~lung development | 4 | 5.71 | 12.63 | 0.04894 |
GO, gene oncology; BP, biological process; CC, cellular component; MF, molecular function; FDR, false discovery rate.
Table 3
Term | Count | % | Fold | FDR |
---|---|---|---|---|
hsa05164:Influenza A | 18 | 25.71 | 11.86 | 3.55E-12 |
hsa05161:Hepatitis B | 16 | 22.86 | 12.65 | 3.10E-11 |
hsa05144:Malaria | 11 | 15.71 | 25.74 | 2.03E-10 |
hsa05200:Pathways in cancer | 20 | 28.57 | 5.83 | 6.52E-09 |
hsa05142:Chagas disease (American trypanosomiasis) | 12 | 17.14 | 13.23 | 1.67E-08 |
hsa05143:African trypanosomiasis | 8 | 11.43 | 27.79 | 1.40E-07 |
hsa04668:TNF signaling pathway | 11 | 15.71 | 11.79 | 2.55E-07 |
hsa05168:Herpes simplex infection | 13 | 18.57 | 8.14 | 4.08E-07 |
hsa05205:Proteoglycans in cancer | 13 | 18.57 | 7.45 | 9.74E-07 |
hsa05162:Measles | 11 | 15.71 | 9.48 | 1.39E-06 |
hsa05166:HTLV-I infection | 14 | 20.00 | 6.32 | 1.39E-06 |
hsa05323:Rheumatoid arthritis | 9 | 12.86 | 11.73 | 5.59E-06 |
hsa04060:Cytokine-cytokine receptor interaction | 13 | 18.57 | 6.13 | 5.61E-06 |
hsa05321:Inflammatory bowel disease (IBD) | 8 | 11.43 | 14.33 | 7.12E-06 |
hsa05160:Hepatitis C | 10 | 14.29 | 8.62 | 1.05E-05 |
hsa04068:FoxO signaling pathway | 10 | 14.29 | 8.56 | 1.05E-05 |
hsa05152:Tuberculosis | 11 | 15.71 | 7.13 | 1.21E-05 |
hsa04620:Toll-like receptor signaling pathway | 9 | 12.86 | 9.73 | 1.55E-05 |
hsa05133:Pertussis | 8 | 11.43 | 12.23 | 1.56E-05 |
hsa05145:Toxoplasmosis | 9 | 12.86 | 9.38 | 1.85E-05 |
hsa05221:Acute myeloid leukemia | 7 | 10.00 | 14.33 | 3.37E-05 |
hsa04514:Cell adhesion molecules (CAMs) | 9 | 12.86 | 7.27 | 1.11E-04 |
hsa05140:Leishmaniasis | 7 | 10.00 | 11.30 | 1.23E-04 |
hsa04932:Non-alcoholic fatty liver disease (NAFLD) | 9 | 12.86 | 6.83 | 1.59E-04 |
hsa04064:NF-kappa B signaling pathway | 7 | 10.00 | 9.22 | 3.59E-04 |
hsa05332:Graft-versus-host disease | 5 | 7.14 | 17.37 | 6.32E-04 |
hsa05212:Pancreatic cancer | 6 | 8.57 | 10.58 | 7.98E-04 |
hsa05330:Allograft rejection | 5 | 7.14 | 15.49 | 9.15E-04 |
hsa04010:MAPK signaling pathway | 10 | 14.29 | 4.53 | 9.15E-04 |
hsa04622:RIG-I-like receptor signaling pathway | 6 | 8.57 | 9.83 | 9.86E-04 |
hsa04920:Adipocytokine signaling pathway | 6 | 8.57 | 9.83 | 9.86E-04 |
hsa05169:Epstein-Barr virus infection | 7 | 10.00 | 6.58 | 0.001779502 |
hsa04151:PI3K-Akt signaling pathway | 11 | 15.71 | 3.66 | 0.001844849 |
hsa05014:Amyotrophic lateral sclerosis (ALS) | 5 | 7.14 | 11.46 | 0.002439226 |
hsa04210:Apoptosis | 5 | 7.14 | 9.25 | 0.005317397 |
hsa05146:Amoebiasis | 6 | 8.57 | 6.49 | 0.005621139 |
hsa04623:Cytosolic DNA-sensing pathway | 5 | 7.14 | 8.96 | 0.005656646 |
hsa04670:Leukocyte transendothelial migration | 6 | 8.57 | 5.98 | 0.007605907 |
hsa04917:Prolactin signaling pathway | 5 | 7.14 | 8.07 | 0.007849669 |
hsa04650:Natural killer cell mediated cytotoxicity | 6 | 8.57 | 5.64 | 0.009324623 |
hsa04062:Chemokine signaling pathway | 7 | 10.00 | 4.31 | 0.011938051 |
hsa05219:Bladder cancer | 4 | 5.71 | 11.19 | 0.011949943 |
hsa04940:Type I diabetes mellitus | 4 | 5.71 | 10.92 | 0.012494005 |
hsa04640:Hematopoietic cell lineage | 5 | 7.14 | 6.59 | 0.014401449 |
hsa04550:Signaling pathways regulating pluripotency of stem cells | 6 | 8.57 | 4.91 | 0.014832723 |
hsa04672:Intestinal immune network for IgA production | 4 | 5.71 | 9.76 | 0.016004866 |
hsa04630:Jak-STAT signaling pathway | 6 | 8.57 | 4.74 | 0.016251128 |
hsa05203:Viral carcinogenesis | 7 | 10.00 | 3.91 | 0.016251128 |
hsa04015:Rap1 signaling pathway | 7 | 10.00 | 3.82 | 0.017832046 |
hsa04066:HIF-1 signaling pathway | 5 | 7.14 | 5.97 | 0.017867158 |
hsa05320:Autoimmune thyroid disease | 4 | 5.71 | 8.82 | 0.019089807 |
hsa04660:T cell receptor signaling pathway | 5 | 7.14 | 5.73 | 0.019773142 |
hsa04621:NOD-like receptor signaling pathway | 4 | 5.71 | 8.19 | 0.022491681 |
hsa04014:Ras signaling pathway | 7 | 10.00 | 3.55 | 0.022744545 |
hsa05202:Transcriptional misregulation in cancer | 6 | 8.57 | 4.12 | 0.024897494 |
hsa05210:Colorectal cancer | 4 | 5.71 | 7.40 | 0.028012543 |
hsa05211:Renal cell carcinoma | 4 | 5.71 | 6.95 | 0.032508076 |
hsa04071:Sphingolipid signaling pathway | 5 | 7.14 | 4.78 | 0.032740155 |
hsa05220:Chronic myeloid leukemia | 4 | 5.71 | 6.37 | 0.039485241 |
hsa04380:Osteoclast differentiation | 5 | 7.14 | 4.38 | 0.042128115 |
hsa05310:Asthma | 3 | 4.29 | 11.47 | 0.043777439 |
KEGG, Kyoto Encyclopedia of Genes and Genomes; FDR, false discovery rate.
Discussion
At present, there is no specific drug for GBS, and established treatment is generally based on immune-modulating treatment with plasma exchange or intravenous immunoglobulin in combination with supportive care (11-13). Recently, some novel drugs have been reported to be potentially effective, such as memantine, bifidobacterium, gabapentin, etc. (14-16). However, the effect of TCM was seldom investigated in GBS treatment. The application of network pharmacology in Chinese medicine has attracted the attention of researchers in recent years. Network pharmacology is a novel, promising, and cost-effective approach in discovering bioactive ingredients, predicting drug action targets, and analyzing drug action mechanisms from the perspective of biological network balance (17). It has been used in different diseases, such as ankylosing spondylitis, cancer, myocardial infarction, anemia, ulcerative colitis, etc. (17-21). The GBS-EP used in our hospital is composed of eight TCM herbs. After screening, a total of 154 active ingredients were obtained, such as the main active ingredients of astragalus syringae (including nicotinic acid, linolenic acid, proline, etc.), the main active ingredients of licorice (including glycol, good rotol, isorhamnetin, cadherin, naringin, glycyrrhizin, etc.), the main active ingredients of atractylodes (including 3-acetoxy atractylone, 2-hydroxyisooxypropyl-3-hydroxy-7-iso Pentene-2,3-dihydrobenzofuran-5-carboxylic acid, etc.), the main active ingredients of cork (including opazone, pterin, dehydrotanshinone II A, niromycin, rutin, quercetin, etc.), the main active ingredients of coix seed (e.g., sitosterol), the main active ingredients of salvia (including paclitaxel, isoperatorin, dehydrotanshinone II A, gallate, formyl tanshinone, etc.), the main active ingredients of red peony (including ellagic acid, paeoniflorin, spinach sterol, 9-ethyl-neo-cocoside A, etc.), and the main active ingredients of poria cocos (including ergosterol-3β-ol, ergosterol peroxide, and dehydroepidermis sour, etc.) A total of 70 common drug/disease targets were discovered, including CEBPA (CCAAT/Enhancer-Binding Protein Alpha), CPT2 (Carnitine Palmitoyltransferase 2), CRP (C-reactive protein), ICAM1 (Intercellular Adhesion Molecule 1), IL6 (interleukin 6), and PECAM1 (Platelet And Endothelial Cell Adhesion Molecule 1), and proteins such as EGFR, TNF, ITGAL, etc. were at key nodes in the PPI network.
The above genes may be a novel target for GBS treatment. For example, the relationship between TNF and GBS has been reported in a number of studies, showing that it is highly related to the inflammatory response of GBS, and there have been case reports claiming that anti-TNF therapy may trigger the occurrence of GBS (22). All types of GBS have been reported to have increased serum levels of TNF-α (23,24). There are some evidences that EGFR and ITGAL are directly involved in GBS; in particular, the EGFR signal plays an important role in demyelination and remyelination e, which may underpin a part of the mechanism of GBS development. Thus, anti-EGFR therapy may have a potential therapeutic value. Also, ITGAL is associated with multiple sclerosis (25). It is possible that these mechanisms (similar to demyelination-like effects, remyelination, multiple sclerosis) mediate the occurrence of GBS. The CREBBP gene is ubiquitously expressed and involved in the transcriptional co-activation of many different transcription factors. It binds to cAMP-response element binding protein (CREB), this gene is now known to play critical roles in embryonic development, growth control, and homeostasis by coupling chromatin remodeling to transcription factor recognition. The direct influence of CREBBP on GBS is still unknown. A study used quantitative global gene expression microarray and analyzed the peripheral blood leukocytes samples of 7 GBS patients with and 7 healthy controls (26). They found that CREBBP was significantly increased (fold change =2.56, P=0.034), and they also proved that CREB (the target of CREBBP) is one of the important hub genes. CPT2 is a nuclear protein which is transported to the mitochondrial inner membrane. The variation of CPT2 may cause symptoms like recurrent myoglobinuria, episodes of muscle pain, stiffness, and rhabdomyolysis (27,28), which are consistent with manifestation of GBS. Moreover, it was reported that GBS and CPT2-deficiency associated increase of rhabdomyolysis episodes are both statin-induced muscle symptoms. However, the definite causal relationship between GBS and CPT2 needs further investigation. Collectively, many well-known targets, and especially inflammatory roles, may be involved in the therapeutic mechanism of the GBS-EP and the pathogenesis of GBS, and are worthy of in-depth follow-up study.
In GO enrichment analysis, the enrichments of biological processes and cellular components were the most significant, such as GO:0006954-inflammatory response, GO:0005615 extracellular space, GO:0010628 positive regulation of gene expression, GO:0097191 extrinsic apoptotic signaling pathway, GO:0045944 positive regulation of transcription from the RNA polymerase II promoter, GO:0045121 membrane raft, GO:0009897 external side of plasma membrane, GO:0031663 lipopolysaccharide-mediated signaling pathway, GO:0051092 positive regulation of NF-kappaB transcription factor activity, GO:0043066-negative regulation of the apoptotic process, and GO:0006955-immune response, etc. This is consistent with the established etiological and pathological phenotypic characteristics of GBS (29-33).
In the signaling pathways, influenza A, hepatitis B, malaria, and hepatitis C were highly enriched. Interestingly, this result implied that the herbs in the GBS-EP, with excellent activity of “strengthening the Zheng-Qi and clearing away the Heat-Evil” in the TCM theory, can target similar roles to the pathogenesis of the hepatitis B, hepatitis C, influenza A, malaria, etc. This is reasonable as the pathogenesis of GBS can include abnormal immune response caused by infectious pathogens. On the other hand, clinical evidence also shows that GBS can be induced after injection of the influenza A (34-37) and hepatitis B (38-40) vaccines. Moreover, malaria and GBS have also been reported to be comorbidities (41-43). The enrichment results of the above signaling pathways help in understanding the pathogenesis of GBS and also in discovering potential treatments for GBS from the aforementioned comorbid phenomena. In addition, we noticed some unseen enrichment of signaling pathways for GBS, such as hsa04068: FoxO signaling pathway, hsa05321: inflammatory bowel disease (IBD), etc. These signals are highly novel and are worthy of further exploration.
Based on above analysis, the results can help guide clinical practice of GBS treatment. For example, some hub genes may play more important roles than others (e.g., EGFR, TNF, and ITGAL), and the proportion of the ingredients/herbs (such as licorice, atractylodes, and cork) associated with these genes can be increased to test a potential performance optimization our hospital’s experiential prescription. Moreover, our experiential prescription may alternatively play a role when some drugs cannot be given in some scenarios. For example, IL-6 is a common target of many herbs in this prescription and a GBS relative gene. For some patients cannot receive the IL-6 antibody treatment for social or medical environmental reasons, this prescription can be used as a preferred choice. We believe that the outcomes of this work may help conduct drug innovation through the network pharmacology analysis. However, there are some difficulties at present. For example, the content of specific ingredients of each drug is not fully understood. In addition, currently, the oral bioavailability of each ingredient was obtained only through the database, and there is no actual animal or clinically validating data. In addition, the level of the specific targets of these ingredients in the key pathological tissues and cell types of GBS still needs to be identified.
Still, this study has some limitations. The major limitation is that it does not include the laboratory verification of some important molecules, such as EGFR, TNF, and ITGAL. Moreover, some proteins within the common-target set might be detectable in the blood sample using ELSIA or Cytokine chips, such as IL6, TGFB1, TNF-α, VEGFA, MMP9, CXCL10, IL10, IL1A, CCL3, and ICAM3. Our further work will accumulate sufficient clinical samples to provide conducive evidence (by dividing individuals into the healthy control, GBS, and GBS-treated-by-TCM). Besides, we mainly focused on the level of gene expression, but the correlation between metabolome changes and the key ingredients are not clear.
In summary, this study used network pharmacology to analyze the mechanism of the hospital GBS-EP in GBS treatment. The 154 active ingredients (such as quercetin, dehydrotanshinone IIA, stigmasterol, etc.) may target key genes (such as EGFR, TNF, and ITGAL, etc.) and play a therapeutic role through crucial functions, like the inflammatory response, extracellular space, and positive regulation of gene expression, and signaling pathways, such as influenza A, hepatitis B, malaria, and hepatitis C.
Acknowledgments
Funding: None.
Footnote
Reporting Checklist: The authors have completed the MDAR reporting checklist. Available at https://dx.doi.org/10.21037/apm-21-1743
Conflicts of Interest: All authors have completed the ICMJE uniform disclosure form (available at https://dx.doi.org/10.21037/apm-21-1743). The authors have no conflicts of interest to declare.
Ethical Statement: The authors are accountable for all aspects of the work in ensuring that questions related to the accuracy or integrity of any part of the work are appropriately investigated and resolved. The study was conducted in accordance with the Declaration of Helsinki (as revised in 2013).
Open Access Statement: This is an Open Access article distributed in accordance with the Creative Commons Attribution-NonCommercial-NoDerivs 4.0 International License (CC BY-NC-ND 4.0), which permits the non-commercial replication and distribution of the article with the strict proviso that no changes or edits are made and the original work is properly cited (including links to both the formal publication through the relevant DOI and the license). See: https://creativecommons.org/licenses/by-nc-nd/4.0/.
References
- Mirian A, Nicolle MW, Budhram A. Guillain-Barré syndrome. CMAJ 2021;193:E378 [Crossref] [PubMed]
- Papri N, Islam Z, Leonhard SE, et al. Guillain-Barré syndrome in low-income and middle-income countries: challenges and prospects. Nat Rev Neurol 2021;17:285-96. [Crossref] [PubMed]
- Shahrizaila N, Lehmann HC, Kuwabara S. Guillain-Barré syndrome. Lancet 2021;397:1214-28. [Crossref] [PubMed]
- Chen XL, Xiao QL, Pang ZH, et al. Molecular mechanisms of An-Chuan Granule for the treatment of asthma based on a network pharmacology approach and experimental validation. Biosci Rep 2021;41:BSR20204247 [Crossref] [PubMed]
- Feng C, Zhao M, Jiang L, et al. Mechanism of Modified Danggui Sini Decoction for Knee Osteoarthritis Based on Network Pharmacology and Molecular Docking. Evid Based Complement Alternat Med 2021;2021:6680637 [Crossref] [PubMed]
- Feng SH, Zhao B, Zhan X, et al. Danggui Buxue Decoction in the Treatment of Metastatic Colon Cancer: Network Pharmacology Analysis and Experimental Validation. Drug Des Devel Ther 2021;15:705-20. [Crossref] [PubMed]
- Guo C, Kang X, Cao F, et al. Network Pharmacology and Molecular Docking on the Molecular Mechanism of Luo-hua-zi-zhu (LHZZ) Granule in the Prevention and Treatment of Bowel Precancerous Lesions. Front Pharmacol 2021;12:629021 [Crossref] [PubMed]
- He DD, Zhang XK, Zhu XY, et al. Network pharmacology and RNA-sequencing reveal the molecular mechanism of Xuebijing injection on COVID-19-induced cardiac dysfunction. Comput Biol Med 2021;131:104293 [Crossref] [PubMed]
- Li X, Tang H, Tang Q, et al. Decoding the Mechanism of Huanglian Jiedu Decoction in Treating Pneumonia Based on Network Pharmacology and Molecular Docking. Front Cell Dev Biol 2021;9:638366 [Crossref] [PubMed]
- Shi H, Tian S, Tian H. Network Pharmacology Interpretation of Fuzheng-Jiedu Decoction against Colorectal Cancer. Evid Based Complement Alternat Med 2021;2021:4652492 [Crossref] [PubMed]
- Yang L, Zhao X. Integrated Chinese and Western Medicine for Acute Guillain-barré Syndrome Treatment. Transl Neurosci 2020;11:38-47. [Crossref] [PubMed]
- Jia L, Zhang HL. Plasma Exchange-Intravenous Immunoglobulin Synergy in the Treatment of Guillain-Barré Syndrome. J Child Neurol 2020;35:346-7. [Crossref] [PubMed]
- Doets AY, Hughes RA, Brassington R, et al. Pharmacological treatment other than corticosteroids, intravenous immunoglobulin and plasma exchange for Guillain-Barré syndrome. Cochrane Database Syst Rev 2020;1:CD008630 [Crossref] [PubMed]
- Siddharthan V, Wang H, de Oliveira AL, et al. Memantine treatment reduces the incidence of flaccid paralysis in a zika virus mouse model of temporary paralysis with similarities to Guillain-Barré syndrome. Antivir Chem Chemother 2020;28:2040206620950143 [Crossref] [PubMed]
- Shi P, Qu H, Nian D, et al. Treatment of Guillain-Barré syndrome with Bifidobacterium infantis through regulation of T helper cells subsets. Int Immunopharmacol 2018;61:290-6. [Crossref] [PubMed]
- Liu J, Wang LN, McNicol ED. Pharmacological treatment for pain in Guillain-Barré syndrome. Cochrane Database Syst Rev 2015;CD009950 [Crossref] [PubMed]
- Zhang J, Zhou Y, Ma Z. Multi-target mechanism of Tripteryguim wilfordii Hook for treatment of ankylosing spondylitis based on network pharmacology and molecular docking. Ann Med 2021;53:1090-8. [Crossref] [PubMed]
- Wang Y, Chu F, Lin J, et al. Erianin, the main active ingredient of Dendrobium chrysotoxum Lindl, inhibits precancerous lesions of gastric cancer (PLGC) through suppression of the HRAS-PI3K-AKT signaling pathway as revealed by network pharmacology and in vitro experimental verification. J Ethnopharmacol 2021;279:114399 [Crossref] [PubMed]
- Li FH, Guo SW, Zhan TW, et al. Integrating network pharmacology and experimental evidence to decipher the cardioprotective mechanism of Yiqihuoxue decoction in rats after myocardial infarction. J Ethnopharmacol 2021;279:114062 [Crossref] [PubMed]
- Wang W, Xu C, Li X, et al. Exploration of the potential mechanism of Banxia Xiexin Decoction for the effects on TNBS-induced ulcerative colitis rats with the assistance of network pharmacology analysis. J Ethnopharmacol 2021;277:114197 [Crossref] [PubMed]
- Wu L, Chen Y, Chen M, et al. Application of network pharmacology and molecular docking to elucidate the potential mechanism of Astragalus-Scorpion against prostate cancer. Andrologia 2021;53:e14165 [Crossref] [PubMed]
- Patwala K, Crump N, De Cruz P. Guillain-Barré syndrome in association with antitumour necrosis factor therapy: a case of mistaken identity. BMJ Case Rep 2017;2017:bcr-2017-219481 [Crossref] [PubMed]
- Gigli GL, Vogrig A, Nilo A, et al. HLA and immunological features of SARS-CoV-2-induced Guillain-Barré syndrome. Neurol Sci 2020;41:3391-4. [Crossref] [PubMed]
- Huang P, Xu M, He XY. Correlations between microRNA-146a and immunoglobulin and inflammatory factors in Guillain-Barré syndrome. J Int Med Res 2020;48:300060520904842 [Crossref] [PubMed]
- Damotte V, Guillot-Noel L, Patsopoulos NA, et al. A gene pathway analysis highlights the role of cellular adhesion molecules in multiple sclerosis susceptibility. Genes Immun 2014;15:126-32. [Crossref] [PubMed]
- Chang KH, Chuang TJ, Lyu RK, et al. Identification of gene networks and pathways associated with Guillain-Barré syndrome. PLoS One 2012;7:e29506 [Crossref] [PubMed]
- Sigauke E, Rakheja D, Kitson K, et al. Carnitine palmitoyltransferase II deficiency: a clinical, biochemical, and molecular review. Lab Invest 2003;83:1543-54. [Crossref] [PubMed]
- Olpin SE, Afifi A, Clark S, et al. Mutation and biochemical analysis in carnitine palmitoyltransferase type II (CPT II) deficiency. J Inherit Metab Dis 2003;26:543-57. [Crossref] [PubMed]
- Rodríguez Y, Rojas M, Pacheco Y, et al. Guillain-Barré syndrome, transverse myelitis and infectious diseases. Cell Mol Immunol 2018;15:547-62. [Crossref] [PubMed]
- Walling AD, Dickson G. Guillain-Barré syndrome. Am Fam Physician 2013;87:191-7. [PubMed]
- Kieseier BC, Mathey EK, Sommer C, et al. Immune-mediated neuropathies. Nat Rev Dis Primers 2018;4:31. [Crossref] [PubMed]
- Mazzeo A, Aguennouz M, Messina C, et al. Immunolocalization and activation of transcription factor nuclear factor kappa B in dysimmune neuropathies and familial amyloidotic polyneuropathy. Arch Neurol 2004;61:1097-102. [Crossref] [PubMed]
- Conti G, Scarpini E, Rostami A, et al. Schwann cell undergoes apoptosis during experimental allergic neuritis (EAN). J Neurol Sci 1998;161:29-35. [Crossref] [PubMed]
- Prestel J, Volkers P, Mentzer D, et al. Risk of Guillain-Barré syndrome following pandemic influenza A(H1N1) 2009 vaccination in Germany. Pharmacoepidemiol Drug Saf 2014;23:1192-204. [Crossref] [PubMed]
- Wiwanitkit V. Guillain-Barré syndrome after H1N1 influenza: a concern. Neuroepidemiology 2013;40:295. [Crossref] [PubMed]
- Kim C, Rhie S, Suh M, et al. Pandemic influenza A vaccination and incidence of Guillain-Barré syndrome in Korea. Vaccine 2015;33:1815-23. [Crossref] [PubMed]
- Romio S, Weibel D, Dieleman JP, et al. Guillain-Barré syndrome and adjuvanted pandemic influenza A (H1N1) 2009 vaccines: a multinational self-controlled case series in Europe. PLoS One 2014;9:e82222 [Crossref] [PubMed]
- Sonavane AD, Saigal S, Kathuria A, et al. Guillain-Barré syndrome: rare extra-intestinal manifestation of hepatitis B. Clin J Gastroenterol 2018;11:312-4. [Crossref] [PubMed]
- Seti NK, Reddi R, Anand I, et al. Gulliane Barre syndrome following vaccination with hepatitis B vaccine. J Assoc Physicians India 2002;50:989. [PubMed]
- Sinsawaiwong S, Thampanitchawong P. Guillain - Barre' syndrome following recombinant hepatitis B vaccine and literature review. J Med Assoc Thai 2000;83:1124-6. [PubMed]
- Kanjalkar M, Karnad DR, Narayana RV, et al. Guillain-Barre syndrome following malaria. J Infect 1999;38:48-50. [Crossref] [PubMed]
- . Shubhakaran. Guillain Barre Syndrome and Malaria. J Assoc Physicians India 2014;62:867. [PubMed]
- Sokrab TE, Eltahir A, Idris MN, et al. Guillain-Barré syndrome following acute falciparum malaria. Neurology 2002;59:1281-3. [Crossref] [PubMed]
(English Language Editor: A. Kassem)