Intravenous iron injection does not impair liver function in a rat model of cirrhosis
Introduction
Chronic liver disease is often accompanied by anemia, which occurs in approximately 75% of patients with advanced liver disease (1) and is attributed to multifactorial causes including bone marrow suppression due to viruses or ethanol use, renal insufficiency, and variceal bleeding (2-4). Iron homeostasis is the result of a complex mechanism, which may be substantially disturbed in patients with chronic liver disease of different etiologies and stages. Iron deficiency (ID) anemia is often documented, especially in patients with advanced liver disease and portal hypertension, and is usually due to chronic blood loss (5). ID is a potentially treatable cause of anemia and several studies have shown benefits of iron treatment for patient outcomes after various surgeries (6,7). Ferric carboxymaltose is a recently developed intravenous iron supplement (8,9), which can be administered as a single high dose within 15 minutes and cause rapid hemoglobin elevation. Because of the relative safety profile of ferric carboxymaltose compared with other intravenous iron drugs, it might be suitable for the treatment of patients with liver disease (10).
To our knowledge, there has been minimal evidence concerning the safety of iron therapy in patients with end-stage liver disease. Moreover, preoperative iron therapy has not been established in patients undergoing hepatectomy or liver transplantation because of concerns regarding excessive iron overload and the potentially negative clinical outcomes associated with iron accumulation in the livers of patients with cirrhosis (11,12). Therefore, in patients with chronic liver disease, the impact of exogenous iron supplementation on the liver should be predetermined before using iron in clinical practice. In particular, there is a need to determine whether the contributions of enhanced hepatic iron deposition can accelerate liver fibrotic progression or exacerbate inflammation and oxidative stress. Previous animal studies demonstrated that iron hepatotoxicity may be less frequent than is commonly assumed, and most animal models of chronic iron overload failed to show substantial fibrosis when iron was the only stressor (10,13,14). However, no pre-clinical or clinical trials have evaluated the safety and efficacy of intravenous iron treatment in the context of various liver diseases. Therefore, we designed this preliminary experimental study to investigate the effects of intravenous iron supplements on the liver in a rat model of cirrhosis. We present the following article in accordance with the ARRIVE reporting checklist (available at https://apm.amegroups.com/article/view/10.21037/apm-21-3039/rc).
Methods
Study design and treatment
In total, 55 male Sprague-Dawley rats aged 5 weeks and weighing 140–160 g were housed in an animal experimental unit under 23±3 ℃, 55%±15% relative humidity, 10–20 cycles/hour ventilation frequency, 12-hour light/dark cycle, and 150–300 lux. During the experimental period, environmental conditions were assessed regularly, and no changes were observed that would presumably affect the test results. Dreambio standard rat chow (Cargill Agripurina Co., Ltd., Seoul, South Korea) and water from a polycarbonate drinking bottle were provided ad libitum. A wooden rug (Cotec Co., Gyeonggi-do, South Korea) was also supplied. During the acclimatization and testing periods, no more than five animals were housed in each polycarbonate cage (W × L × H: 235 mm × 380 mm × 175 mm). The breeding boxes, rugs, and water bottles were changed at least once weekly. Animals determined to be healthy during the acclimation period were weighed and distributed randomly; thus, the average weight of each group was evenly distributed. All experimental animal protocols and animal procedures complied with the Guide for the Care and Use of Laboratory Animals (National Academy of Sciences NIH Publication 86-23, revised 1985) (15).
Pilot study for conformation of liver cirrhosis (LC)
Figure 1 shows the overall study design including pilot study and main experiment. Carbon tetrachloride (CCl4) was dissolved in purified olive oil to a concentration of 50% and administered twice weekly via intraperitoneal injection (2 mL/kg) (16). Blood samples were obtained at 6–10 weeks, and aspartate aminotransferase (AST) and alanine aminotransferase (ALT) levels were measured to check the state of hepatocellular injury. Blood samples were collected through the tail vein and serum was centrifuged at 3,000 rpm for 10 minutes, and AST and ALT levels were measured using a blood biochemical analyzer and an automatic analyzer. Liver fibrosis was confirmed by gross histopathological examination based on liver sample collection from 10 rats (two rats per week; these rats were not included in the grouped analyses described below). Sirius red staining was also performed to confirm cirrhosis. Following the administration of experimental and control substances, CCl4 injection was continued twice weekly until euthanasia to maintain the cirrhotic state of the liver in all animals.
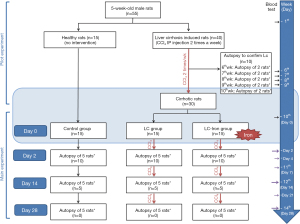
Main experiment
Forty-five rats were distributed among three groups. Fifteen normal, healthy rats without cirrhosis were maintained as a baseline control group. Of the 30 remaining rats (all with cirrhosis), 15 continued to receive CCl4 twice weekly with intravenous saline (i.e., the LC group), and 15 continued to receive CCl4 in combination with Ferinject iron treatment [i.e., the liver cirrhosis iron (LC-iron) group]. The investigators, except the principal investigator, were blinded to the animal treatments. Ferinject is a recently developed intravenous iron supplement that contains ferric carboxymaltose as its main component (8,9). We used Ferinject because it showed benefits for liver, heart, and kidney function compared with other ferric carboxymaltose agents In rat models (10) (Figure 1).
After confirmation of LC, animals in the LC-iron group were fixed in a calibrated frame, and intravenous iron was slowly injected at a rate of 1 mL/min through the tail vein using a syringe with a 26-gauge needle. The dose contained 20 mg iron/kg body weight, in the form of Ferinject diluted with normal saline. The dose was adjusted for each animal according to body weight. This drug was administered only once as the manufacturer suggested. Experiments were performed under a project license (certificate No. IACUC 18-KE-327) granted by institutional board of KNOTUS Co., Ltd., Incheon-si, Korea, in compliance with KOTUC Co., Ltd. laboratory institutional guidelines for the care and use of animals.
Autopsy
At 2, 14, and 28 days after iron administration, five animals from each group were anesthetized using ether. Blood was collected via posterior vena cava puncture, and the animals were euthanized by exsanguination. Liver tissues were harvested, and the right lobe was divided into two sections, of which one was frozen at −70 ℃, and the other was fixed in 10% neutral buffered formalin. The entire left lobe was fixed in 10% neutral buffered formalin solution. On days 14 and 28, portal vein pressure was measured by direct cannulation using a 26-gauge needle before blood collection.
Biochemistry
Blood samples were collected before injection, as well as at 2, 4, 7, 14, 21, and 28 days after iron administration. Complete blood count vials containing EDTA-2K (anticoagulant, ADVIA 2120, Siemens, USA) were used to collect samples for hemoglobin and mean corpuscular volume measurements.
Portions of the blood collected before iron treatment and at 2, 4, 7, 14, 21, and 28 days were retained in a vacutainer tube containing a clot activator and incubated for 15–20 minutes at room temperature. Serum was centrifuged at 3,000 rpm for 10 minutes, and AST and ALT levels were measured using a blood biochemical analyzer and an automatic analyzer.
Histology and quantification of fibrosis
Extracted liver tissues were fixed, embedded in paraffin, sectioned, and stained using hematoxylin-eosin and Prussian blue to identify fibrosis and iron deposits, respectively. Histopathological changes were observed using an optical microscope (BX53, Olympus, Japan). Morphological examinations were performed at a magnification of ×100. In all instances, two independent observers performed blinded evaluations, and mean percentages were calculated. Histochemical localization in the liver tissue of each rat was calculated using a computer and image analyzer and expressed as the percentage of the area per millimeter of positive iron deposit staining. Liver cell fibrosis was scored from 0 to 4 (17): 0 = no sign of fibrosis or cirrhosis; 1 = enlarged, fibrotic portal tracts; 2 = periportal or portal–portal septa but intact architecture; 3 = fibrosis with architectural distortion but no obvious cirrhosis; and 4 = probable or definite cirrhosis. Iron accumulation was scored from 1 to 2 (18).
Chemicals and reagents
Stored blood samples and liver tissue samples collected on days 2, 14, and 28 were further analyzed using enzyme-linked immunosorbent assays and other assay kits. Serum was isolated and analyzed for the levels of total iron binding capacity, serum iron (cat. no. ab239715, Abcam, USA), ferritin (cat. no. ab157732, Abcam), C-reactive protein (cat. no. ab260058, Abcam), and hepcidin (cat. no. CSB-EL0 101 24RA, Cusabio, USA). Proteins were isolated from liver tissues to measure the levels of malondialdehyde (MDA) (cat. no. ab238537, Abcam), tumor necrosis factor-α (TNF-α) (cat. no. ab100785, Abcam), and interleukin-6 (IL-6) (cat. no. ab100772, Abcam). The absorbance was measured using a microplate spectrophotometer (Epoch, BioTek, USA).
Statistical analysis
The normality of the data distribution was assessed using the Kolmogorov-Smirnov test. For assessing differences among groups, data with a normal distribution were compared using one-way analysis of variance with the Bonferroni post-hoc test and data without a normal distribution were compared using the Friedman test with the Dunn post-hoc test. Quantitative variables are expressed as medians (interquartile range) or means (standard deviation), and qualitative variables are expressed as proportions (%), unless otherwise noted. A P value <0.05 was considered statistically significant. Analyses were performed using IBM SPSS Statistics version 21 or GraphPad Prism.
Results
Pilot study to confirm of liver fibrosis
Ten weeks after the initiation of CCl4 administration, AST levels were significantly higher in the LC (443±110 IU/L) and LC-iron (444±117 IU/L) groups than in the control group (69±7 IU/L, P<0.001). ALT levels were also significantly higher in the LC (518±186 IU/L) and LC-iron (520±252 IU/L) groups than in the control group (91±7 IU/L, P<0.001). Histological analyses showed disrupted liver architecture with fibrotic bands, parenchymal nodules, and vascular distortion, which implied LC. Sirius red staining showed significantly greater levels of fibrosis in the LC and LC-iron groups than in the control group.
Main experiment: liver fibrosis and iron deposition
Histological examination indicated that after CCL4 administration, there were significantly greater degrees of fibrosis in the LC and LC-iron groups than in the control group (Figure 2A). The level of iron accumulation was significantly greater in the LC-iron group than in the LC group at all measurement points (Figure 2B). The degrees of fibrosis were comparable between the LC-iron and LC groups on days 2, 14, and 28 (P=1.0, P=0.25, and P=1.0, respectively). The degree of hepatic iron accumulation was significantly greater in the LC-iron group than in the LC group on days 2 (P<0.001), 14 (P=0.029), and 28 (P<0.01). Histopathological examination indicated ballooning cells, fatty changes, fibrosis, and iron accumulation in liver tissue (Figure 2). Focal iron accumulation was observed in the portal triad in the LC group, but diffuse accumulation was present in the hepatic triad and liver lobules in the LC-iron group. Iron deposition (visualized using Prussian blue staining) was mainly deposited to reticuloendothelial cells in the LC-iron group but showed mixed distribution in non-reticuloendothelial hepatocytes and reticuloendothelial cells in LC group.
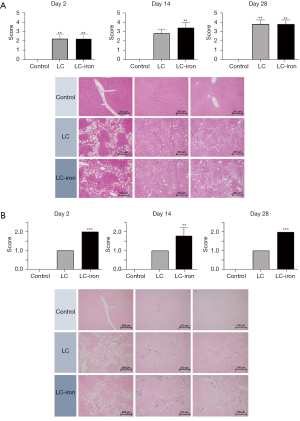
Portal vein pressure
Portal vein pressure was higher in the LC and LC-iron groups than in the control group. On days 14 and 28, there were no significant differences between the LC-iron and LC groups (P=0.25 on day 14 and P=0.35 on day 28; Figure 3).
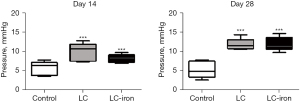
Liver function test
During the test period, AST and ALT levels were significantly higher in both the LC and LC-iron groups than in the control group (P<0.01). Mean AST and ALT levels of control group throughout the main experiment were 98.4±14.9 and 75.9±12.7 IU/L, respectively. It was negligible to be showed in the graph to put together with LC and LC-iron group so we did not show the control group in the Figure 4. AST/ALT levels were not significantly different between the LC and LC-iron groups at 0, 2, 14, or 28 days (Figure 4). ALT levels on days 4 and 7 (P<0.03 and P<0.001) and AST level on day 7 (P<0.001) after treatment, were significantly lower in the LC-iron group than in the LC group (Figure 4).
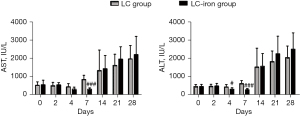
Levels of ID anemia-related parameters
Prior to intravenous iron administration, anemia was observed in both the LC and LC-iron groups, and the hemoglobin level was comparable between these two groups (12.2±1.4 vs. 12.2±1.6 g/dL, P=0.87). Mean corpuscular volume was also comparable between the LC and LC-iron groups (56.2±4.7 vs. 56.8±5.3 fL, P=0.76), which implied microcytic anemia due to ID or chronic disease. There were no significant differences between the LC and LC-iron groups in terms of other hematological measures.
On days 2, 4, 7, and 14 after Ferinject administration, there were no significant differences between the LC and LC-iron groups in terms of the hemoglobin level (12.9±1.7 vs. 12.9±2.0 g/dL, P=0.66 on day 2; 12.6±2.3 vs. 12.6±0.9 g/dL, P=0.19 on day 4; 12.0±2.3 vs. 12.7±1.3 g/dL, P=0.34 on day 7; and 13.0±0.8 vs. 13.1±1.1 g/dL, P=0.60 on day 14; Table 1) and other hematological measures. Furthermore, no difference in the hemoglobin level between the LC and LC-iron groups was observed on day 21 (14.2±0.8 vs. 14.6±0.3 g/dL, P=0.17). The serum ferritin level was significantly lower (P=0.045), but the C-reactive protein level higher (P=0.076), in the LC-iron group than in the LC group on days 2 and 14 (Table 1). The serum iron level was comparable between the LC-iron and LC groups across all time points measured (Table 1). On day 2, the serum total iron binding capacity was significantly higher in the LC-iron group than in the LC group, but the levels in both groups were below the normal range (P=0.047). This difference did not remain on day 14. Transferrin saturation was not significantly different between the groups (Table 1).
Table 1
Days | Group | ||
---|---|---|---|
Control | LC | LC-iron | |
Hemoglobin (g/dL) | |||
2 | 15.4±0.5 | 12.0±2.3*** | 12.7±1.3** |
14 | 16.0±0.4 | 13.0±0.8*** | 13.1±1.1*** |
28 | 17.3±0.2 | 14.9±0.8*** | 15.6±0.7*** |
Ferritin (ng/mL) | |||
2 | 18.25±8.05 | 65.83±16.50*** | 45.44±7.87**# |
14 | 20.32±4.83 | 70.27±21.17*** | 37.92±15.09# |
28 | 23.27±9.31 | 86.47±19.72*** | 71.32±14.50*** |
Transferrin saturation (%) | |||
2 | 64.29±5.00 | 65.54±12.23 | 55.75±5.44 |
14 | 57.66±3.73 | 59.54±8.88 | 67.07±8.58 |
28 | 61.50±17.46 | 64.96±3.84 | 68.65±14.23 |
C-reactive protein (µg/mL) | |||
2 | 270.37±23.42 | 668.11±42.99* | 652.87±7.57*** |
14 | 276.97±18.47 | 924.98±24.24* | 834.89±52.92*** |
28 | 262.66±15.17 | 852.26±81.65*** | 788.06±62.97***# |
Hepcidin (ng/mL) | |||
2 | 2.32±0.33 | 6.39±2.18* | 9.02±2.88*** |
14 | 1.76±0.44 | 4.41±1.70* | 6.21±1.69*** |
28 | 1.41±0.26 | 4.96±1.10*** | 6.64±1.38***# |
Serum iron (µmol/L) | |||
2 | 50.02±3.69 | 54.86±9.88 | 55.18±7.97 |
14 | 48.59±5.16 | 63.07±17.89 | 76.43±14.02* |
28 | 43.81±10.08 | 68.49±7.47 | 72.30±24.28* |
Total iron binding capacity (µmol/L) | |||
2 | 77.87±3.20 | 84.12±7.89 | 99.11±12.83**# |
14 | 84.12±3.98 | 105.89±26.22 | 113.64±11.57* |
28 | 72.04±7.15 | 105.37±8.19*** | 103.50±15.82** |
Data are shown as means ± standard deviation. Control group: normal, healthy rats without cirrhosis; LC group: liver cirrhosis induced rats continued to receive CCl4 twice weekly with intravenous saline at day 0; LC-iron group: liver cirrhosis induced rats continued to receive CCl4 twice weekly with administration of intravenous iron (20 mg/kg) at day 0; days 2, 14, and 28 reflect times after infusion of iron. */**/***, significant difference at p<0.05/p<0.01/p<0.001 compared with the control group; #, significant difference at p<0.05 compared with the LC group. CCl4, carbon tetrachloride; LC, liver cirrhosis.
On day 28 after Ferinject administration, the level of hemoglobin tended to be higher in the LC-iron group than in the LC group, but this difference was not statistically significant (15.6±0.7 vs. 14.9±0.8 g/dL, P=0.67). The serum ferritin level was not significantly different between the LC and LC-iron groups, but the C-reactive protein level was lower in the LC-iron group than in the LC group (P=0.009; Table 1). The level of serum hepcidin was higher in the LC-iron group than in the LC group, but this difference was significant only on day 28 (P=0.047; Table 1).
Levels of pro-inflammatory and oxidative stress markers
At all time points, TNF-α, IL-6, and MDA levels were significantly higher in the LC-iron group than in the control group. On days 2, 14, and 28, the level of TNF-α was significantly lower in the LC-iron group than in the LC group (P=0.009, P=0.047, and P=0.009, respectively; Figure 5). On days 2, 14, and 28, the level of IL-6 was also significantly lower in the LC-iron group than in the LC group (P=0.009, P=0.009, and P=0.028, respectively; Figure 5). The MDA level was comparable between the LC-iron and LC groups on day 2 but was significantly lower in the LC-iron group than in the LC group on days 14 and 28 (P<0.05, Figure 5).
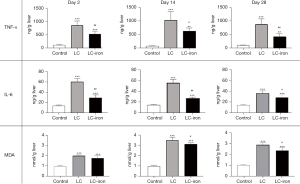
Discussion
Because there is limited evidence concerning the safety of supplementary iron in patients with LC, preoperative iron therapy has not been implemented in patients with end-stage liver disease to correct preoperative anemia, despite its benefits in other surgical settings. To our knowledge, this is the first experimental study to evaluate the hepatic effects of intravenous iron in a rat model of cirrhosis. The iron treatment did not induce significant changes in liver function, degree of fibrosis, or portal vein pressure, although there was substantial iron accumulation in the liver parenchyma after treatment. Notably, there were significantly lower degrees of inflammation and oxidative stress in liver tissues in the LC-iron group than in the LC group (no iron treatment). These results in a rat model of cirrhosis provide preclinical evidence that intravenous iron injection may not impair liver function or aggravate the degree of fibrosis, and that it may provide benefits in terms of inflammation and oxidative stress.
Safety of intravenous iron treatment
Because the liver plays a major role in iron metabolism, patients with hepatic disease often have abnormalities in iron homeostasis. The liver is the main organ that produces hepcidin, an iron regulatory hormone expressed under conditions of iron overload and during inflammation, which blocks the absorption of iron from enterocytes. In chronic liver disease, Hepcidin gene expression is altered, usually causing iron overload and leading to iron deposition in the liver, as well as higher levels of non-transferrin-bound iron in the bloodstream. However, no increase in the level of transferrin iron, which represents the normal form of circulating iron, is observed (19). Accordingly, paradoxical coexistence of iron excess and ID is observed in patients with liver disease. Liver fibrosis is a pathological stage in several chronic liver diseases (20), and excessive iron accumulation can accelerate the Fenton reaction. This reaction generates noxious reactive oxygen species and induces the expression of MDA and both pro-fibrinogenic and pro-inflammatory mediators (e.g., TNF-α and IL-6), leading to liver parenchymal damage and fibrosis. Recently, preoperative iron supplementation has been shown to correct anemia and improve clinical outcomes in patients with chronic diseases such as cancer, chronic renal failure, and end-stage liver disease (7,21,22). Thus, there is growing interest in both the clinical efficacy and safety of iron supplementation in patients with advanced liver disease.
The pathway of iron distribution and storage after supplementary iron injection is involved in hepatic toxicity. Storage of iron in the reticuloendothelial system (i.e., in Kupffer cells) represents appropriate deposition, which implies a reduced likelihood of oxidative damage (23). However, the detection of iron in non-reticuloendothelial hepatocytes within the liver, such as in parenchymal and sinusoidal epithelial cells, is considered abnormal and related to oxidative stress. In the present study, the degree of liver fibrosis was comparable after iron injection between the LC-iron and LC groups, although iron deposition was significantly higher in the LC-iron group. Iron deposition (visualized using Prussian blue staining) was restricted to reticuloendothelial cells in the LC-iron group, which implied that injected intravenous iron was deposited in the appropriate locations. Previous studies in rat models showed that Ferinject has a favorable safety profile in terms of liver function, oxidative stress level, and inflammatory responses, with appropriate deposition of iron in reticuloendothelial system cells in the liver (24-26). In accordance with these findings, despite the theoretical harmful impact of external iron injection on the diseased liver, the present study did not show a deleterious effect of iron, according to the liver function test results and comparison of fibrosis or portal vein pressure between LC-iron and LC groups. Our findings suggest that supplementary iron injection does not worsen preexisting LC. Moreover, Ferinject is a stable intravenous iron product that induces a lower level of labile iron, which might have contributed to this favorable profile in iron-treated rats (10).
In this study, significant reductions in ALT levels at days 4 and 7 and AST level at day 7 were observed in the LC-iron group after iron treatment, but had resolved by day 14. There are two possible underlying mechanisms. First, the effect of CCl4, which induces fibrosis, may have been suppressed because of the chemical interaction between CCl4 and intravenous iron. To rule out this possibility, we conducted a supplementary experiment examining whether CCl4 administered in conjunction with external iron could alter liver function tests. Our results showed that concomitant injections of the two agents did not result in significant reductions in ALT and AST levels, implying that no interaction occurred between these agents. Second, an elevated hepcidin level following iron injection may be related to reductions in AST and ALT levels. In our analysis, the hepcidin level was significantly greater in the LC-iron group than in the LC group, which can be explained by natural reaction following high dose of iron injection. Some of previous researches have described that an acute elevation of hepcidin can reduce inflammatory cytokine levels, thereby lowering the ferritin level. Urrutia et al. demonstrated that hepcidin pretreatment has the potential to relieve the damage caused by inflammatory signaling (27), via downregulation of IL-6 and TNF-α expression in astrocytes and microglia. This intracellular mechanism can thus protect neurons from oxidative stress. In addition, treatment with ad-hepcidin under non-inflammatory conditions can protect neurons from iron loading (27,28). Similarly, in the present study, there were significantly lower degrees of inflammation and oxidative stress in liver tissues in the LC-iron group than in the LC group and the significant reductions in ALT and AST levels might be attributed to concomitant decreases in the levels of inflammatory and oxidative stress markers. However, this is a speculative assumption since there are no data showing this relationship in the present study.
Efficacy of intravenous iron treatment for anemia
In this study, serum iron, total iron binding capacity, and transferrin saturation levels tended to be higher in the LC-iron group than in the LC group. Greater white blood cell count and reticulocyte in cirrhotic rats, combined with a significantly lower hemoglobin level, may have been caused by enhanced inflammation due to cirrhosis and abnormal hematopoietic function. The hemoglobin level was not significantly different between the LC-iron and LC groups throughout the experimental period, although it was slightly higher in the LC-iron group on day 28. Previous studies evaluating the efficacy of intravenous iron for treating anemia revealed that clinical and laboratory responses were typically observed within 2–3 weeks after treatment with intravenous iron, and improvements were expected to continue (29). However, we would not expect iron administration to have a major impact on the anemia since this is not a model that causes ID. In inflammatory states by liver disease, although serum levels of ferritin are high, iron is unable to circulate or be delivered to the bone marrow, which in turn leads to iron-restricted erythropoiesis (30,31). Previous study has made the evidence highlighting that preoperative ID, even in the absence of anemia is an important prognostic marker for postoperative mortality (32). Recent evidence of importance of absolute and functional ID in both anemic and non-anemic patients may validate the findings of the study.
Possible clinical implications
Preoperative anemia occurs frequently in patients undergoing surgery, and mild anemia can increase the requirement for allogeneic red blood cell transfusions, which are associated with various types of postoperative morbidity and mortality (32,33). Accordingly, patient blood management has been recently applied in clinical settings. The main components of patient blood management include optimizing erythrocyte mass, detecting anemia, managing underlying causal disorders, and treating suboptimal iron stores, ID, chronic disease-related anemia, and iron-restricted erythropoiesis. These considerations are especially important in high-risk patients with chronic liver disease who are undergoing various surgeries (e.g., hepatectomy and liver transplantation). Notably, heavy blood loss is frequently expected during and after surgery because of impaired coagulation, presence of a rich portosystemic collateral system, and surgical procedures involving major vessels (34,35). In patients with end-stage liver disease, blood transfusion can lead to major organ dysfunction related to a greater circulating blood volume and dilutional coagulation disorders (36). Accordingly, efforts to treat preoperative anemia are focused on reducing red blood cell transfusions (37). Several case reports have demonstrated that preoperative administration of intravenous iron to Jehovah’s Witness patients with end-stage liver disease enables liver transplantation surgery without blood transfusion, suggesting that intravenous iron can be administered safely in this population (22,38-42). In this regard, our results may provide preclinical evidence for the adoption of preoperative iron therapy in patients with end-stage liver disease.
Limitations
There were several limitations in this study. First, because the aim of this study was to evaluate the safety of intravenous iron in subjects with LC, we used a rat model of cirrhosis and did not assess the presence of anemia in this model. Although we determined that the baseline hemoglobin level was below 130 g/L in cirrhosis-induced rats prior to treatment with Ferinject, this level was not sufficiently low to assess whether Ferinject can raise the level of hemoglobin in our model. Therefore, animal models with both LC and anemia should be used for more accurate tests. Because inflammatory state following LC is known to alter the iron related biomarkers, markers that is minimally affected by inflammation, such as the soluble transferrin receptor, can be used for test. Furthermore, combination treatment with vitamins, erythropoietin, or folic acid may achieve better efficacy. Second, we used only one Ferinject dose of 20 mg/kg, which is the standard dose recommended by the manufacturer. Thus, further experiments using various doses in a rat model of cirrhosis are needed to identify and confirm safe and effective dosing levels. Third, although we aimed to examine perioperative iron metabolism, this study did not include a surgical procedure. An appropriate animal experiment involving a surgical procedure is therefore warranted prior to clinical investigations.
In conclusion, in a rat model of CCl4-induced cirrhosis, the administration of intravenous iron did not result in significant changes in liver function test results or the degree of liver fibrosis. The treatment was beneficial in terms of reducing inflammation and oxidative stress. Although the administration of intravenous iron appears safe in our rat model of cirrhosis, further preclinical and clinical studies are warranted to confirm the safety and efficacy of intravenous iron in patients with LC or end-stage liver disease.
Acknowledgments
Funding: This study was supported by a grant provided by the Basic Science Research Program through the National Research Foundation of Korea (NRF), funded by the Ministry of Science and Information & Communication Technology (No. 2019R1F1A1063201).
Footnote
Reporting Checklist: The authors have completed the ARRIVE reporting checklist. Available at https://apm.amegroups.com/article/view/10.21037/apm-21-3039/rc
Data Sharing Statement: Available at https://apm.amegroups.com/article/view/10.21037/apm-21-3039/dss
Peer Review File: Available at https://apm.amegroups.com/article/view/10.21037/apm-21-3039/prf
Conflicts of Interest: All authors have completed the ICMJE uniform disclosure form (available at https://apm.amegroups.com/article/view/10.21037/apm-21-3039/coif). JHK and JSK report that the study was supported by a grant provided by the Basic Science Research Program through the National Research Foundation of Korea (NRF), funded by the Ministry of Science and Information & Communication Technology (No. 2019R1F1A1063201). The other authors have no conflicts of interest to declare.
Ethical Statement: The authors are accountable for all aspects of the work in ensuring that questions related to the accuracy or integrity of any part of the work are appropriately investigated and resolved. Experiments were performed under a project license (Certificate No. IACUC 18-KE-327) granted by institutional board of KNOTUS Co., Ltd., Incheon-si, Korea, in compliance with KOTUC Co., Ltd. laboratory institutional guidelines for the care and use of animals.
Open Access Statement: This is an Open Access article distributed in accordance with the Creative Commons Attribution-NonCommercial-NoDerivs 4.0 International License (CC BY-NC-ND 4.0), which permits the non-commercial replication and distribution of the article with the strict proviso that no changes or edits are made and the original work is properly cited (including links to both the formal publication through the relevant DOI and the license). See: https://creativecommons.org/licenses/by-nc-nd/4.0/.
References
- McHutchison JG, Manns MP, Longo DL. Definition and management of anemia in patients infected with hepatitis C virus. Liver Int 2006;26:389-98. [Crossref] [PubMed]
- Sheehy TW, Berman A. The anemia of cirrhosis. J Lab Clin Med 1960;56:72-82. [PubMed]
- Berman L, Axelrod AR. The blood and bone marrow in patients with cirrhosis of the liver. Blood 1949;4:511-33. [Crossref] [PubMed]
- Phillips DL, Keeffe EB. Hematologic manifestations of gastrointestinal disease. Hematol Oncol Clin North Am 1987;1:207-28. [Crossref] [PubMed]
- Kimber C, Deller DJ, Ibbotson RN, et al. The mechanism of anaemia in chronic liver disease. Q J Med 1965;34:33-64. [PubMed]
- Spahn DR, Schoenrath F, Spahn GH, et al. Effect of ultra-short-term treatment of patients with iron deficiency or anaemia undergoing cardiac surgery: a prospective randomised trial. Lancet 2019;393:2201-12. [Crossref] [PubMed]
- Kim YW, Bae JM, Park YK, et al. Effect of Intravenous Ferric Carboxymaltose on Hemoglobin Response Among Patients With Acute Isovolemic Anemia Following Gastrectomy: The FAIRY Randomized Clinical Trial. JAMA 2017;317:2097-104. [Crossref] [PubMed]
- Funk F, Ryle P, Canclini C, et al. The new generation of intravenous iron: chemistry, pharmacology, and toxicology of ferric carboxymaltose. Arzneimittelforschung 2010;60:345-53. [PubMed]
- Keating GM. Ferric carboxymaltose: a review of its use in iron deficiency. Drugs 2015;75:101-27. [Crossref] [PubMed]
- Toblli JE, Cao G, Rico L, et al. Cardiovascular, liver, and renal toxicity associated with an intravenous ferric carboxymaltose similar versus the originator compound. Drug Des Devel Ther 2017;11:3401-12. [Crossref] [PubMed]
- Intragumtornchai T, Rojnukkarin P, Swasdikul D, et al. The role of serum ferritin in the diagnosis of iron deficiency anaemia in patients with liver cirrhosis. J Intern Med 1998;243:233-41. [Crossref] [PubMed]
- Prieto J, Barry M, Sherlock S. Serum ferritin in patients with iron overload and with acute and chronic liver diseases. Gastroenterology 1975;68:525-33. [Crossref] [PubMed]
- Bloomer SA, Brown KE. Iron-Induced Liver Injury: A Critical Reappraisal. Int J Mol Sci 2019;20:2132. [Crossref] [PubMed]
- Brown KE, Dennery PA, Ridnour LA, et al. Effect of iron overload and dietary fat on indices of oxidative stress and hepatic fibrogenesis in rats. Liver Int 2003;23:232-42. [Crossref] [PubMed]
- OECD series on principles of good laboratory practice and compliance monitoring. Ann Ist Super Sanita 1997;33:1-172. [PubMed]
- Fortea JI, Fernández-Mena C, Puerto M, et al. Comparison of Two Protocols of Carbon Tetrachloride-Induced Cirrhosis in Rats - Improving Yield and Reproducibility. Sci Rep 2018;8:9163. [Crossref] [PubMed]
- Brunt EM. Grading and staging the histopathological lesions of chronic hepatitis: the Knodell histology activity index and beyond. Hepatology 2000;31:241-6. [Crossref] [PubMed]
- Hall AP, Davies W, Stamp K, et al. Comparison of computerized image analysis with traditional semiquantitative scoring of Perls' Prussian Blue stained hepatic iron deposition. Toxicol Pathol 2013;41:992-1000. [Crossref] [PubMed]
- Milic S, Mikolasevic I, Orlic L, et al. The Role of Iron and Iron Overload in Chronic Liver Disease. Med Sci Monit 2016;22:2144-51. [Crossref] [PubMed]
- Mehta KJ, Farnaud SJ, Sharp PA. Iron and liver fibrosis: Mechanistic and clinical aspects. World J Gastroenterol 2019;25:521-38. [Crossref] [PubMed]
- Auerbach M, Gafter-Gvili A, Macdougall IC. Intravenous iron: a framework for changing the management of iron deficiency. Lancet Haematol 2020;7:e342-50. [Crossref] [PubMed]
- Jabbour N, Gagandeep S, Mateo R, et al. Transfusion free surgery: single institution experience of 27 consecutive liver transplants in Jehovah's Witnesses. J Am Coll Surg 2005;201:412-7. [Crossref] [PubMed]
- Muñoz M, García-Erce JA, Remacha AF. Disorders of iron metabolism. Part 1: molecular basis of iron homoeostasis. J Clin Pathol 2011;64:281-6. [Crossref] [PubMed]
- Toblli JE, Cao G, Olivieri L, et al. Comparison of the renal, cardiovascular and hepatic toxicity data of original intravenous iron compounds. Nephrol Dial Transplant 2010;25:3631-40. [Crossref] [PubMed]
- Toblli JE, Cao G, Oliveri L, et al. Assessment of the extent of oxidative stress induced by intravenous ferumoxytol, ferric carboxymaltose, iron sucrose and iron dextran in a nonclinical model. Arzneimittelforschung 2011;61:399-410. [Crossref] [PubMed]
- Toblli JE, Cao G, Giani JF, et al. Nitrosative Stress and Apoptosis by Intravenous Ferumoxytol, Iron Isomaltoside 1000, Iron Dextran, Iron Sucrose, and Ferric Carboxymaltose in a Nonclinical Model. Drug Res (Stuttg) 2015;65:354-60. [PubMed]
- Urrutia PJ, Hirsch EC, González-Billault C, et al. Hepcidin attenuates amyloid beta-induced inflammatory and pro-oxidant responses in astrocytes and microglia. J Neurochem 2017;142:140-52. [Crossref] [PubMed]
- Vela D. The Dual Role of Hepcidin in Brain Iron Load and Inflammation. Front Neurosci 2018;12:740. [Crossref] [PubMed]
- Shander A, Muñoz M, Spahn DR. Intravenous iron for severe iron deficiency anaemia. Br J Anaesth 2018;121:342-4. [Crossref] [PubMed]
- Weiss G, Goodnough LT. Anemia of chronic disease. N Engl J Med 2005;352:1011-23. [Crossref] [PubMed]
- Goodnough LT, Nemeth E, Ganz T. Detection, evaluation, and management of iron-restricted erythropoiesis. Blood 2010;116:4754-61. [Crossref] [PubMed]
- Rössler J, Schoenrath F, Seifert B, et al. Iron deficiency is associated with higher mortality in patients undergoing cardiac surgery: a prospective study. Br J Anaesth 2020;124:25-34. [Crossref] [PubMed]
- Musallam KM, Tamim HM, Richards T, et al. Preoperative anaemia and postoperative outcomes in non-cardiac surgery: a retrospective cohort study. Lancet 2011;378:1396-407. [Crossref] [PubMed]
- Aydogan MS, Erdogan MA, Yucel A, et al. Effects of preoperative iron deficiency on transfusion requirements in liver transplantation recipients: a prospective observational study. Transplant Proc 2013;45:2277-82. [Crossref] [PubMed]
- Vamvakas EC. Transfusion-associated cancer recurrence and postoperative infection: meta-analysis of randomized, controlled clinical trials. Transfusion 1996;36:175-86. [Crossref] [PubMed]
- Fatourou EM, Tsochatzis EA. Transfusion strategies in patients with cirrhosis: less is more. Liver Int 2016;36:503-4. [Crossref] [PubMed]
- Tohme S, Varley PR, Landsittel DP, et al. Preoperative anemia and postoperative outcomes after hepatectomy. HPB (Oxford) 2016;18:255-61. [Crossref] [PubMed]
- Kalra PA, Bhandari S. Efficacy and safety of iron isomaltoside (Monofer(®)) in the management of patients with iron deficiency anemia. Int J Nephrol Renovasc Dis 2016;9:53-64. [Crossref] [PubMed]
- Jeffrey GP, McCall J, Gane E, et al. Liver transplantation in Jehovah's Witness patients in Australasia. Med J Aust 2007;187:188-9. [Crossref] [PubMed]
- Jeong JY, Jee HS, Koo BS, et al. Liver transplantation in Jehovah's Witnesses: two cases report. Korean J Anesthesiol 2017;70:350-5. [Crossref] [PubMed]
- Stoye A, Chapin JW, Botha J, et al. Bloodless liver transplantation in a Jehovah's Witness. Int Anesthesiol Clin 2011;49:108-15. [Crossref] [PubMed]
- Sheth M, Kulkarni S, Dhanireddy K, et al. Blood Conservation Strategies and Liver Transplantation Transfusion-Free Techniques Derived from Jehovah's Witness Surgical Cohorts. Mo Med 2015;112:389-92. [PubMed]