Evaluating the diagnostic value of using metagenomic next-generation sequencing on bronchoalveolar lavage fluid and tissue in infectious pathogens located in the peripheral lung field
Introduction
Lower respiratory tract infections (LRTI) is defined as radiological evidence of new or increasing pulmonary infiltrate(s) plus one or more of the following: fever, dyspnea, hemoptysis, hypothermia, wheezing, shortness of breath, physical findings, just like hypoxemia and rales (1). LRTI is the most common infectious disease. Because of potential pathogens like fungi, viruses and bacteria, LRTI can be hardly diagnosed (2-4). At present, among the pathogen detection methods of smear methods and microscope analysis of cultures are very common. However, these two methods are less sensitive and take longer time. Thanks to the continuously improved techniques for fast LRTI detection, the use of antimicrobial agents is also expanding. Bronchoalveolar lavage fluid (BALF) culture is close to the gold standard for histological diagnosis and has been recognized by clinics and laboratories because it meets the requirements for accurate diagnosis of LRTI. However, BALF culture is not conducted routinely in clinics because of the difficulties in collection of samples, possible cross-infection risk, tissue damage, and reluctant acceptance by patients. It is of great significance for respiratory patients and clinicians to optimize culture results of different specimens in the diagnosis of LRTI.
LRTI include acute tracheobronchial bronchitis, chronic bronchitis and pneumonia. Pneumonia is a very important component of LRTI. The infected area of the surrounding lung field is mainly concentrated in the third oval region, and consistent findings can be obtained from computed tomography (CT) scans (5). As for peri-pulmonary areas, the bronchus cannot be used for accurate diagnosis and observation (6). The other method that can be used to measure stability is transthoracic percutaneous biopsy (TTNA), but despite satisfactory surgery can be achieved, TTNA related complications can be fatal (7). Therefore, collection of pathogenic microorganisms at their exact site via bronchoscopy is particularly important.
The navigational bronchial lens has improved the specific diagnostic method for peripheral lesions. One of the two approaches that can be used today is electromagnetic navigation (8). It directs a guide sheath and biopsy instrument to peripheral lesions using electromagnetic position-sensor information (9). Alternatively, virtual bronchoscopic navigation (VBN) as the other method of navigational bronchial lens can reconstruct the image of the cavity by computer simulation navigation technology to observe the surface of the cavity. For VBN, the internal cavity image can be constructed by means of computer simulation and used for the observation of the inner surface. Finally, a dynamic image is generated by playback of the VBN, and it was similar to the endoscope which can be moved forward or backward to form a direct vision (10). Refer to the analysis results of the research we conducted, endobronchial ultrasound is suggested to show prognostic significance in treating tiny peripheral lesions.
New metagenome sequencing technology (mNGS) is an advanced sequencing method at present. At the same time, it has been has been widely used in the analysis of microbial community and high-throughput sequencing in clinical sample detection. The types of nucleic acids in the samples can be identified by bioinformatic analysis and quantitative data such as sequence number and coverage of the pathogens. The greatest advantages of mNGS are its high sensitivity, low costs and high throughput. Strikingly, mNGS can simultaneously detect millions or even hundreds of millions of DNA or RNA sequences, which greatly accelerates the speed of whole-genome sequencing and greatly reduces the cost of single-base sequencing. Consequently, mNGS has completely changed the previous research model, which brings new changes to macrogenomics, genomics and transcriptome, and mNGS are also constantly penetrating into the field of microbiology. At present, relevant research results show that mNGS have a very significant effect on the rapid diagnosis of complex infections (11). Furthermore, mNGS has demonstrated its ability to identify microbial pathogens when traditional diagnostics have failed (12).
mNGS, the openly accessible diagnosis system, has been used to diagnose over 8,000 bacterial species, like parasites, viruses, bacteria, and fungi. Thereby providing an effective technique for the diagnosis of incurable and rare pathogenic microbial infections. Ai et al. used mNGS to diagnose cross-species transmission of the pig herpes virus, and thus provide targeted treatment for the patient (13), and Wang et al. confirmed a rare amoebic encephalitis with mNGS (14).
In the previous research, the value of VBN combined with BALF in the diagnosis of peripheral pulmonary nodules were evaluated. However, few studies have evaluated the value of VBN and BALF in the diagnosis of peripheral pulmonary infection. In this paper, the main content of the study is the diagnostic method combining VBN and mNGS as a potential diagnostic tool for patients with lower respiratory tract infection in hospital. Our focus was to evaluate the application of mNGS combined with VBN for infections in the peripheral lung field (PLF). We present the following article in accordance with the STARD reporting checklist (available at https://apm.amegroups.com/article/view/10.21037/apm-21-3474/rc).
Methods
Patients
The research work in this paper belongs to the category of single-center retrospective study and has been approved and recognized by the relevant administrative institutions. At the same time, all the personnel involved in this study have signed the relevant informed letters and consent forms.
Selection requirements (see Figure 1): (I) immunodeficient cases; (II) possible opportunistic pathogen infection; (III) over 14 years of age; (IV) lesions suspected to be infected; and (V) lesions differentiated from other diseases. The exclusion criteria were: (I) bronchial lesion consistent with hemorrhage, distortion, and deformation reaction found on bronchoscopy; (II) suspected noninfectious lesion or noninfectious lesion confirmed by pathological examination; (III) presence of comorbidities just like malignant arrhythmia, serious hypertension, serious asthma, severe myocardial infarction (MI), and unstable angina pectoris over the last 3 months; (IV) pregnant women or those who cannot proceed in the absence of antiplatelet or anticoagulant medication; (V) lungs with multiple bullae at risk of rupturing; and (VI) severe wasting or other reasons for inability to participate in the study; (VII) missing data on the index test and indeterminate index test.
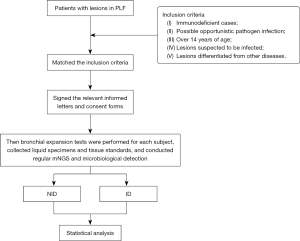
Patients infected with PLF between July 2018 and February 2018 were analyzed and divided into two analysis groups [non-infectious disease (NID) group and infectious disease (ID) group]. Then bronchial expansion tests were performed for each subject, collected liquid specimens and tissue standards, and conducted regular mNGS and microbiological detection and analysis. No difference in age or sex was statistically significant between two groups. The study was conducted in accordance with the Declaration of Helsinki (as revised in 2013). The study was approved by the Ethics Board of The First Hospital of Qinhuangdao (No. 2022A071) and informed consent was taken from all the patients. Informed consents of patients under the age of 18 have been obtained from their parents/guardians.
Preparation of direct path electronic navigation
Procedure
Each included case was examined by the thin video bronchoscope (BF-P-260F; outer diameter, 4.0 mm; Olympus Medical Systems, Japan). Virtual images were controlled by the physician assistant in the process of bronchoscopy, which were used to instruct the insertion of endoscope by the bronchoscopist.
Bronchoscopy was assisted by the VBN system (DirectPathV1.02, Cybernet Sems, China). After reaching the target, tissue samples were collected using biopsy forceps (JHYFB-18-105-O-A1, Changzhou Jiuhong Medical Devices Co. Ltd.) via the working channel of the endoscope. Biopsy specimens were fixed with formalin and histological examination was performed. Altogether 87 cases enrolled in this work underwent BALF. BALF and lung biopsies were delivered within two hours to the histopathology laboratory and the microbiology laboratory and used to identify the mycobacterium tuberculosis complex (MTBC), fungi and bacteria. KOH test, Gram staining and Ziehl-Neelsen staining were performed using smear microscopy. At the same time, two pathologists independently issue the results of the pathological examination report. The histopathological laboratory processed the clinical samples using standard methods. The remaining tissues and BALF samples need to be matched one-to-one with patient-related records and stored at −70 °C.
Metagenomic next-generation sequencing (mNGS) and bioinformatics
During the analysis and extraction of respiratory DNA samples, the main use of Tianan microDNA kit to extract. At the same time, the DNA fragments extracted by ultrasound were segmented to obtain fragments of 200–500 bp. Agilent 2100 Bioanalyzer (USA) mainly functioned to regulate DNA fragment quality and size. Circular single-stranded DNA structure was formed by cyclization. The prepared DNA Nanoballs (DNB) nanospheres were loaded into the sequencing chip and were sequenced by BGISEQ-500. Three days were typically required to complete the mNGS analysis and to issue the reports (15).
Preprocessing and reference databases
Low-quality data and data for lengths of less than 35 bp were removed to obtain high-quality data. Relative to BWA (Burrow-Wheeler Aligne) (BWA: http://bio-bwa.sourceforge.net/), the high-quality data were obtained by removing the sequencing aligned to the human genome. The read data with lower complexity was removed, and the large microbiological database was compared with the remaining data.
Sanger sequencing was performed to validate the sequencing results.
To assess microbial contaminants in our reagents and laboratory environment, we sequenced 10 control samples from healthy donors.
Diagnostic criteria
Case definitions of LRTI were derived from the US CDC/NHSN Surveillance definition of pneumonia. Microbiological criteria are used in conjunction with diagnostic criteria. Clinical criteria needed to have at least one fulfillment among items 1–3, and at least two fulfillments among items 4–6. The clinical criteria were: (I) >2 serial chest imaging results in new, progressive or persistent infiltration, consolidation, or cavitation; (II) WBC data increased and exceeded 12,000 mm3; (III) fever (the temperature was more than 38 °C); (IV) adults over 70 years of age, altered mental status with no other recognized cause; (V) new sputum changes or purulent, sputum aspiration or secretions need to be improved; (VI) problems just like dyspnea worsen, the shortness of cough, breath or and ventilation needs increase.
We adopted the following biological criteria: (I) positive lower respiratory or pleural fluid culture; (II) positive results after separate blood culture; (III) positive antibody or antigen testing; (IV) positive results of viral PCR analysis.
Criteria for the mNGS result
All organisms involved in the identification were classified according to their coverage rates in a particular sample group and an interpretation of the strictly mapped reads. In order to carry out further research and analysis, 10 pathogenic bacterial species were acquired, and these pathogenic reads ranked in the top 10. First, according to results obtained by Langelier and coworkers (16), viral mNGS and bacteria (except mycobacteria) are 5 times more effective than other microorganisms in identifying microorganisms. Also, ensure that the number of strict mapping reads exceeds 3. Secondly, the detection of fungi requires more than three readings in strict accordance with the mapping requirements, and exceeds the upper limit of the target reference range (17). Thirdly, for mycobacterium, factors such as low DNA contamination and complex and difficult DNA extraction process should be taken into consideration. If the number exceeds 1, the mycobacterium (MTB) could be determined as positive (18).
Statistical analysis
Use percentages, medians, and frequencies to represent data. When discrete variables were processed, Pearson χ2 and Fisher’s exact test could be used to perform categorical variables analysis. Continuous variables were analyzed and compared by Mann-Whitney U test. A P value lower than 0.05 stood for the statistically significant difference. IBM SPSS Statistics (version 22) was used for statistical analyses.
Results
Sample and patient characteristics
Difference in age (F=0.905, P=0.117) or sex (χ2=1.219, P=0.269) was not significant. mNG and conventional tissue culture were adopted for each standard. Group ID 61/87 (70.1%) identified in combination with mycobacterium complex 6/61 (9.8%), bacteria 17/61 (27.9%) and fungi 24/61 (39.3%) and virus 9/61 (14.8%). Five of the target subjects were infected at the same time, accounting for 8.2% among the overall cases (Figure 2A). Pathology and culture techniques were used to diagnose the primordia in all patients.
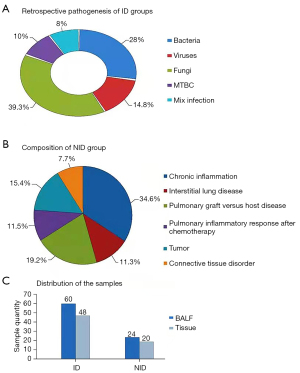
Interstitial pulmonary disease was found in the lower part of the sample (3, 11.5%), connective tissue disease (2, 7.7%) NID group (26/87, 29.9%) as well as chronic inflammation (9, 34.6%), besides the lung graft versus host disease caused by chemotherapy of (5, 19.2%), lung inflammation (3, 11.5%) and tumor (4, 15.4%) (Figure 2B).
There were 152 cases undergoing mNGS, while the remaining patients from BALF and the organization were 84 and 68, respectively (Figure 2C). By mNGS diagnosis, Acinetobacter baumannii, Pseudomonas aeruginosa and Rothia mucilaginosa were the bacterial species with the highest abundance; according to mNGS diagnosis, fungal species Aspergillus fumigatus, Pneumocystis jeri, and Cryptococcus neoformans were the fungal species showed the greatest levels. The top three virus types were human breast adenovirus B, human γ-herpesvirus 4 and human β-herpesvirus 5, and the diagnosis is realized by mNGS. The read numbers were 3-17800.
Comparison of diagnostic values of mNGS and culture
Table 1 presents the related mNGS positive rates, culture experiments in the ID group and the NID group. For the ID group and the NID group, the probability of positive prediction for mNGS test was 90.6% and 64.5%, respectively. Therefore, the corresponding negative and positive likelihood ratios (NLR and PLR) were 0.233 and 4.07, respectively. Meanwhile, the negative and positive prediction rates of tissue mNGS were 56.7% and 92.1%, separately. The higher sensitivity of BALF mNGS and tissue mNGS compared to cultures was consistent with the expected performance (81.4% vs. 32.8%; χ2=43.83, P=0.000; 72.9% and 32.8%; Chi-square =25.384, P=0.000). However, the different specificities were not of statistical significance between normal culture and mNGS (96.2% vs. 80.0%; P=0.093; 96.2% and 85.0%; P=0.092).
Table 1
Group | ID | NID | Sensitivity (%) | Specificity (%) | |||
---|---|---|---|---|---|---|---|
Positive | Negative | Positive | Negative | ||||
BALF mNGS | 48 | 11 | 5 | 20 | 81.4* | 80.0§ | |
Tissue mNGS | 35 | 13 | 3 | 17 | 72.9* | 85.0§ | |
Culture | 20 | 41 | 1 | 25 | 32.8 | 96.2 |
*, BALF and tissue mNGS increased the sensitivity rate in comparison with culture (81.4% vs. 32.8%; χ2=43.83, P=0.000; 72.9% vs. 32.8%; χ2=25.384, P=0.000); §, the specificity difference of mNGS (BALF and tissue) and culture was not significant (80.0% vs. 96.2%; P=0.093; 85.0% vs. 96.2%; P=0.303). mNGS, metagenomic next-generation sequencing; BALF, bronchoalveolar lavage fluid; ID, infectious diseases; NID, noninfectious diseases.
Fungal detection by metagenomic sequencing
To evaluate the diagnostic performance between BALF and tissue mNGS for fungi detection, this study screened 87 affected cases and classified them as two groups, namely, fungal and non-fungal groups. The results of tissue mNGS and BALF mNGS for diagnosis of patients are shown in Figure 3A below. According to Figure 3B, tissue mNGS-derived negative predictive value and central gold prediction value in diagnosing disease were 86% and 83.8%, separately, and the corresponding PLR and NLR were 10.492 and 0.34, separately. On this basis, the two detection methods were basically consistent in terms of detection sensitivity. The software analysis results were (76.9% vs. 68.2%; χ2=0.461, P=0.532). Difference in detection specificity was not significant between those 2 detection approaches; 91.5% vs. 93.5% were obtained upon consistency analysis (χ2=0.161, P=0.688).
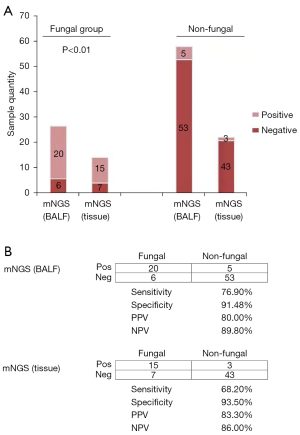
Bacterial detection by metagenomic sequencing
Of all the patients who participated in the diagnosis, in the bacterial group, 19 samples were positive, and 1 was negative using BALF mNGS; 10 samples were positive and 6 were negative using tissue mNGS. In the non-bacterial group, 12 samples were positive, and 52 were negative by BALF mNGS; 4 samples were positive, and 48 were negative using tissue mNGS. Meanwhile, the 98.1% negative and 61.3% positive prediction rates were achieved by BALF. Additionally, BALF mNGS attained the PLR as high as 5.08, and NLR of 0.06 (Figure 4). Moreover, the tissue mNGS achieved the negative and positive prediction rates at 88.9% and 71.4%, separately, with the corresponding PLR of 8.12 and NLR of 0.406. The comparison of the two different methods shows that there is a significant difference in detection sensitivity; 95.0% vs. 62.5% were obtained upon statistical analysis (P=0.03). Difference in specificity was not significant between both detection approaches, according to statistical analyses (81.3% vs. 92.3%, χ2=2.95, P=0.086).
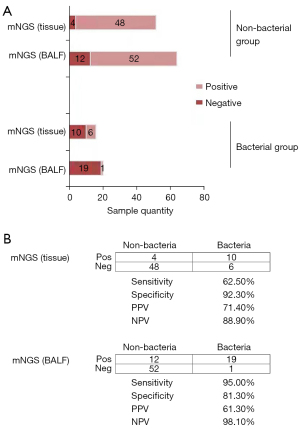
Correlation and concordance for fungi and bacteria between BALF mNGS and tissue mNGS
Figure 5A shows the fourteen fungi identified in BALF and tissue using mNGS. One fungi were identified only by BALF mNGS, two fungi were identified only by tissue mNGS. A consistency test also showed that these two methods had some degree of consistency (k=0.673, P=0.001). As Figure 5B shown, in bacterial-infection samples, nine bacteria were recognized using both BALF and tissue mNGS, two bacteria were recognized using BALF mNGS, and one bacteria were recognized using tissue mNGS only. When applying Cohen’s Kappa statistics for a measure of agreement, only a slight agreement (k=0.329, P=0.005) was found between the two techniques.
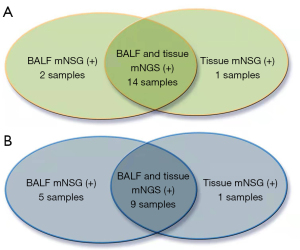
Discussion
This is the first study to analyze the value of mNGS and VBN in the diagnosis of LRTI. At present, tissue culture remains the preferred choice to examine the LRTI etiology. However, the sensitivity of this method is low for patients with immunosuppression or underlying diseases, as well as in patients with fastidious organisms or viral co-infection (19). The sensitivity of this method is also limited by the use of antibiotics and antifungals. Tissue culture only detects viable microorganisms, and the medium used is not optimized for culturing fungi and fastidious bacteria. This work enrolled altogether 87 patients. We found that mNGS added diagnostic value by its timely detection of clinically relevant microbes that were missed by culture. mNGS can be directly sequenced to extract sample DNA, with little deviation. This is also one of the pathogen detection methods MNGS has a unique advantage.
A previous study was the first to demonstrate that mNGS can be used to detect pathogens in cerebrospinal fluid (20), and Xie et al. reported a retrospective study on the application of mNGS in pathogen detection of severe pneumonia (21). As found by Xie’s study, viruses (15.4%), Acinetobacter baumannii (12.3%), fungi (11.8%), pseudomonas aeruginosa (10.1%), and Staphylococcus aureus (5.6%) were the most abundant species in MTBC, bacteria 17/61 (27.9%) and fungi 24/61 (39.3%) and viral 9/61 (14.8%), including 5 (8.2%) cases with mixed infections. Consistent with results from Xie and colleagues, the top three bacteria found in our study using mNGS were P. aeruginosa, A. baumannii and R. mucilaginosa, which are most commonly found in LRTIs.
The focus of the present study was the etiological diagnosis of patients with LRTI using mNGS in two different types of samples, and we found that mNGS was advantageous from several aspects.
In comparison with common tissue culture (32.8%), BALF mGNS achieved the pathogen detection sensitivity 81.4% (P<0.01). Meanwhile, BALF mNGS attained the pathogen detection sensitivity of 72.9 relative to 32.8% attained by routine tissue culture. mNGS achieved comparable diagnosis sensitivity (81.4%) and specificity (80.0%) with those (100% and 76.5%, respectively) found in a similar study by Li et al. (22). Specifically, mNGS obtains nucleic-acid-sequence information of the pathogen directly from the sample and comparatively analyzes the resulting sequences through bioinformatic methods, which are independent of the culture results. Relying on unbiased and random methods, mNGS can detect the genomes of both known pathogens and unknown microorganisms, which makes mNGS a powerful approach for identifying patients infected with new pathogens.
Second, mNGS is known for its superior feasibility and stability in detecting fungi. Fungi and fungal spores can exist in digestive tract, human lungs, the mouth and skin (23). Fungi have a thick cell wall that differs from that of bacteria, so bacterial DNA extraction methods are not necessarily applicable to fungi. Fungi require unique methods for different DNA testing methods, PCR amplification and DNA extraction can produce heterogeneous findings of fungi. As far as we know, some fungi and new species are difficult or even impossible to identify by conventional culture (24). NGS technology is better than conventional culture in terms of both the breadth and depth of detection, as well as in the analysis of common cell structures. Differences in specificity and statistical value were not significant between BALF mNGS and tissue mNGS. The result is (68.2% vs. 76.9%, χ2=0.461, P=0.532), (93.5% vs. 91.5%, χ2=0.161, P=0.688). The consistency test also showed that these two methods had some degree of consistency (k=0.673, P=0.000) which demonstrates the reliability of BALF mNGS and tissue mNGS in the diagnosis of fungal infection.
Third, in diagnosing bacteria, applying mNGS contributes to demonstrating the sensitivity and specificity. In the present work, tissue mNGS and BALF mNGS attained the sensitivity of 62.5% and 95% in diagnosis; while their specificity values were 92.3% and 81.3%, separately. There are many differences in the tests of specificity and sensitivity of mNGs in different literatures, Miao et al. (25) reported a retrospective analysis of a large sample of 511 patients that were divided into an ID group, NID group, and unknown-cause group. By integrating prior research results, BALF mGNS achieved the negative and positive prediction rates of 37.4% and 91.2% in detecting IDS, separately; as for tissue mNGS its sensitivity was 50.7% and its specificity was 85.7% in detection. Li et al. (22) came to similar results. The study in this paper found that the sensitivity of mNGS was relatively similar to the research results published by Miao (62.5% vs. 50.7%). Additionally, the analysis results obtained by ourselves found that the performance of BALF mNGS in terms of detection sensitivity was consistent with the results reported by Li et al. In terms of sensitivity, BALF mNGS had a higher advantage than tissue mNGS (95.0% vs. 62.5%, P=0.03). However, there is no significant difference in the detection specificity between both approaches.
Finally, the rapid feedback of mNGS may hasten clinical decision making and guide clinical laboratories to improving culture conditions for fastidious organisms. Xie et al. (21) found that mNGS technology can determine the pathogens in severe pneumonia patients at an early stage and guide the application of antibacterial drugs, and it decreased the mortality rate. In this study, tissue mNGS and BALF mNGs combined with VBN guided bronchoscopy can achieve accurate pulmonary infection. The diagnosis of peripheral small lung lesions was optimized for VBN detection (26). In other words, using virtual reality image to introduce bronchoscope, this method has the safety of operation. In addition, considering that the VBN does not need to introduce any other equipment, it has a cost advantage. VBN is a VB method clinically applicable to arrive at the peripheral lesions. The lesion bronchial path virtual images can be generated and adopted to navigate when the bronchoscope is advanced. Further clinical trials may be needed to support the idea that VBN assisted bronchoscopy can improve diagnostic value preserve tissue samples and accurately reach lesion sites.
There are several limitations in the present work, like its sample size. Future studies must focus on identifying pathogens, and detection methods should be optimized in intensive care and hospital wards. There are also differences in diagnostic criteria for the report itself. Therefore, the criteria for corresponding diagnosis of pathogens in respect of MGNS are not very clear. It is necessary to enforce strict standards on this process, as correct optimization will foster accurate detection. When mNGS detects low reads, it is a challenge to make an accurate clinical diagnosis. The results may represent true positive results with a low detection rate, but may also represent false positives, and these possibly result from cross-contamination, sequencing errors, or data analysis errors (highly similar or less complex areas) (27). In the present study, we sequenced 10 negative control samples from healthy donors to identify microbial contaminants in our reagents and laboratory environment. The sensitivity of detection of intracellular infected bacteria such as MTB was very low, therefore, when we think that the strict mapping reads more than 1, then the result is positive. According to detection findings and clinical manifestations, we can make a final diagnosis.
The advantages of the clinical application of NGS technology are mainly in terms of its low cost short-sequence sequencing and high throughput. Through short-sequence sequencing, microbial species can be identified quickly and accurately. Although mNGS has broad application prospects, it also has limitations and shortcomings, like sequencing depth lacking, inability to achieve adequate coverage, and increased likelihood of misdiagnosis when the length of the pathogenic gene is short and/or there is a large variety of genes (28). Due to the defects in quality control methods and the inconsistency of verification standards, mNGS is currently used as a supplement to traditional detection methods (29).
As revealed by our results, both BALF and mNGS can be used for the analysis and detection of pulmonary peripheral pathogens. When tissue culture is combined with mNGs, it can achieve high sensitivity in detection, in particular for infections at PLF sites. In the future, the shortcomings of mNGS for detection of pathogens will be greatly reduced, it can promote clinical popularization and application to a large extent. Therefore, mNGS may be an important detection technology for accurate diagnosis and targeted therapies of clinical IDs. However, it should be noted that mNGS needs to be interpreted and managed precisely. The results of mNGS analysis were compared by clinical observation and routine experimental diagnosis.
Conclusions
Our results indicate that VBN auxiliary bronchoscopy plus mNGS and BALF can be applied in detecting lung pathogens. Meanwhile, mNGS combined with tissue culture is blind, which may achieve high sensitivity in detection, in particular for infections at PLF sites.
Acknowledgments
We thank the physicians who provided clinical support, and patients and their families.
Funding: None.
Footnote
Reporting Checklist: The authors have completed the STARD reporting checklist. Available at https://apm.amegroups.com/article/view/10.21037/apm-21-3474/rc
Data Sharing Statement: Available at https://apm.amegroups.com/article/view/10.21037/apm-21-3474/dss
Conflicts of Interest: All authors have completed the ICMJE uniform disclosure form (available at https://apm.amegroups.com/article/view/10.21037/apm-21-3474/coif). The authors have no conflicts of interest to declare.
Ethical Statement: The authors are accountable for all aspects of the work in ensuring that questions related to the accuracy or integrity of any part of the work are appropriately investigated and resolved. The study was conducted in accordance with the Declaration of Helsinki (as revised in 2013). The study was approved by Ethics Board of The First Hospital of Qinhuangdao (No. 2022A071) and informed consent was taken from all the patients. Informed consents of patients under the age of 18 have been obtained from their parents/guardians. Anyone involved in the study has been hospitalized.
Open Access Statement: This is an Open Access article distributed in accordance with the Creative Commons Attribution-NonCommercial-NoDerivs 4.0 International License (CC BY-NC-ND 4.0), which permits the non-commercial replication and distribution of the article with the strict proviso that no changes or edits are made and the original work is properly cited (including links to both the formal publication through the relevant DOI and the license). See: https://creativecommons.org/licenses/by-nc-nd/4.0/.
References
- World Health Organization (2017). The top10 causes of death. Available online: www.who.int/en/news-room/fact-sheets/detail/the-top-10-causes-of-death
- Renaud C, Campbell AP. Changing epidemiology of respiratory viral infections in hematopoietic cell transplant recipients and solid organ transplant recipients. Curr Opin Infect Dis 2011;24:333-43. [Crossref] [PubMed]
- Ruppé E, Baud D, Schicklin S, et al. Clinical metagenomics for the management of hospital and healthcare-acquired pneumonia. Future Microbiol 2016;11:427-39. [Crossref] [PubMed]
- De La Cruz O, Silveira FP. Respiratory fungal infections in solid organ and hematopoietic stem cell transplantation. Clin Chest Med 2017;38:727-39. [Crossref] [PubMed]
- Baaklini WA, Reinoso MA, Gorin AB, et al. Diagnostic yield offiberoptic bronchoscopy in evaluating solitary pulmonary nodules. Chest 2000;117:1049-54. [Crossref] [PubMed]
- Asano F, Ishida T, Shinagawa N, Sukoh N, et al. Virtual bronchoscopic navigation without X-ray fluoroscopy to diagnose peripheral pulmonary lesions: a randomized trial. BMC Pulm Med 2017;17:184. [Crossref] [PubMed]
- Choi JW, Park CM, Goo JM, et al. C-arm cone-beam CT-guided percutaneous transthoracic needle biopsy of small (</= 20 mm) lung nodules: diagnostic accuracy and complications in 161 patients. AJR Am J Roentgenol 2012;199:W322-30. [Crossref] [PubMed]
- Bessich JL, Sterman DH. Improving electromagnetic navigation: One nodule at a time. Respirology 2020;25:130-1. [Crossref] [PubMed]
- Guo C, Diao X, Huang C, et al. Application and Progress of Electromagnetic Navigation Bronchoscopy in Department of Thoracic Surgery. Zhongguo Fei Ai Za Zhi 2022;25:118-23. [PubMed]
- Asano F. Advanced bronchoscopy for the diagnosis of peripheral pulmonary lesions. Respir Investig 2016;54:224-9. [Crossref] [PubMed]
- Wilson MR, Shanbhag NM, Reid MJ, et al. Diagnosing Balamuthia mandrillaris encephalitis with metagenomic deep sequencing. Ann Neurol 2015;78:722-30. [Crossref] [PubMed]
- Brown JR, Morfopoulou S, Hubb J, et al. Astrovirus VA1/HMO-C: an increasingly recognized neurotropic pathogen in immunocompromised patients. Clin Infect Dis 2015;60:881-8. [Crossref] [PubMed]
- Ai JW, Weng SS, Cheng Q, et al. Human endophthalmitis caused by pseudorabies virus infection, China, 2017. Emerg Infect Dis 2018;24:1087-90. [Crossref] [PubMed]
- Wang Q, Li J, Ji J, et al. A case of Naegleria fowleri related primary amoebic meningoencephalitis in China diagnosed by next-generation sequencing. BMC Infect Dis 2018;18:349. [Crossref] [PubMed]
- Wang X, Ruan Q, Xu B, et al. Human African Trypanosomiasis in Emigrant Returning to China from Gabon, 2017. Emerg Infect Dis 2018;24:400-4. [Crossref] [PubMed]
- Langelier C, Zinter MS, Kalantar K, et al. Metagenomic sequencing detects respiratory pathogens in hematopoietic cellular transplant patients. Am J Respir Crit Care Med 2018;197:524-8. [Crossref] [PubMed]
- Long Y, Zhang YX, Gao YP, et al. Diagnosis of Sepsis with Cell-free DNA by Next-Generation Sequencing Technology in ICU Patients. Arch Med Res 2016;47:365-71. [Crossref] [PubMed]
- Simner PJ, Miller S, Carroll KC. Understanding the promises and hurdles of metagenomic next-generation sequencing as a diagnostic tool for infectious diseases. Clin Infect Dis 2018;66:778-88. [Crossref] [PubMed]
- Van de Beek D, Cabellos C, Dzupova O, et al. ESCMID guideline: diagnosis and treatment of acute bacterial meningitis. Clin Infect Dis 2018;66:778-88.
- Yao M, Zhou J, Zhu Y, et al. Detection of Listeria monocytogenes in CSF from Three Patients with Meningoencephalitis by Next-Generation Sequencing. J Clin Neurol 2016;12:446-51. [Crossref] [PubMed]
- Xie Y, Du J, Jin W, et al. Next generation sequencing for diagnosis of severe pneumonia: China, 2010-2018. J Infect 2019;78:158-69. [Crossref] [PubMed]
- Li H, Gao H, Meng H, et al. Detection of pulmonary infectious pathogens from lung biopsy tissues by metagenomic next-generation Sequencing. Front Cell Infect Microbiol 2018;8:205. [Crossref] [PubMed]
- Gow NA, Netea MG. Medical mycology and fungal immunology: new research perspectives addressing a major world health challenge. Philos Trans R Soc Lond B Biol Sci 2016;371:20150462. [Crossref] [PubMed]
- Teragawa H, Fukuda Y, Matsuda K, et al. Myocardial bridging increases the risk of coronary spasm. Clin Cardiol 2003;26:377-83. [Crossref] [PubMed]
- Miao Q, Ma Y, Wang Q, et al. Microbiological diagnostic performance of metagenomic next generation sequencing when applied to clinical practice. Clin Infect Dis 2018;67:S231-S240. [Crossref] [PubMed]
- Ishida T, Asano F, Yamazaki K, et al. Virtual bronchoscopic navigation combined with endobronchial ultrasound to diagnose small peripheral pulmonary lesions: a randomised trial. Thorax 2011;66:1072-7. [Crossref] [PubMed]
- Yu XJ, Liang MF, Zhang SY. N Engl J Med 2011;364:1523-32. [Crossref] [PubMed]
- Schlaberg R, Chiu CY, Miller S, et al. Validation of metagenomic next-generation sequencing tests for universal pathogen detection. Arch Pathol Lab Med 2017;141:776-86. [Crossref] [PubMed]
- Gargis AS, Kalman L, Lubin IM. Assuring the quality of next generation sequencing in clinical and public health microbiology. J Clin Microbiol 2016;54:2857-65. [Crossref] [PubMed]