The association of leukocyte telomere length and intermediate coronary lesions—a retrospective study
Introduction
It has been reported that about 70% of acute coronary syndromes occurred due to intermediate coronary stenosis (1,2) and approximately 6% intermediate coronary lesions would worsen and require percutaneous coronary intervention (PCI) by 1 year (3). It is thought that plaque instability is responsible for most cases of acute coronary syndrome (ACS) and thin-cap fibro atheroma (TCFA), along with macrophage accumulation, microchannel, and cholesterol crystals, is considered characteristic of unstable plaques (4). TCFA is a form of lipid plaque, recognized for its high-risk plaque rupture (5). Clinical investigations have shown that TCFA is a significant predictor for rapid progression of angiographic stenosis (6) and a strong predictor for future major adverse cardiovascular events (7,8). It is thought that TCFA are often situated near regions of mild luminal stenosis (9,10). Currently, there are no indicators that can predict the existence of TCFA in intermediate coronary lesion patients. Although risk factors including smoking, hypertension, diabetes mellitus (DM), and hyperlipemia can weakly predict the incidence of ACS, patient stratification remains challenging. Novel predictive biomarkers that facilitate better risk stratification and personalized management are urgently needed.
Intracoronary optical coherence tomography (OCT) is an emerging technology that enables cross-sectional vascular imaging with approximately 10–20 μm resolution. The relatively high resolution of OCT makes it a powerful tool for both quantitative plaque analysis and qualitative plaque characterization (11). OCT provide detailed in vivo information on vessel structures and plaque characteristics, including macrophages accumulation, microchannel, cholesterol crystals, fibrous cap thickness lipid length, and lipid arc. Although coronary angiogram and OCT are powerful diagnostic tools for coronary artery diseases, the invasive nature and cost often make it prohibitive to implement on a mass scale.
Telomeric DNA is located at the end of chromosomes, consists of TTAGGG tandem repeats, and is important for maintaining genomic stability. Telomeres, a biomarker of aging, gradually shortened during each cell division due to replication end problem, and when telomeric DNA reaches a critical length, cells enter replicative senescence (12,13). Given clinical accessibility, leukocyte telomere length (LTL) has been used to better stratify age related diseases including ischemic heart disease.
Here we sought to investigate whether LTL can serve as a biomarker to predict whether a patient, who has a coronary intermediate lesion, requires PCI treatment or not. Moreover, we attempted to explore the potential association between LTL and plaque instability in this retrospective study. We present the following article in accordance with the STROBE reporting checklist (available at https://apm.amegroups.com/article/view/10.21037/apm-21-2295/rc).
Methods
Study sample
Study sample were retrospectively selected with patients that exhibited 40–70% diameter stenosis (based on coronary angiography) and whom underwent OCT examinations, from Shanghai Ninth People’s Hospital, Shanghai Jiao Tong University School of Medicine from the period of January 1, 2016 to December 31, 2017. Exclusion criteria include: (I) ST-segment elevation myocardial infarction (STEMI) and non-ST-segment elevation myocardial infarction (NSTEMI); (II) left main disease, chronic total occlusion (CTO), extremely tortuous vessels or heavily calcified lesions; (III) previous PCI or coronary artery bypass graft (CABG) on the target vessel; (IV) serious liver and kidney dysfunction; (V) malignant tumors; (VI) inflammatory diseases.
From total of 3,106 patients that underwent angiogram, 417 patients exhibiting intermediate coronary lesion (40–70% diameter stenosis) underwent OCT. On the basis of the inclusion and exclusion criteria, a total of 121 participants were included finally (Figure 1). Patients were divided into two groups according to whether they had PCI (PCI group, n=71) or not (non-PCI group, n=50). All information was obtained from the electronic medical record system and outpatient or telephone follow-up.
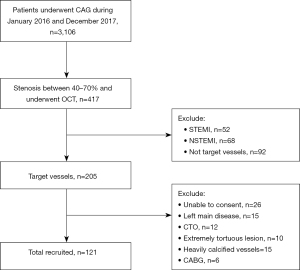
Blood samples were collected after overnight fasting to evaluate serum levels of total cholesterol, low density lipoprotein cholesterol (LDL-C), HDL-C, triglycerides (TG), glucose, white blood cells (WBC), and c-reactive protein (CRP). Blood samples for LTL measurement were collected when patients underwent OCT and samples were stored at −80 ℃ prior to DNA processing in the biobank of Ninth People’s Hospital. All blood works were quantified by standard methods.
Angiogram and analysis
Patients were pre-treated with aspirin (300 mg) and ticagrelor (180 mg) or clopidogrel (300 mg) at least 2 hours prior to angiogram. Coronary angiography was performed via the femoral or radial approach using 6-F or 7-F guiding catheters. Angiographic analyses were performed using Cardiovascular Angiography Analysis System 5.10 (Pie Medical Imaging B.V., Maastricht, Netherlands) by two independent investigators. Minimal luminal diameter (MLD) was the smallest lumen diameter in the lesion segment; reference vessel diameter was defined as the averaged diameter of the proximal and distal coronary segments without obvious narrowing; percentage (%) diameter stenosis (DS) was calculated as: (reference vessel diameter − minimum lumen diameter)/(reference vessel diameter) ×100%. Vessels identified as target vessel based on the patient’s symptoms, electrocardiogram, echocardiography, and other examination results that included in the analysis.
OCT imaging and analysis
Patients with intermediate coronary lesions (40–70% occlusion) were subjected to OCT imaging. OCT was performed using a frequency-domain OCT imaging system (ILUMIEN; St. Jude Medical Inc., St Paul, MN, USA). A 0.016-inch OCT catheter (Image Wire; Light-Lab Imaging/Saint Jude Medical, Westford, MA, USA) was advanced to the distal site of the target lesion through a balloon catheter. The occlusion balloon was inflated at 0.5–0.7 atmosphere at the proximal site of the lesion. Contrast medium was infused into the coronary artery from the distal tip of the occlusion balloon catheter at 0.5–2.0 mL/s by a high-pressure injector. The vessel was imaged with an automatic pullback device at 3 mm/s.
OCT image analysis was carried out using Light-Lab Imaging software at Ninth People's Hospital, Shanghai Jiao Tong University School of Medicine. Two experienced investigators analyzed the OCT images in a blinded fashion. The morphology of the lesions was evaluated based on the 2012 Intravascular Optical Coherence Tomography guideline (14). In case of disagreement between the reviewers, a third reader was involved to reach a consensus. In this retrospective study, PCI was performed when at least one of the following criteria was met: (I) area stenosis (AS) between 50% and 70% and minimal luminal area (MLA) <2.5 mm2; (II) AS between 50% and 70% and plaque rupture (15). Patient's symptoms, electrocardiogram, echocardiography, and other examination results should be considered sometimes. The lesion near smallest lumen area was included for analysis.
The lesion length was measured in the longitudinal view around the lesion site with the smallest lumen area. The lipid-rich plaque was those the necrotic core arc greater than 90°. The lipid arc was measured at 1-mm intervals throughout the length of each lesion and the values were averaged. The lipid length was measured in the longitudinal view. The lipid index was defined as the mean lipid arc multiplied by the lipid length. Fibrous cap was defined as a distinct layer of connective tissue covering the lipid-rich plaque (16). Macrophages normally showed multiple strong back reflections; Microchannel was defined as a black hole with a diameter of 50–300 mm that was present on at least 3 consecutive frames; Cholesterol crystals were defined as linear, highly backscattering structures within the plaque (17).
Real-time PCR quantification of telomere length
DNA extraction was performed using a commercially available kit (Easy Pure Blood Genomic DNA Kit, Trans-Gen Biotech, Beijing, China) following standard procedures. The average telomere lengths of patient DNA samples were quantified by real-time quantitative polymerase chain reaction (RT-qPCR) in a LightCycler480 II Real-Time PCR system (Roche Diagnostics International, Rot-kreuz, Switzerland) using Scien-Cell’s Absolute Human Telomere Length Quantification qPCR Assay Kit (Carlsbad, CA, USA) following manufacturers’ protocol. All reactions were carried in triplicates.
MACE assessment
Follow-up information was collected from a comprehensive medical record database generated based on clinical visits or phone call interviews. All patients were followed up for at least 24 months. Major adverse clinical events (MACE) were defined as cardiac death, acute myocardial infarction (AMI), and/or target lesion revascularization (TLR). (I) Cardiac deaths were defined as all deaths without clear non-cardiac causes; (II) myocardial infarctions were defined based on the diagnostic criteria of the 4th universal definition of myocardial infarction consensus; (III) target lesion revascularizations (TLR) were defined as PCI or coronary artery bypass grafting on target lesion.
Statistical analysis
All statistical analyses were performed using SPSS 26 (SPSS, IBM, Armonk, NY, USA). Continuous variables were expressed as mean ± standard deviation (SD) unless otherwise specified; categorical variables were expressed as numbers and percentages. Continuous variables were assessed using unpaired Student’s t-tests, while categorical variables were compared using chi-square tests. Logistic regression models were used to explore the influence of independent variables on PCI as the dependent variable. The areas under the receiver operating characteristic (ROC) curves for LTL related to PCI was calculated. The relationships between two numerical variables were investigated using a simple linear regression analysis. The incidence of MACE, was studied using the Kaplan-Meier method and compared using the log-rank test. A P value less than 0.05 was considered statistically significant.
Study approval
This study protocol conforms to the ethical guidelines of the 2013 Declaration of Helsinki and was approved by the Ethics Committee of Ninth People’s Hospital, Shanghai Jiao Tong University School of Medicine, Shanghai, China (No. SH9H-2021-T1-1). All individual consent for this retrospective analysis was waived.
Results
A total of 121 participants (80 males and 41 females; mean age 65.29±10.43 years, minimum 30 years, and maximum 85 years) were included in this study. Of these 121 patients, 71 patients received PCI treatment (PCI group, n=71) and 50 did not (non-PCI group, n=50).
The clinical characteristics of the patients are shown in Table 1. There were no significant differences in age, hypertension, hyperlipidemia, cerebral infarction, kidney dysfunction and use of drugs before admitted or at discharge between the PCI and the non-PCI group. Average leukocyte telomere length was significantly shorter in the PCI group compared to the non-PCI group (12.54±2.70 vs. 15.32±3.72 kb, P<0.001). The PCI group contained more men (73.2% vs. 56.0%, P=0.049), higher incidence of diabetes mellitus history (39.4% vs. 22.0%, P=0.043), and higher incidence of CHD family history (60.6% vs. 40.0%, P=0.026). Among other clinical characteristics we observed no significant difference between the two groups with exception to HDL and LDL levels. The PCI group exhibited higher LDL levels (2.93±1.08 vs. 2.52±0.98 mmol/L, P=0.034) and lower HDL levels (1.03±0.33 vs. 1.28±0.82 mmol/L, P=0.044) compared to the non-PCI patients.
Table 1
Variables | Non-PCI (n=50) | PCI (n=71) | t/U/χ2 value | P value |
---|---|---|---|---|
Age, years | 66.14±10.54 | 64.69±10.38 | −0.752 | 0.454 |
Men, n (%) | 28 (56.0) | 52 (73.2) | 3.892 | 0.049 |
Hypertension, n (%) | 34 (68.0) | 40 (56.3) | 1.68 | 0.195 |
DM, n (%) | 11 (22.0) | 28 (39.4) | 4.084 | 0.043 |
Smoker, n (%) | 22 (44.0) | 41 (57.7) | 2.221 | 0.136 |
Hyperlipidemia, n (%) | 20 (40.0) | 35 (49.3) | 1.352 | 0.245 |
Family history, n (%) | 20 (40.0) | 43 (60.6) | 4.971 | 0.026 |
Cerebral infarction, n (%) | 8 (16.0) | 14 (19.7) | 0.273 | 0.602 |
Kidney dysfunction, n (%) | 7 (14.0) | 3 (4.2) | 3.697 | 0.054 |
TC, mmol/L | 4.12±1.17 | 4.08±1.15 | −0.177 | 0.86 |
HDL-C, mmol/L | 1.28±0.82 | 1.03±0.33 | −2.062 | 0.044 |
LDL-C, mmol/L | 2.52±0.98 | 2.93±1.08 | 2.143 | 0.034 |
TG, mmol/L | 1.84±1.40 | 1.42±0.74 | −1.938 | 0.057 |
Glucose, mmol/L | 6.35±2.72 | 6.03±2.09 | −0.714 | 0.477 |
WBC, (109/L) | 6.30±2.03 | 6.86±2.54 | −1.619 | 0.108 |
CRP, mg/dL | 3.61±2.42 | 5.77±11.39 | 1.323 | 0.188 |
Cr, μmol/L | 88.70±33.69 | 96.51±107.82 | 0.495 | 0.622 |
Treatment before admitted | ||||
Anti-platelet | 25 (50.0) | 39 (54.9) | 0.286 | 0.593 |
Statins | 18 (36.0) | 26 (36.6) | 0.005 | 0.944 |
β-blocker | 11 (22.0) | 17 (23.9) | 0.062 | 0.803 |
ACEI/ARB | 20 (40.0) | 30 (42.3) | 0.061 | 0.804 |
CCB | 19 (38.0) | 25 (35.2) | 0.099 | 0.754 |
Insulin | 4 (8.0) | 11 (15.5) | 1.517 | 0.218 |
Treatment at discharge | ||||
Anti-platelet | 48 (96.0) | 71 (100.0) | 2.888 | 0.089 |
Statins | 47 (94.0) | 70 (98.6) | 1.935 | 0.164 |
β-blocker | 15 (30.0) | 20 (28.2) | 0.048 | 0.827 |
ACEI/ARB | 21 (42.0) | 30 (42.3) | 0.001 | 0.978 |
CCB | 17 (34.0) | 21 (29.6) | 0.266 | 0.606 |
Insulin | 5 (10.0) | 12 (16.9) | 1.157 | 0.282 |
LTL, kb | 15.32±3.72 | 12.54±2.70 | −4.521 | <0.001 |
Values were represented by mean ± SD, or n (%). PCI, percutaneous coronary intervention; DM, diabetes mellitus; TC, total cholesterol; HDL-C, high density lipoprotein cholesterol; LDL-C, low density lipoproteins cholesterol; TG, triglyceride; WBC, white blood cell; CRP, C-reactive protein; Cr, creatinine; ACEI, angiotensin-converting enzyme inhibitors; ARB, angiotensin receptor blockers; CCB, calcium channel blocker; LTL, leukocyte telomere length.
Next, we characterized target lesions using OCT imaging (Figure 2) and results are summarized in Table 2. There was no significant difference in treated vessel location (P=0.232), incidence of lipid rich plaque (LRP) (88.7% vs. 78.0%, P=0.11), microchannel (40.8% vs. 26.0%, P=0.091), fissure (19.7% vs. 8.0%, P=0.074) between the PCI group and non-PCI group. However, lipid length (17.17±9.94 vs. 12.21±10.15 mm, P=0.01), mean lipid arc (213.80±96.79 vs. 137.71±109.87°, P<0.001), and lipid index (4,286.82±3,012.54 vs. 2,444.87±2,677.59 °*mm, P=0.001) were greater in the PCI group than the non-PCI group. TCFA (36.6% vs. 16.0%, P=0.013), macrophages accumulation (56.3% vs. 38.0%, P=0.047), plaque rupture (23.9% vs. 6.0%, P=0.009), cholesterol crystal (49.3% vs. 30.0%, P=0.034), and dissection (23.9% vs. 4.0%, P=0.003) were more common in the PCI group compared to the non-PCI group.
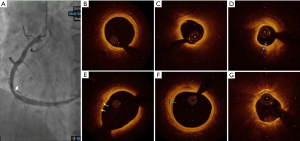
Table 2
Variables | Non-PCI (n=50) | PCI (n=71) | t/U/χ2 value | P value |
---|---|---|---|---|
Vessel location | 2.924 | 0.232 | ||
LAD, n (%) | 35 (70.0) | 39 (54.9) | ||
LCX, n (%) | 5 (10.0) | 9 (12.7) | ||
RCA, n (%) | 10 (20.0) | 23(32.4) | ||
MLA, mm2 | 3.73±2.00 | 2.93±2.31 | −2.000 | 0.048 |
DS% | 53.65±16.71 | 61.11±18.01 | 2.312 | 0.023 |
Qualitative OCT analysis | ||||
Rupture, n (%) | 3 (6.0) | 17 (23.9) | 6.847 | 0.009 |
Macrophages, n (%) | 19 (38.0) | 40 (56.3) | 3.949 | 0.047 |
Microchannel, n (%) | 13 (26.0) | 29 (40.8) | 2.853 | 0.091 |
ChCs, n (%) | 15 (30.0) | 35 (49.3) | 4.505 | 0.034 |
Fissure, n (%) | 4 (8.0) | 14 (19.7) | 3.182 | 0.074 |
Dissection, n (%) | 2 (4.0) | 17 (23.9) | 8.816 | 0.003 |
LRP, n (%) | 39 (78.0) | 63 (88.7) | 2.553 | 0.11 |
TCFA, n (%) | 8 (16.0) | 26 (36.6) | 6.174 | 0.013 |
Calcification, n (%) | 29 (58.0) | 23 (32.4) | 7.849 | 0.005 |
Quantitative OCT analysis | ||||
Lesion length, mm | 8.41±7.81 | 21.72±9.67 | 2.005 | 0.047 |
Lipid length, mm | 12.21±10.15 | 17.17±9.94 | 2.624 | 0.01 |
Mean lipid arc, ° | 137.71±109.87 | 213.80±96.79 | 4.026 | <0.001 |
Lipid index, °* mm | 2,444.87±2,677.59 | 4,286.82±3,012.54 | 3.465 | 0.001 |
FCT, μm | 106.06±36.63 | 76.19±19.02 | −4.554 | <0.001 |
Values were represented by mean ± SD, or n (%). PCI, percutaneous coronary intervention; LAD, left anterior descending coronary artery; LCX, left circumflex artery; RCA, right coronary artery; MLA, minimal lumen area; DS, diameter stenosis; OCT, optical coherence tomography; ChCs, cholesterol crystals; LRP, lipid rich plaque; TCFA, thin-cap fibroatheroma; lipid index, mean lipid arc * lipid length; mean lipid arc: the lipid arc was measured at 1-mm intervals throughout the length of lesion and the averaged value was mean lipid arc; FCT, fibrous cap thickness.
Possible influencing factors in PCI, such as age, sex, diabetes and CHD family history, LDL levels, were assessed using logistic regression modeling (Table 3). In univariate analysis, sex, diabetes, LDL levels, CHD family history, and LTL were significantly associated with PCI. Both univariate and multivariate logistic regression analyses showed that LTL was significantly associated with PCI in intermediate coronary lesion patients. In multivariate analysis, LTL was associated with PCI with an odds ratio (OR) of 0.952 [95% confidence interval (CI): 0.930–0.974, P<0.001]. These data suggest that LTL is an independently protective factor for determining if an intermediate coronary lesion patient requires PCI intervention.
Table 3
Variables | Univariable analysis OR (95% CI) | P value | Multivariable analysis OR (95% CI) | P value |
---|---|---|---|---|
Age | 0.987 (0.952–1.022) | 0.451 | 0.972 (0.931–1.016) | 0.206 |
Male | 2.15 (0.999–4.629) | 0.05 | 3.029 (1.177–7.790) | 0.022 |
Smokers | 0.575 (0.277–1.194) | 0.137 | 0.586 (0.210–1.631) | 0.306 |
Diabetes | 2.309 (1.016–5.248) | 0.046 | 3.798 (1.305–11.055) | 0.014 |
Family history | 0.434 (0.207–0.909) | 0.027 | 0.573 (0.214–1.535) | 0.268 |
LDL-C | 1.5 (1.022–2.202) | 0.038 | 1.286 (0.796–2.075) | 0.304 |
LTL | 0.958 (0.938–0.978) | <0.001 | 0.952 (0.930–0.974) | <0.001 |
In the multivariable adjusted models, all dependent variables were adjusted for each other. PCI, percutaneous coronary intervention; OR, odd ratio; CI, confidence interval; LDL-C, low-density lipoproteins-cholesterol; LTL, leukocyte telomere length.
To determine the sensitivity and specificity of LTL for predicting whether a patient with intermediate coronary lesion need PCI or not, we performed ROC analysis (Figure 3). The area under the ROC curve (AUC) of LTL was 0.714 (95% CI: 0.619–0.808 P<0.001). Based on the ROC curve analysis, the optimal cut-off value was 13.45 kb, and the sensitivity and specificity were 72.0% and 62.0%, respectively (Table 4).
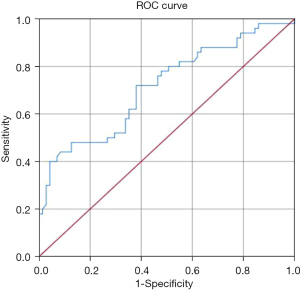
Table 4
LTL (kb) | Sensitivity % | Specificity % | DOC |
---|---|---|---|
11.29 | 0.880 (0.832–0.928) | 0.451 (0.403–0.499) | 0.3338 |
12.41 | 0.80 (0.752–0.848) | 0.493 (0.445–0.541) | 0.2970 |
13.45 | 0.720 (0.672–0.768) | 0.620 (0.572–0.668) | 0.2228 |
13.81 | 0.620 (0.572–0.668) | 0.648 (0.600–0.696) | 0.2683 |
14.51 | 0.500 (0.452–0.548) | 0.732 (0.684–0.780) | 0.3218 |
AUC =0.714 (95% CI: 0.619–0.808), P<0.001. ROC, receiver operating characteristic; LTL, leukocyte telomere length; DOC, distance on curve equaling square root of (1-Sen)2+(1-Spe)2; AUC, area under the ROC curve.
Furthermore, LTL was inversely correlated with lipid length (r =−0.190, P=0.037), lipid arc (r =−0.301, P=0.001), lipid index (r =−0.182, P=0.046), and positive correlation with FCT (r =0.213, P=0.034) (Table 5).
Table 5
LTL | Lipid length | Lipid arc | Lipid index | FCT |
---|---|---|---|---|
r | −0.190 | −0.301 | −0.182 | 0.213 |
P value | 0.037 | 0.001 | 0.046 | 0.034 |
LTL, leukocyte telomere length; FCT, fibrous cap thickness.
During the 24 months follow-up period, the total incidence of MACE was 3.3% (4/121). There was no death and AMI in both groups. Two patients suffered from TLR in the Non-PCI group (2/50, 4%) and two patients experienced TLR in the PCI group (2/71, 2.8%). There was no significant difference between the two groups (P=0.84) (Figure 4).
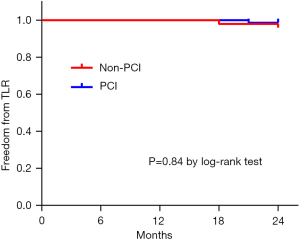
Discussion
In this study, patients who receive PCI treatment had shorter LTL than patients who didn’t need PCI. Multivariate analysis suggest that LTL is an independently protective factor for determining if an intermediate coronary lesion patient requires PCI intervention. Moreover, we also found that shorter LTL was associated with increased incidence of plaque instability measured by OCT. LTL is inversely associated with lipid length, lipid arc and lipid index. There was no significant difference in total incidence of MACE between the two groups during our 24 months follow-up.
Major international clinical guidelines on myocardial revascularization support the use of physiological assessment, such as fractional flow reserve (FFR), to assess the hemodynamic relevance of intermediate-grade stenosis. Other non-invasive diagnostic tools, such as myocardial scintigraphy, myocardial contrast echocardiography, or single-photon emission CT (SPECT) can also be used to assessment of myocardial ischemia (18,19). However, these tests are often cost-prohibitive for patients and large-scale prospective studies are warranted. In contrast, optical coherence tomography (OCT), has clearly demonstrated a favorable impact on PCI optimization (20) and a comparison between FFR and OCT shows that OCT guidance is associated with lower occurrence of the composite of major adverse cardiac events or significant angina and FFR guidance is associated with a higher rate of medical management after a follow up of 13 months (21). It provided support proof that OCT may be as an assessment tool for decision of intermediate coronary lesions. In this retrospective study, we used OCT guidance for PCI treatment.
Studies of LTL and cardio-metabolic outcomes have yielded conflicting results. D’Mello et al. (22) found a significant association between shortened LTL and myocardial infarction; however, Haycock et al. (23) also showed LTL inversely associated with non-fatal myocardial infarction and coronary heart disease deaths. Paradoxically, both studies did not find a significant association between LTL and coronary artery disease when measured as a secondary outcome defined by angina and nonfatal ischemic heart diseases. Recently, a study which assessed telomere length in circulating leukocytes from 1,459 volunteers without established cardiovascular disease from the PESA study, indicated that average LTL and short telomere load are not significant independent determinants of subclinical atherosclerosis (24). Remarkably, in a 10 years prospective study, in an atherosclerosis progression model, LTL was strongly associated with advanced, but not early, atherogenesis (25). Together, it is thought that short LTL most likely does not initiate atherosclerosis but rather is associated with plaque progression and ischemic events due to plaque instability. In our study, we used OCT as the gold standard to quantify plaque components in coronary arterial walls (14). Consistent with above study, our study showed a direct correlation between LTL and plaque instability. Given plaque instability is significantly associated with ischemic events, our results showing that short LTL is highly correlated with plaques instability is consistent with previous study.
In a large-scale genome-wide association meta-analysis, several alleles were shown to be significantly associated with shorter LTL with increased risk of CHD after adjustment for other CHD risk factors (26). Madrid et al. used a Mendelian randomization approach demonstrating that shorter telomeres are associated in the development of ischemic heart disease, independent of conventional cardiovascular risk factors (27). The mechanism behind a possible causal relationship between short telomere length and ischemic heart disease has not been fully elucidated. Telomere shortening, often associated with inflammation, may contribute to atherosclerosis through cellular senescence. It has been shown that the accumulation of senescent cells, a prominent feature of atherosclerotic plaques, reduces the regenerative potential of affected tissues, promotes apoptosis, further exacerbates inflammation, and results in endothelial dysfunction (28). It has also been hypothesized that senescent vascular smooth muscle cells results in blocked proliferative capacity and leads to fibrous caps thinning and plaque instability (29). Here, our study show that LTL is significant associate with FCT, lipid length, lipid arc and lipid index and together speculate impaired regenerative capacity of vasculature and results in atherogenesis.
In a recent study, Demanelis et al. (30) measured relative telomere length of 6,391 unique tissue samples in more than 25 tissue types from 952 donors, and they show that LTL is well correlated to organ telomere length across human tissue types, and suggests LTL as a proxy aging. Their finding supports the use of LTL as a proxy for estimating biological aging in large epidemiological studies. Using skeletal muscle telomere length as a reference point, Benetos et al. (31) show that short LTL is associated with increased atherosclerosis propensity. In accordance to these findings, we observed short LTL is significantly associated with plaque instability as measured by OCT imaging. Although a larger study is warranted, our LTL data suggests its usefulness as a risk prediction assay. The DNA extraction and PCR reaction procedures are relative standardized and clinical implementation at the time of admission, prior to surgery, may facilitate decision making. LTL can be combined with other risk factors to provide superior risk stratification. For high-risk patients, OCT, IVUS, or FFR may be second line options if angiography shows intermediate coronary lesions. Those patients should be followed up more closely and have more rigorous lipid management whether underwent PCI or not.
Study limitations
Several potential limitations should be acknowledged. First, this study was a retrospectively analyzed performed in a single university hospital, and the study population was small. Second, only patients who underwent OCT-guided PCI were included, so selection bias may have occurred. Third, systematic 3-vessel imaging was not performed to assess nonculprit plaques. However, in real-world practice, 3-vessel imaging is impractical and may expose patients to unjustified additional risk. Fourth, only plaques near target lesion were enrolled. Another type of selection bias may have occurred. A prospective large-scale study will need in future.
Conclusions
These data recognize a correlation between LTL and whether patients needed PCI. We may suggest LTL as a biomarker to provide indication recommendations for intermediate coronary lesion patients. This study also show correlation between LTL and plaque instability and may provide some information about the association of aging and atherosclerosis. As discussed, in one hand, it would be important to validate our findings in a larger cohort and carry out longitudinal follow-up studies. In another hand, the relationship between aging and plaque instability can be deeply researched in laboratory.
Acknowledgments
Funding: This work was supported by research projects from Shanghai Science and Technology Commission [18411950500 to CQW]; the SHIPM-mu fund from Shanghai Institute of Precision Medicine, Ninth People’s Hospital Shanghai Jiao Tong University School of Medicine (jc201905 to ALZ); the National Natural Science Foundation of China (82070248 to ACYC) and the project of construction and application of biobank for coronary heart disease of Shanghai Ninth People’s Hospital (YBKA201910 to JFZ).
Footnote
Reporting Checklist: The authors have completed the STROBE reporting checklist. Available at https://apm.amegroups.com/article/view/10.21037/apm-21-2295/rc
Data Sharing Statement: Available at https://apm.amegroups.com/article/view/10.21037/apm-21-2295/dss
Peer Review File: Available at https://apm.amegroups.com/article/view/10.21037/apm-21-2295/prf
Conflicts of Interest: All authors have completed the ICMJE uniform disclosure form (available at https://apm.amegroups.com/article/view/10.21037/apm-21-2295/coif). The authors have no conflicts of interest to declare.
Ethical Statement: The authors are accountable for all aspects of the work in ensuring that questions related to the accuracy or integrity of any part of the work are appropriately investigated and resolved. The study was conducted in accordance with the Declaration of Helsinki (as revised in 2013). The study was approved by Ethics Committee of Ninth People's Hospital, Shanghai Jiao Tong University School of Medicine, Shanghai, China (No. SH9H-2021-T1-1). All individual consent for this retrospective analysis was waived.
Open Access Statement: This is an Open Access article distributed in accordance with the Creative Commons Attribution-NonCommercial-NoDerivs 4.0 International License (CC BY-NC-ND 4.0), which permits the non-commercial replication and distribution of the article with the strict proviso that no changes or edits are made and the original work is properly cited (including links to both the formal publication through the relevant DOI and the license). See: https://creativecommons.org/licenses/by-nc-nd/4.0/.
References
- Tobis J, Azarbal B, Slavin L. Assessment of intermediate severity coronary lesions in the catheterization laboratory. J Am Coll Cardiol 2007;49:839-48. [Crossref] [PubMed]
- Rzeczuch K, Szajn G, Jankowska E, et al. Borderline coronary lesions may lead to serious coronary events--long-term outcome in 65 conservatively treated patients. Kardiol Pol 2005;63:50-6; discussion 57. [PubMed]
- Glaser R, Selzer F, Faxon DP, et al. Clinical progression of incidental, asymptomatic lesions discovered during culprit vessel coronary intervention. Circulation 2005;111:143-9. [Crossref] [PubMed]
- Tomaniak M, Katagiri Y, Modolo R, et al. Vulnerable plaques and patients: state-of-the-art. Eur Heart J 2020;41:2997-3004. [Crossref] [PubMed]
- Kostis WJ, Deng Y, Pantazopoulos JS, et al. Trends in mortality of acute myocardial infarction after discharge from the hospital. Circ Cardiovasc Qual Outcomes 2010;3:581-9. [Crossref] [PubMed]
- Fujiyoshi K, Minami Y, Ishida K, et al. Incidence, factors, and clinical significance of cholesterol crystals in coronary plaque: An optical coherence tomography study. Atherosclerosis 2019;283:79-84. [Crossref] [PubMed]
- Uemura S, Ishigami K, Soeda T, et al. Thin-cap fibroatheroma and microchannel findings in optical coherence tomography correlate with subsequent progression of coronary atheromatous plaques. Eur Heart J 2012;33:78-85. [Crossref] [PubMed]
- Stone GW, Maehara A, Lansky AJ, et al. A prospective natural-history study of coronary atherosclerosis. N Engl J Med 2011;364:226-35. [Crossref] [PubMed]
- Cheng JM, Garcia-Garcia HM, de Boer SP, et al. In vivo detection of high-risk coronary plaques by radiofrequency intravascular ultrasound and cardiovascular outcome: results of the ATHEROREMO-IVUS study. Eur Heart J 2014;35:639-47. [Crossref] [PubMed]
- Shah PK. Mechanisms of plaque vulnerability and rupture. J Am Coll Cardiol 2003;41:15S-22S. [Crossref] [PubMed]
- Prati F, Regar E, Mintz GS, et al. Expert review document on methodology, terminology, and clinical applications of optical coherence tomography: physical principles, methodology of image acquisition, and clinical application for assessment of coronary arteries and atherosclerosis. Eur Heart J 2010;31:401-15. [Crossref] [PubMed]
- Blackburn EH, Epel ES. Telomeres and adversity: Too toxic to ignore. Nature 2012;490:169-71. [Crossref] [PubMed]
- Blackburn EH, Epel ES, Lin J. Human telomere biology: A contributory and interactive factor in aging, disease risks, and protection. Science 2015;350:1193-8. [Crossref] [PubMed]
- Tearney GJ, Regar E, Akasaka T, et al. Consensus standards for acquisition, measurement, and reporting of intravascular optical coherence tomography studies: a report from the International Working Group for Intravascular Optical Coherence Tomography Standardization and Validation. J Am Coll Cardiol 2012;59:1058-72. [Crossref] [PubMed]
- Burzotta F, Leone AM, De Maria GL, et al. Fractional flow reserve or optical coherence tomography guidance to revascularize intermediate coronary stenosis using angioplasty (FORZA) trial: study protocol for a randomized controlled trial. Trials 2014;15:140. [Crossref] [PubMed]
- Sinclair H, Bourantas C, Bagnall A, et al. OCT for the identification of vulnerable plaque in acute coronary syndrome. JACC Cardiovasc Imaging 2015;8:198-209. [Crossref] [PubMed]
- Yabushita H, Bouma BE, Houser SL, et al. Characterization of human atherosclerosis by optical coherence tomography. Circulation 2002;106:1640-5. [Crossref] [PubMed]
- Neumann FJ, Sousa-Uva M, Ahlsson A, et al. 2018 ESC/EACTS Guidelines on myocardial revascularization. Eur Heart J 2019;40:87-165. [Crossref] [PubMed]
- Levine GN, Bates ER, Blankenship JC, et al. 2011 ACCF/AHA/SCAI Guideline for Percutaneous Coronary Intervention: a report of the American College of Cardiology Foundation/American Heart Association Task Force on Practice Guidelines and the Society for Cardiovascular Angiography and Interventions. Circulation 2011;124:e574-651. [PubMed]
- Jones DA, Rathod KS, Koganti S, et al. Angiography Alone Versus Angiography Plus Optical Coherence Tomography to Guide Percutaneous Coronary Intervention: Outcomes From the Pan-London PCI Cohort. JACC Cardiovasc Interv 2018;11:1313-21. [Crossref] [PubMed]
- Burzotta F, Leone AM, Aurigemma C, et al. Fractional Flow Reserve or Optical Coherence Tomography to Guide Management of Angiographically Intermediate Coronary Stenosis: A Single-Center Trial. JACC Cardiovasc Interv 2020;13:49-58. [Crossref] [PubMed]
- D'Mello MJ, Ross SA, Briel M, et al. Association between shortened leukocyte telomere length and cardiometabolic outcomes: systematic review and meta-analysis. Circ Cardiovasc Genet 2015;8:82-90. [Crossref] [PubMed]
- Haycock PC, Heydon EE, Kaptoge S, et al. Leucocyte telomere length and risk of cardiovascular disease: systematic review and meta-analysis. BMJ 2014;349:g4227. [Crossref] [PubMed]
- Fernández-Alvira JM, Fuster V, Dorado B, et al. Short Telomere Load, Telomere Length, and Subclinical Atherosclerosis: The PESA Study. J Am Coll Cardiol 2016;67:2467-76. [Crossref] [PubMed]
- Willeit P, Willeit J, Brandstätter A, et al. Cellular aging reflected by leukocyte telomere length predicts advanced atherosclerosis and cardiovascular disease risk. Arterioscler Thromb Vasc Biol 2010;30:1649-56. [Crossref] [PubMed]
- Codd V, Nelson CP, Albrecht E, et al. Identification of seven loci affecting mean telomere length and their association with disease. Nat Genet 2013;45:422-7, 427e1-2.
- Scheller Madrid A, Rode L, Nordestgaard BG, et al. Short Telomere Length and Ischemic Heart Disease: Observational and Genetic Studies in 290 022 Individuals. Clin Chem 2016;62:1140-9. [Crossref] [PubMed]
- Matthews C, Gorenne I, Scott S, et al. Vascular smooth muscle cells undergo telomere-based senescence in human atherosclerosis: effects of telomerase and oxidative stress. Circ Res 2006;99:156-64. [Crossref] [PubMed]
- Gorenne I, Kavurma M, Scott S, et al. Vascular smooth muscle cell senescence in atherosclerosis. Cardiovasc Res 2006;72:9-17. [Crossref] [PubMed]
- Demanelis K, Jasmine F, Chen LS, et al. Determinants of telomere length across human tissues. Science 2020;369:eaaz6876. [Crossref] [PubMed]
- Benetos A, Toupance S, Gautier S, et al. Short Leukocyte Telomere Length Precedes Clinical Expression of Atherosclerosis: The Blood-and-Muscle Model. Circ Res 2018;122:616-23. [Crossref] [PubMed]