Exploring the complex pathways between somatosensory impairment, physical ability and affective symptoms following stroke: mediation analyses
Introduction
Stroke is the third most common cause of disability of disability-adjusted life-years (DALYs) from all-cause after ischemic heart disease, according to the Global Burden of Disease (GBD) 2013 stroke burden report (1-3). Anxiety and depression are the most common issues in post-stroke patients (4-6), and the prevalence of post-stroke depression (PSD) is 31.1%, and post-stroke anxiety (PSA) 20.4% in Germany (7). Furthermore, these features frequently lead to worse activities of daily living (ADL) or quality of life. Li et al. found anxiety and depression scores were meaningful predictors for ADL and quality of life (8). Therefore, improving anxiety or depression is critical to improving overall health and quality of life in patients after stroke. In this context, affective symptoms are defined as one’s symptoms and disorders of mood (e.g., anxiety, depression).
It has been reported that most patients after stroke suffer from disabilities, such as motor impairment, muscles weakness, and balance disorder (9). Physical disability resulting from the stroke such as poor physical prowess and quality of life can directly affect post-stroke mood disorders (10). A recent study found that limb-extremity motor impairments were also associated with depressive symptoms (11). Boudokhane et al. demonstrated that the severity of physical function was a significant factor in predicting health-related quality of life in patients with stroke (12), and in another study, strong correlations were established among depression, balance, and physical performance (13). Similarly, impaired movement may result in poor quality of life, which in turn caused stress, anxiety, vulnerability, and frustration to patients and families. Thus, rehabilitation professionals especially pay attention to muscle strength, the flexibility of a joint, and patients’ daily life functioning. Yuan et al., for example, found that improving muscle strength in patients with stroke can be beneficial for the management of patients with mental health disorders (14). Therefore, in the study, we assume that physical activity is negatively associated with affective symptoms.
Nevertheless, in terms of somatic sensation, studies focusing on the correlation between somatosensory impairment and depression or anxiety are few concerning patients with stroke. Impaired sensory function is indirectly associated with mood disorders. For example, neuropathic pain syndromes have the characteristics of unique sensory symptoms, such as hyperalgesia, burning pain, and dysesthesias, which frequently occur in combination with psychiatric disorders (15). Thus, we propose that somatic sensation may negatively influence affective symptoms.
Summing up the above discussion, the first purpose of this study is to elucidate the mechanism through which physical ability and somatosensory impairment influence affective symptoms in patients with stroke.
According to the theory of postural control, it is proposed by Massion in 1992, holding that maintaining equilibrium is base for the postural support for the movement. It also highlights that during a given motor act, the central nervous system has to not only coordinate posture and movement but also coordinate posture and equilibrium (16). The postural control theory has been developed extensively in recent decades. Likewise, core stability is derived from this theory. It is widely accepted that core stability is important for improving athletic function. In 2006, Kibler et al. suggests that core stability is pivotal for rehabilitation because it is the base for extremity function (17). It may be concluded that balance is a critical and complex skill integrating many physiological system such as mobility, postural stability, autonomic nervous system (18). Consequently, we assume that balance impairment can mediate the relationship between physical ability and affective symptoms and the relationship between somatosensory impairment and affective symptoms.
At first, we theorize this relationship by drawing on the theory of postural control. The theory discusses that trunk stability is one of the essential components of balance, which plays a vital role in improving sitting and standing balance, gait, and ADL for all people including children, older persons, and post-stroke subjects (19-21). Clinically, the prevalence of balance impairment was 48.1% post-stroke (22). Balance is crucial to maintaining functional activities during sitting and standing. Postural control and sitting balance have been reported to be significant predictors of discharge after stroke (23-28), and balance disorders indicate links between falls and mood disorders (29). For example, poor trunk stability leads to balance disorders as a result of falls, and fall events induce anxiety or depression in patients. Thus, recurrent falls could be directly linked with anxiety or depression (21) and even cause adverse effects on ADL or quality of life (30). Furthermore, Alghwiri et al. concluded that better balance ability and physical performance were associated with lower depression scores (13), while French et al. found depression symptoms moderate between the relationship physical capability and balance self-efficacy (31). Despite these studies have not directly indicated that balance function mediates the relationship between physical ability and affective symptoms, they do suggest an association between them. Consequently, the second purpose of this study is to elucidate the balance function is a mediator through which physical ability influences affective symptoms in patients with stroke. What’s more, one review illustrates that postural stability is associated with autonomic regulation, because they may share neural substrates (18). Considering the autonomic nervous system participating in postural control, we assume that somatic sensation may influence affective symptoms through balance function for patients with stroke.
In addition to the theory of postural control, we especially focus on cognitive ability. This is a fact that cognitive capability has played an important role in the ability to live independently after a stroke (32). A cross-sectional study of older women showed that those who had been inactive physically across the life course may have poorer cognitive performance. This finding also indicated early-life physical activity may be associated with a lower risk of cognitive impairment in old age (33), which in turn may suggest that physical disability may influence the decrease of cognitive capability. Besides, cognitive impairment has been found to be related to poststroke depression (34). Thus, we propose that physical ability may also affect mood through cognitive capability for stroke patients.
In summary, the aim of this study is threefold. Firstly, we explore the relationship between physical ability, somatosensory impairment and affective symptoms. Secondly, we explore the mediating effect of the balance impairment on the relationship between physical ability and affective symptoms, and the relationship between somatosensory impairment and affective symptoms. Thirdly, we explore the mediating effect of cognitive capability on the relationship between physical ability and affective symptoms. Therefore, we hypothesized that (I) physical ability was negatively associated with affective symptoms (H1c), and somatosensory impairment was positively associated with balance impairment (H2c); (II) balance impairment could mediate the relationship between physical ability and affective symptoms (H1a, H1b) and the relationship between somatosensory impairment and affective symptoms (H2a, H1b); (III) cognitive capacity may mediate the relationship between physical ability and affective symptoms (H3a, H3b) (Figure 1). We present the following article in accordance with the STROBE reporting checklist (available at https://apm.amegroups.com/article/view/10.21037/apm-22-567/rc).
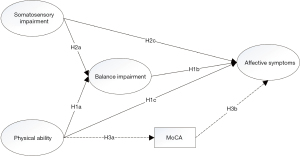
Methods
Participants
Our study was a multicenter cross-sectional clinical trial and used the consecutive sampling method to recruit participants. A total of 1,765 participants with a diagnosis of stroke were enrolled from 10 different inpatient rehabilitation departments, including the Second People’s Hospital of Kunming City, St. John’s Rehabilitation Hospital, the People’s Hospital of Xinping County, the People’s Hospital of Yuxi City, the First People’s Hospital of Qujing City, the People’s Hospital of Kaiyuan City, Kaiyuan Workers’ Hospital, the People’s Hospital of Lufeng County, the People’s Hospital of Baoshan City, the Second Affiliated Hospital of Kunming Medical University, in various cities across Yunnan Province between August 2019 and February 2021. The study was conducted in accordance with the Declaration of Helsinki (as revised in 2013) (35). The research was approved by the ethics committee of the Second Affiliated Hospital of Kunming Medical University (No. PJ-2019-04). All participating hospitals were informed and agreed to the study. Informed consent was taken from all the patients. The inclusion criteria were as follows: (I) ≥18 years old; (II) patients diagnosed with stroke (ischemic or hemorrhagic) confirmed by a neurologist with radiological evidence by either computed tomography (CT) scan and/or magnetic resonance imaging (MRI); (III) stroke of more than one month; (IV) stable non-fluctuating stroke with no acute ongoing neurological development; (V) signed an informed consent. The exclusion criteria were as follows: (I) major depressive or anxiety disorder before stroke; (II) malignancies; (III) severe disease including dementia, loss of consciousness, or renal, hepatic, or heart disease. Finally, 1,058 eligible participants were included, and their important functions were evaluated on the day of admission and discharge using various validated scales addressing physical activity function, somatosensory function, cognitive capability, and functional balance performance (Figure 2).
Assessment
Demographic variables, including the age of onset, gender, lesion location, stroke events, disease duration, medical history, and previous episodes of depression, were collected at the baseline.
Somatosensory measurement
A somatosensory examination was performed by a senior neurologist. The assessment included three subtests; superficial sensation, deep sensation, and complex sensory function. The superficial sensation consisted of three items (light touch sensation, pain, and temperature sensation), the deep sensation had three items (joint position sensation, vibration sensation, and kinesthesia), and the complex sensory functions had four items (two-point discrimination, stereognosis, graphesthesia, and barognosis). One somatosensory assessment was carried out on the affected upper and lower extremities at the time of admission, and was scored as 0 point (normal), 1 point (decreased), and 2 points (absent or hyperalgesia), with higher scores representing poor sensory disturbance.
Physical ability measurement
Physical ability was measured using the Brunnstrom recovery stage (BRS), manual muscle testing (MMT), and the Barthel index (BI) assessment.
BRS (36) is used to assess the motor recovery stage of paresis after stroke (37), including upper and lower extremities due to its acceptable concurrent and predictive validity (38). The recovery process was classified into six stages from 1 (flaccidity) to 6 (near-to-normal motor control), and the total score equaled the sum of the stages of the arm, hand, and leg on the affected side. For example, if an individual suffered left hemiplegia, their hand stage was 1, the upper limb stage was 3, the lower limb stage was 3, and the left total score was 7. High scores indicate positive recovery.
MMT (39,40) is the most commonly used instrument for recording impairment in muscle strength, and consists of six scores from 0 (no contraction) to 5 (normal power). According to the standard procedures, as demonstrated by Hislop and Montgomery (41), the proximal and distal muscle strength in both the upper and lower extremities was assessed by a therapist with 5-year experience. The total score was calculated bilaterally as the sum of all measured muscle groups of elbows (extensors/flexors), wrist (extensors/flexors), hip (extensors/flexors), and knees (extensors/flexors), with a maximum total score of 80. The higher the total score, the better the muscle strength.
BI (42) is regarded as a functional independence tool to measure the patient’s functional ability without family and social help. The total score equals the sum of each item in a range of 0–100. Most items were allocated to three grades: 0 (inability to perform the task), 5 (any assistance required), 10 (total independence). High scores represent an enhanced ability to live independently.
Balance measurement
Balance function was measured using the Lindmark motor capacity assessment for balance (LMCA-B) (43,44), and the scores were verified by an experienced therapist. Two subscales selected to evaluate the function of sitting and standing balance consisted of two items that were scored on a 4-point scale from 0 (cannot sit/cannot stand even with support) to 3 (can sit for 5 min without support/can stand with only slight support of one hand). The total score contains both the sitting and the standing balance scores. A high total score represents better balance function.
The mini-balance evaluation systems test (Mini-BEST) (45) is a developed 14-item scale involving dynamic balance. Each item has three levels: 0 (severe), 1 (moderate), and 2 (normal) and the full score of the test is 28 points. The test assesses several postural control systems through the performance of dynamic balance tasks, such as the capability to react to postural perturbations, to stand on an inclined surface, or to walk while performing a cognitive task. A high score suggests good balance function.
Balance confidence: The activities-specific balance confidence (ABC) scale is a 16-item questionnaire that assesses a patient’s level of balance confidence. The score of each item is range from 0 (no confidence) to 100 (complete confidence) (46). The mean of the 16 items is an individual’s total ABC-score, and a higher score indicates greater balance confidence in daily activities.
Cognitive measurement
The Montreal Cognitive Assessment (MoCA) is an effective screening tool to detect patients with cognitive impairment, and is a one-page 30-point test administered in 10 minutes (47,48). The MoCA tests the following eight cognitive domains: memory; visuospatial ability; executive function; attention; naming task; language; abstraction; and orientation in time and place and was standardized for Chinese participants. The maximum score is 30 points, with lower scores indicating poorer cognitive ability.
Affective symptoms measurement
Affective symptoms include anxiety and depression. Anxiety was measured by the Hamilton Anxiety Scale (HAM-A), and depression was assessed by the Hamilton Depression Scale (HAM-D).
The HAM-A is a 14-item questionnaire (49) commonly used in both clinical and research settings. The items include a series of symptoms and measures representing psychic anxiety and somatic anxiety on a scale of 0 (not present) to 4 (very severe), and high scores indicate high severity in anxiety.
The HAM-D is a 17-item survey (50-52) widely used to clinically quantify the severity of depression. Its scores range from 0 (none) to 4 (very much), with high scores indicating a high severity of depression.
Procedure
The data were collected by four members over 2 years. Firstly, informed consent was obtained, then various neurological assessment instruments, including somatosensory, physical ability, balance impairment, cognitive capability, affective symptoms, and the quality of life, were selected, and data entered into Excel. Subsequently, 10 hospitals were invited to participate in relevant training programs every 3 months, and two trained investigators made irregular visits to different hospitals to collect information and conduct quality control.
Statistical analysis
Structural equation modeling (SEM) was conducted with IBM SPSS/AMOS version 23.0. Missing data were contained in some variables for some patients (<10%). Missing data were assumed to be missing at random and were handled by an imputation process of the maximum likelihood estimation (53). Descriptive statistics was conducted to characterize the participants. The competing models were tested using SEM with latent and observed variables. Latent variables included: (I) physical ability: Brunnstrom, muscle strength, and BI; (II) somatosensory impairment: superficial sensation, deep sensation, and complex sensory function; (III) balance impairment: sitting and standing balance, Mini-BEST, and ABC; (IV) affective symptoms: HAM-D and HAM-A. An Exploratory factor analysis (EFA) was conducted first to construct the measure models. Correlation analysis was performed to evaluate the strength of associations between latent variables and observed variables. Then, the significant observed variables were further analyzed with multiple linear regression analysis to independently explain part of the latent variables. The average variance extracted (AVE) and composite reliability (CR) of each observed variable were calculated to test the convergence validity.
The next stage of the statistical analysis was a path analysis. Based on the above results and proposed hypothesis, a path analysis was conducted to test the relationship between the latent variables. SEM is a multivariate statistical analysis technique including two important stages, the measure model and the structural model. A path analysis, a main analysis in the structural model, is considered as a set of multiple linear regression analysis. Additionally, the structural model was used to estimate the direct and indirect effects of the latent variables proposed. A P value of 0.05 or less indicated statistical significance.
To verify cognitive capability and balance impairment may have the mediating effect between physical ability and affective symptoms, we proposed two competing models to better explain our hypothesis. In model 1, we hypothesized that balance impairment would be a mediator which would affect affective symptoms through somatosensory and physical ability. In this model, we did not take account of cognitive capability. In model 2, the assumptions mentioned above holds, and we added the path that the MoCA variable would be another mediator variable. The physical ability variable may impact the affective symptoms variable through the MoCA variable. In other words, we compared these two models to test the significant effect of the hypothesized causal connections between these relationships on model performance.
The asymptotically distribution-free method was conducted to assess the measurement and structure model (54). To test whether the model fit the data, Hooper et al. suggested the following goodness-of-fit indices (55): the χ2 value, the ratio of χ2 to the degree of freedom (χ2/df<2), the goodness-of-fit index (GFI >0.9), the comparative five index (CFI >0.95), the standardized root mean square residual (SRMR <0.08), the Tucker-Lewis index (TLI >0.95), and root mean square error of approximation (RMSEA <0.06). Multiple model fit statistics were evaluated to determine fit and to provide a robust assessment and overcome limitations of individual tests (56). To compare the most parsimonious model, we used the Akaike information criterion (AIC) and Bayesian information criterion (BIC) to indicate which model was better, and smaller values suggested a good fitting, parsimonious model. Finally, the bootstrap procedure of 2,000 samples for bias-corrected confidence intervals (CI) was used to obtain the significance of overall indirect effects from the model (56).
Results
Sample characteristics
The characteristics of study participants are listed in Table 1, and their mean age was 60.8±12.3 years. The infarction events included 788 (74.5%) ischemic strokes, 266 (25.4%) hemorrhagic strokes, and four (0.4%) undetermined. MoCA scores revealed the below-average levels of cognitive abilities in this sample as 17.3±6.7. The average baseline level of anxiety score was 5.7±6.0, and the average baseline level of depression score was 5.6±6.4.
Table 1
Characteristics | n (%)/mean ± SD |
---|---|
Age, years | 60.8±12.3 |
Male | 679 (64.2) |
Course of the disease (d) | 102.4±431.5 |
Stroke events | |
Ischemic stroke | 788 (74.5) |
Hemorrhagic stroke | 266 (25.4) |
Undetermined | 4 (0.4) |
Stroke lesion sites | |
Left | 516 (48.8) |
Right | 469 (44.3) |
Both | 73 (6.9) |
GCS | 14.9±0.6 |
Brunnstrom | 12.0±4.7 |
Muscle strength | 63.1±15.8 |
BI | 77.6±26.0 |
Superficial sensation | 0.8±1.0 |
Deep sensation | 1.0±1.3 |
Complex sensory function | 1.1±1.5 |
Sitting balance | 2.6±0.7 |
Standing balance | 2.1±0.8 |
Mini-BEST | 15.4±11.3 |
ABC | 50.7±37.7 |
Anxiety | 5.7±6.0 |
Depression | 5.6±6.4 |
MoCA | 17.3±6.7 |
GCS, Glasgow coma scale; BI, Barthel index; Mini-BEST, mini-balance evaluation systems test; ABC, activities-specific balance confidence; MoCA, Montreal Cognitive Assessment.
Reliability and validity
Measurement model analysis
The factor loadings of the observed variables with their latent variables were examined, and Table 2 lists these and the P value of the constructs. Typically, the factor loadings should be >0.7, and the P value should be <0.05, which guarantees the convergent validity of the constructs (57).
Table 2
Latent variable | Observed indicator | Unstd. | λ | S.E. | t | P | R2 | CR | AVE |
---|---|---|---|---|---|---|---|---|---|
Somatosensory impairment | Superficial sensation | 1.000 | 0.846 | 0.717 | 0.918 | 0.789 | |||
Deep sensation | 1.563 | 0.937 | 0.028 | 55.406 | <0.001 | 0.878 | |||
Complex sensory function | 1.695 | 0.879 | 0.040 | 42.205 | <0.001 | 0.773 | |||
Physical ability | Brunnstrom | 1.000 | 0.938 | <0.001 | 0.880 | 0.935 | 0.828 | ||
Muscle strength | 3.095 | 0.909 | 0.064 | 48.371 | <0.001 | 0.826 | |||
BI | 4.966 | 0.882 | 0.124 | 39.929 | <0.001 | 0.778 | |||
Balance impairment | Standing balance | 1.000 | 0.837 | <0.001 | 0.701 | 0.943 | 0.848 | ||
Mini-BEST | 15.155 | 0.973 | 0.291 | 52.123 | <0.001 | 0.946 | |||
ABC | 50.550 | 0.946 | 0.029 | 33.416 | <0.001 | 0.895 | |||
Affective symptoms | Depression | 1.000 | 0.933 | <0.001 | 0.871 | 0.741 | 0.589 | ||
Anxiety | 0.956 | 0.947 | 0.029 | 33.416 | <0.001 | 0.897 |
Unstd., unstandardized estimate; λ, standardized estimate; S.E., standard errors; R2, square multiple correlation; CR, composition reliability; AVE, average variance extracted; BI, Barthel index; Mini-BEST, mini-balance evaluation systems test; ABC, activities-specific balance confidence.
The assessment of the convergent validity and discriminant validity of the measurement model depends on examining the standardized path coefficient, the AVE, and the CR (57). The CR should be >0.60, and the AVE should be >0.50 (58). As shown in Table 2, the CR from all the constructs is >0.60, and the AVE is >0.50, which is considered acceptable, as was the convergent validity of the measurement model. According to the Fornell-Larcker criterion (58), the discriminant validity was evaluated by comparing the square root of each AVE in the diagonal with the correlation coefficients (off-diagonal) for each construct in the relevant rows and columns (Table 3).
Table 3
Latent variable | AVE | Physical ability | Somatosensory impairment | Balance impairment | Affective symptoms |
---|---|---|---|---|---|
Physical ability | 0.828 | 0.910※ | |||
Somatosensory impairment | 0.789 | −0.658 | 0.888※ | ||
Balance impairment | 0.848 | 0.891 | −0.592 | 0.921※ | |
Affective symptoms | 0.589 | −0.422 | 0.281 | −0.464 | 0.767※ |
※, the values of the square root of AVE. These values are greater than the correlations of other constructs, suggesting adequate discriminant validity. AVE, average variance extracted.
Path analysis
Compared two models, the final model (model 2) had adequate model fit indices. In the model 1, the model fit statistics were (χ2=733.96, χ2/df=6.99, GFI =0.938, CFI =0.971, TLI =0.97, SRMR =0.035, RMSEA =0.053, AIC =787.96, BIC =788.58) (Figure 3). However, as we did not take into account the role of cognitive capability, model 2 was set after adding the observed variable of MoCA. The model fit indices were (χ2=243.5, χ2/df=5.181, GFI =0.977, CFI =0.944, TLI =0.922, SRMR =0.063, and RMSEA =0.063, AIC =303.51, BIC =304.26) (Figure 4). The result of analyzing the difference of the variation of the two models was significant (Δχ2=−490.46, Δdf=−58, P<0.05, ΔRMSEA =0.01, ΔSMEA =0.028, ΔAIC=−484.45, ΔBIC =−484.32), although RMSEA and SMEA increased. AIC and BIC decreased in model 2, suggesting this was the more parsimonious model. Compared with these results, model 2 was determined to be the final model in the study.
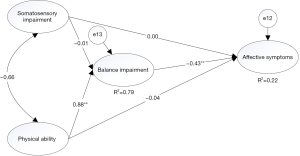
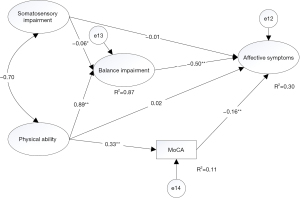
Table 4 indicates the standardized path coefficients and significance for the final model. For the direct effects, all paths were significant, except that from somatosensory impairment to affective symptoms (β=−0.007, P=0.895), and that from physical ability to affective symptoms (β=0.050, P=0.750). Additionally, balance impairment was positively affected by physical ability and negatively affected by somatosensory impairment. Furthermore, these variables explained 87.1% of the variance of balance impairment, while affective symptoms were negatively affected by balance impairment and MoCA, which explained 29.9% of the variance in affective symptoms. MoCA was positively affected by physical ability, and the explanatory power of the variable of MoCA was 11.1%.
Table 4
Paths | Unstd. | β | S.E. | t | P | R2 |
---|---|---|---|---|---|---|
Somatosensory impairment → balance impairment | −0.053 | −0.061 | 0.026 | −2.050 | <0.05* | |
Physical ability → balance impairment | 0.140 | 0.893 | 0.005 | 27.623 | <0.001** | 0.871 |
Balance impairment → affective symptoms | −4.178 | −0.533 | 1.242 | −3.364 | <0.001** | 0.299 |
MoCA → affective symptoms | −0.133 | −0.157 | 0.031 | −4.245 | <0.001** | |
Physical ability → MoCA | 0.479 | 0.329 | 0.044 | 10.828 | <0.001** | 0.110 |
Somatosensory impairment → affective symptoms | −0.049 | −0.007 | 0.370 | −0.131 | 0.895 | |
Physical ability → affective symptoms | 0.061 | 0.050 | 0.192 | 0.318 | 0.750 |
*, P<0.05; **, P<0.001. Unstd., unstandardized path coefficients; β, standardized path coefficients; S.E., standard errors; R2, square multiple correlation; MoCA, Montreal Cognitive Assessment.
Bootstrapping tests were used to verify the indirect effects of each path for somatosensory impairment and physical ability on affective symptoms, and the indirect effect of the physical ability path to affective symptoms through balance impairment and MoCA was significant. The effect size of physical ability through balance impairment on affective symptoms was −1.037 to −0.225 at the bias-corrected 95% CI, which did not include 0 in the range of the effect, and P<0.001 and R2=0.996, suggesting the indirect effect was significant. Additionally, the effect size of physical ability through MoCA on affective symptoms (95% CI: −0.115 to −0.027, P=0.001, R2=0.108) was significant, and the total effect was also statistically significant (95% CI: −0.771 to −0.448, P<0.001). However, the direct effect of the path of physical ability to affective symptoms was not significant, with an effect size of −0.351 to 0.511 (P=0.782). Therefore, balance and cognitive impairment had mediating effects on the relationship between physical ability and affective symptoms (Table 5). On the other hand, the path for somatosensory impairment on affective symptoms through balance impairment was not significant.
Table 5
Effect | Paths | Point estimate | Product of coefficients | Bootstrapping (Bias-corrected 95% CI) | P | R2 | |||
---|---|---|---|---|---|---|---|---|---|
S.E. | Z | Lower | Upper | ||||||
Total effect | Somatosensory impairment → balance impairment → affective symptoms | 0.174 | 0.429 | 0.406 | −0.729 | 0.969 | 0.684 | ||
Physical ability → balance impairment → affective symptoms | −0.587 | 0.079 | −7.430 | −0.771 | −0.448 | <0.001** | |||
Physical ability → MoCA → affective symptoms | −0.587 | 0.079 | −7.430 | −0.771 | −0.448 | <0.001** | |||
Indirect effect | Somatosensory impairment → affective symptoms | 0.223 | 0.141 | 1.582 | 0.015 | 0.631 | 0.112 | ||
Physical ability → affective symptoms | −0.648 | 0.206 | −3.146 | −1.112 | −0.279 | <0.001** | |||
Physical ability → balance impairment → affective symptoms | −0.584 | 0.201 | −2.905 | −1.037 | −0.225 | <0.001** | 0.996 | ||
Physical ability → MoCA → affective symptoms | −0.063 | 0.022 | −2.864 | −0.115 | −0.027 | 0.001* | 0.108 | ||
Direct effect | Somatosensory impairment → affective symptoms | −0.049 | 0.432 | −0.113 | −0.939 | 0.742 | 0.910 | ||
Physical ability → affective symptoms | 0.061 | 0.221 | 0.276 | −0.351 | 0.511 | 0.782 |
*, P<0.05; **, P<0.001. S.E., standard errors; R2, square multiple correlation; MoCA, Montreal Cognitive Assessment.
Discussion
Considering the high prevalence of post-stroke depression/anxiety, this study analyses the factors impacting post-stroke mood disorders and discusses how physical ability influences affective symptoms in patients with stroke. Based on the theory of postural control and the role of cognitive capability, we put forward our research hypotheses. After analyzing the multi-center clinical data using exploratory factor analysis and structural equation model, the results showed that physical ability cannot directly influence post-stroke mood disorders, but can indirectly affect them through two mediating variables: balance impairment and cognitive capability. Nevertheless, balance impairment does not play a mediating role in the relationship between somatosensory impairment and affective symptoms. Additionally, we also found somatosensory impairment significantly negative influenced balance impairment, and physical ability significantly positively affected balance impairment.
Our study provides a new perspective to understand the meditating role of balance function, which has a moderate indirect effect on the latent affective symptoms variable. High balance disturbance is associated with increased mood disorder. This finding is in line with previous evidence linking balance impairment to depression (22,59,60). Similarly, evidence provided by a study on elderly patients suggested that depression problems were associated with postural disturbance (60). Moreover, the finding that balance responds to anxiety is also consistent with the conclusion of the study by Rafsten et al. (61), wherein postural balance function had a moderate correlation with anxiety, which was also reported in other studies (62-64). In summary, these findings demonstrated balance impairment might contribute to mood disorders, including anxiety and depression.
The link between balance and anxiety/depression might indicate the problem of underlying falls in mood disorders, and one study indicated falls may result in depression, leading to a fall problem (59). Balance disorders have been reported as the most common cause of falls in the elderly (65), and demonstrated that improved balance reduced the fear of falls (21). Other studies have also suggested that improving postural control presented a positive trend of decreased fall recurrence significantly (29,66), while in a retrospective study (67), it showed anxiety operated as an outcome of an experienced fall. Moreover, two recent studies demonstrated that fall experience was associated with depression (68,69).
Generally speaking, several putative biological theories may be given to explain the link between it and affective symptoms. Firstly, highly integrated neural networks are responsible for processing emotional information, and balance control and anxiety processing may share similar neural substrates (70). Secondly, attention resources contribute to the postural changes seen in Parkinson’s disease or stroke, such that individuals direct their attention to movements processes, threat-related stimuli, and self-regulatory strategies to compensate for these attentional changes (71). Anxiety feelings are also expressed when threatened (72).
The findings also suggest somatosensory impairment significantly negatively influenced balance impairment (P<0.05, β=−0.061), and physical ability significantly positively affected balance impairment (P<0.001, β=0.893). These results suggest physical ability and somatic sensation are critical factors of balance function, indicating that balance impairment plays a primary role in mood pathology for patients after stroke who have multiple dysfunctions. The current findings could be explained by the theory of core stability, as it was mentioned before. From the perspective of developmental kinesiology, trunk stabilization is a prerequisite for any form of movement and motor performance of the extremities, which is easily observed at the early stages of infant development. For example, in the neonatal stage, a newborn cannot grasp or reach purposefully, and can only appear to have a meaningful limb movement by balancing their head or neck (20). Collectively, this review argues that purposeful movement depends on trunk support. Therefore, core stability is essential for any movement. Multisensory integration is also important for motion. For example, if individuals have insufficient multi-sensory integration, they usually activate too many unnecessary muscles for stabilization and make inefficient movements (73), and it is difficult for such individuals to relax postural muscles, which have negative impacts on postural control. Therefore, understanding a patient’s physical ability and somatosensory impairment has important implications for determining the most effective treatment approach in the context of improving affective symptoms.
In terms of physical therapy, corrective stabilization training or balance exercises may be a primary step in the treatment of anxiety or depression symptoms with patients after stroke. Trunk training can also improve postural control or balance disorders, as well as the fear of falling (21,29). However, no study has yet addressed the direct correlation between stabilization training and mood disorders. A recent systematic review indicated depression was significantly associated with post-stroke physical activity (74), and a prospective cohort study put forth both anxiety had a significant non-linear correlation with physical activity and confirmed the indirect effect of physical activity and mood disorder (75). Nonetheless, additional studies are required to examine whether providing balance training to patients with early stroke as early as possible to complete the target of core stabilization helps reduce anxiety or depression symptoms. Furthermore, these findings demonstrate that various methods are needed to achieve this.
Our findings also showed another indirect path between physical ability and affective symptoms through MoCA was significant. It has previously been shown that motor function is related to global cognition in patients with mild strokes (76), which is in line with our results. Ben Assayag et al. (77) found motor performance was a significant predictor of cognitive decline after stroke. Similarly, one review from the psychological and psychosomatic perspective summarized the dynamic relationship between mind and body, and demonstrated the relationship shared the same neural substrates that may help translate thoughts into feelings (78).
Practical implications
The current study provides significant practical implication in designing comprehensive rehabilitation interventions. It is helpful for scholars to target the relevant factors together to improve affective symptoms after stroke. First, given the role of balance function in our study, rehabilitation professionals could pay more attention to the balance function which will help to strengthen physical ability and improve post-stroke mood disorders. Family caregivers should take into account patients’ balance impairment to protect their safety in an effective manner. Second, our study also found an important role of cognitive capability. Rehabilitation doctors and therapists should deal with the cognitive capability of patients with stroke to reduce patients’ risk post-stroke anxiety or post-stroke depression. In this way, rehabilitation professionals can integrate the various assessment results into treatment plans, resulting in more comprehensive and targeted interventions.
Limitations
The current study utilized an SEM approach to examine the relationship between balance deficits, somatosensory impairment, physical ability, cognitive capability, and mood disorders. Nevertheless, some limitations are present. The data were collected in a cross-sectional design, and Bullock et al. suggested longitudinal studies are needed to explore any causal association (79). Although the present research findings cannot directly conclude the causality, scientific assumptions and derivations can provide important information. Consequently, further studies should be designed to clarify the causal correlation between balance deficits and affective symptoms. Moreover, we did not employ standardized scales to assess the sensory and balance deficits but relied on the clinician’s experience to evaluate a specific construct. Also, the latent affective symptoms variable in this study was defined by only two measured variables. Therefore, these variables cannot accurately represent the true construction, and further studies using different indicators are needed to verify the robustness of the current results.
Conclusions
This cross-sectional study confirmed balance impairment mainly mediated the relationship between physical ability and affective symptoms, while cognitive capability can play a slightly mediating role in the relationship between physical ability and affective symptoms. The findings may help rehabilitation professionals target effective interventions and enhance balance performance to improve mental status.
Acknowledgments
The authors wish to thank participants in the study.
Funding: The study was supported by the Major Science and Technology Project of Yunnan Province (No. 2018zf016), Rehabilitation Clinical Medical Centre of Yunnan Province (zx2019-04-02), National Key Research and Development Program (2018YFC2002301), and Jiajie Expert Workstation of Yunnan Province (2019IC034).
Footnote
Reporting Checklist: The authors have completed the STROBE reporting checklist. Available at https://apm.amegroups.com/article/view/10.21037/apm-22-567/rc
Data Sharing Statement: Available at https://apm.amegroups.com/article/view/10.21037/apm-22-567/dss
Conflicts of Interest: All authors have completed the ICMJE uniform disclosure form (available at https://apm.amegroups.com/article/view/10.21037/apm-22-567/coif). The authors have no conflicts of interest to declare.
Ethical Statement: The authors are accountable for all aspects of the work in ensuring that questions related to the accuracy or integrity of any part of the work are appropriately investigated and resolved. The study was conducted in accordance with the Declaration of Helsinki (as revised in 2013). The research was approved by the ethics committee of the Second Affiliated Hospital of Kunming Medical University (No. PJ-2019-04). Informed consent was taken from all the patients.
Open Access Statement: This is an Open Access article distributed in accordance with the Creative Commons Attribution-NonCommercial-NoDerivs 4.0 International License (CC BY-NC-ND 4.0), which permits the non-commercial replication and distribution of the article with the strict proviso that no changes or edits are made and the original work is properly cited (including links to both the formal publication through the relevant DOI and the license). See: https://creativecommons.org/licenses/by-nc-nd/4.0/.
References
- Struijs JN, van Genugten ML, Evers SM, et al. Future costs of stroke in the Netherlands: the impact of stroke services. Int J Technol Assess Health Care 2006;22:518-24. [Crossref] [PubMed]
- Katan M, Luft A. Global Burden of Stroke. Semin Neurol 2018;38:208-11. [Crossref] [PubMed]
- Feigin VL, Norrving B, Mensah GA. Global Burden of Stroke. Circ Res 2017;120:439-48. [Crossref] [PubMed]
- Ahlsiö B, Britton M, Murray V, et al. Disablement and quality of life after stroke. Stroke 1984;15:886-90. [Crossref] [PubMed]
- Haghgoo HA, Pazuki ES, Hosseini AS, et al. Depression, activities of daily living and quality of life in patients with stroke. J Neurol Sci 2013;328:87-91. [Crossref] [PubMed]
- Brown C, Hasson H, Thyselius V, et al. Post-stroke depression and functional independence: a conundrum. Acta Neurol Scand 2012;126:45-51. [Crossref] [PubMed]
- Schöttke H, Giabbiconi CM. Post-stroke depression and post-stroke anxiety: prevalence and predictors. Int Psychogeriatr 2015;27:1805-12. [Crossref] [PubMed]
- Li W, Xiao WM, Chen YK, et al. Anxiety in Patients With Acute Ischemic Stroke: Risk Factors and Effects on Functional Status. Front Psychiatry 2019;10:257. [Crossref] [PubMed]
- Carvalho-Pinto BP, Faria CD. Health, function and disability in stroke patients in the community. Braz J Phys Ther 2016;20:355-66. [Crossref] [PubMed]
- Paprocka-Borowicz M, Wiatr M, Ciałowicz M, et al. Influence of Physical Activity and Socio-Economic Status on Depression and Anxiety Symptoms in Patients after Stroke. Int J Environ Res Public Health 2021;18:8058. [Crossref] [PubMed]
- Lin C, Babiker A, Srdanovic N, et al. Depressive symptoms after stroke are associated with worse recovery. Int J Psychiatry Med 2020;55:227-38. [Crossref] [PubMed]
- Boudokhane S, Migaou H, Kalai A, et al. Predictors of Quality of Life in Stroke Survivors: A 1-year Follow-Up Study of a Tunisian Sample. J Stroke Cerebrovasc Dis 2021;30:105600. [Crossref] [PubMed]
- Alghwiri AA. The Correlation between Depression, Balance, and Physical Functioning Post Stroke. J Stroke Cerebrovasc Dis 2016;25:475-9. [Crossref] [PubMed]
- Yuan S, He Y. Effects of physical therapy on mental function in patients with stroke. J Int Med Res 2020;48:300060519861164. [Crossref] [PubMed]
- Gupta MA, Gupta AK. Cutaneous sensory disorder. Semin Cutan Med Surg 2013;32:110-8. [Crossref] [PubMed]
- Massion J. Movement, posture and equilibrium: interaction and coordination. Prog Neurobiol 1992;38:35-56. [Crossref] [PubMed]
- Kibler WB, Press J, Sciascia A. The role of core stability in athletic function. Sports Med 2006;36:189-98. [Crossref] [PubMed]
- Sibley KM, Mochizuki G, Lakhani B, et al. Autonomic contributions in postural control: a review of the evidence. Rev Neurosci 2014;25:687-97. [Crossref] [PubMed]
- Cabanas-Valdés R, Bagur-Calafat C, Girabent-Farrés M, et al. Long-term follow-up of a randomized controlled trial on additional core stability exercises training for improving dynamic sitting balance and trunk control in stroke patients. Clin Rehabil 2017;31:1492-9. [Crossref] [PubMed]
- Kobesova A, Kolar P. Developmental kinesiology: three levels of motor control in the assessment and treatment of the motor system. J Bodyw Mov Ther 2014;18:23-33. [Crossref] [PubMed]
- Gusi N, Carmelo Adsuar J, Corzo H, et al. Balance training reduces fear of falling and improves dynamic balance and isometric strength in institutionalised older people: a randomised trial. J Physiother 2012;58:97-104. [Crossref] [PubMed]
- Khan F, Chevidikunnan MF. Prevalence of Balance Impairment and Factors Associated with Balance among Patients with Stroke. A Cross Sectional Retrospective Case Control Study. Healthcare (Basel) 2021;9:320. [Crossref] [PubMed]
- Verheyden G, Vereeck L, Truijen S, et al. Trunk performance after stroke and the relationship with balance, gait and functional ability. Clin Rehabil 2006;20:451-8. [Crossref] [PubMed]
- Januário F, Campos I, Amaral C. Rehabilitation of postural stability in ataxic/hemiplegic patients after stroke. Disabil Rehabil 2010;32:1775-9. [Crossref] [PubMed]
- Nitz J, Gage A. Post stroke recovery of balanced sitting and ambulation ability. Aust J Physiother 1995;41:263-7. [Crossref] [PubMed]
- Franchignoni FP, Tesio L, Ricupero C, et al. Trunk control test as an early predictor of stroke rehabilitation outcome. Stroke 1997;28:1382-5. [Crossref] [PubMed]
- Di Monaco M, Trucco M, Di Monaco R, et al. The relationship between initial trunk control or postural balance and inpatient rehabilitation outcome after stroke: a prospective comparative study. Clin Rehabil 2010;24:543-54. [Crossref] [PubMed]
- Verheyden G, Nieuwboer A, De Wit L, et al. Trunk performance after stroke: an eye catching predictor of functional outcome. J Neurol Neurosurg Psychiatry 2007;78:694-8. [Crossref] [PubMed]
- Mat S, Ng CT, Tan PJ, et al. Effect of Modified Otago Exercises on Postural Balance, Fear of Falling, and Fall Risk in Older Fallers With Knee Osteoarthritis and Impaired Gait and Balance: A Secondary Analysis. PM R 2018;10:254-62. [Crossref] [PubMed]
- Lin WQ, Huang TY, Liu L, et al. Prevalence and related factors of depression and falls among the elderly living in rural communities of Guangzhou. Psychol Health Med 2020;25:980-8. [Crossref] [PubMed]
- French MA, Miller A, Pohlig RT, et al. Depressive Symptoms Moderate the Relationship Among Physical Capacity, Balance Self-Efficacy, and Participation in People After Stroke. Phys Ther 2021;101:pzab224.
- Meijer R, van Limbeek J, Peusens G, et al. The Stroke Unit Discharge Guideline, a prognostic framework for the discharge outcome from the hospital stroke unit. A prospective cohort study. Clin Rehabil 2005;19:770-8. [Crossref] [PubMed]
- Middleton LE, Barnes DE, Lui LY, et al. Physical activity over the life course and its association with cognitive performance and impairment in old age. J Am Geriatr Soc 2010;58:1322-6. [Crossref] [PubMed]
- Williams OA, Demeyere N. Association of Depression and Anxiety With Cognitive Impairment 6 Months After Stroke. Neurology 2021;96:e1966-74. [Crossref] [PubMed]
- World Medical Association. World Medical Association Declaration of Helsinki: ethical principles for medical research involving human subjects. JAMA 2013;310:2191-4. [Crossref] [PubMed]
- Sawner K JL. Brunnstrom movement therapy in hemiplegia: a neurophysiological approach. 2nd ed. Philadelphia: JB Lippincott; 1992.
- Arya KN, Pandian S, Kumar D, et al. Task-Based Mirror Therapy Augmenting Motor Recovery in Poststroke Hemiparesis: A Randomized Controlled Trial. J Stroke Cerebrovasc Dis 2015;24:1738-48. [Crossref] [PubMed]
- Naghdi S, Ansari NN, Mansouri K, et al. A neurophysiological and clinical study of Brunnstrom recovery stages in the upper limb following stroke. Brain Inj 2010;24:1372-8. [Crossref] [PubMed]
- Cuthbert SC, Goodheart GJ Jr. On the reliability and validity of manual muscle testing: a literature review. Chiropr Osteopat 2007;15:4. [Crossref] [PubMed]
- Bohannon RW. Manual muscle testing: does it meet the standards of an adequate screening test? Clin Rehabil 2005;19:662-7. [Crossref] [PubMed]
- Hislop HJ, Montgomery J. Daniels and Worthingham's muscle testing. Techniques of manual examination, sixth edition. Philadelphia, PA: WB Saunders Co; 1995.
- Mahoney FI, Barthel DW. Functional evaluation: the Barthel index. Md State Med J 1965;14:61-5. [PubMed]
- Lindmark B, Hamrin E. Evaluation of functional capacity after stroke as a basis for active intervention. Presentation of a modified chart for motor capacity assessment and its reliability. Scand J Rehabil Med 1988;20:103-9. [PubMed]
- Ytterberg C, Einarsson U, Holmqvist LW, et al. A population-based study of fall risk factors among people with multiple sclerosis in Stockholm county. J Rehabil Med 2013;45:452-7. [Crossref] [PubMed]
- Franchignoni F, Horak F, Godi M, et al. Using psychometric techniques to improve the Balance Evaluation Systems Test: the mini-BESTest. J Rehabil Med 2010;42:323-31. [Crossref] [PubMed]
- Powell LE, Myers AM. The Activities-specific Balance Confidence (ABC) Scale. J Gerontol A Biol Sci Med Sci 1995;50A:M28-34. [Crossref] [PubMed]
- Nasreddine ZS, Phillips NA, Bédirian V, et al. The Montreal Cognitive Assessment, MoCA: a brief screening tool for mild cognitive impairment. J Am Geriatr Soc 2005;53:695-9. [Crossref] [PubMed]
- Kang JM, Cho YS, Park S, et al. Montreal cognitive assessment reflects cognitive reserve. BMC Geriatr 2018;18:261. [Crossref] [PubMed]
- HAMILTON M. The assessment of anxiety states by rating. Br J Med Psychol 1959;32:50-5. [Crossref] [PubMed]
- HAMILTON M.. A rating scale for depression. J Neurol Neurosurg Psychiatry 1960;23:56-62. [Crossref] [PubMed]
- Fava GA, Kellner R, Munari F, et al. The Hamilton Depression Rating Scale in normals and depressives. Acta Psychiatr Scand 1982;66:26-32. [Crossref] [PubMed]
- Williams JB. Standardizing the Hamilton Depression Rating Scale: past, present, and future. Eur Arch Psychiatry Clin Neurosci 2001;251:II6-12. [Crossref] [PubMed]
- Dong Y, Peng CY. Principled missing data methods for researchers. Springerplus 2013;2:222. [Crossref] [PubMed]
- Bollen KA, Stine RA. Bootstrapping Goodness-of-Fit Measures in Structural Equation Models. Sociol Methods Res 1992;21:205-29. [Crossref]
- Hooper D, Coughlan J, Mullen MR, et al. Structural Equation Modelling Guidelines for Determining Model Fit. J Bus Res 2008;6:53-60.
- Kline RB. Principles and Practice of Structural Equation Modeling, 4th ed. New York: Guilford Press; 2016.
- Bagozzi RP, Yi Y. On the evaluation of structural equation models. J Acad Mark Sci 1988;16:74-94. [Crossref]
- Fornell C, Larcker DF. Structural Equation Models With unobservable Variables and measurement error: Algebra and Statistics. J Mark Res 1981;18:382-8. [Crossref]
- Alenazi AM, Alshehri MM, Alothman S, et al. Functional Reach, Depression Scores, and Number of Medications Are Associated With Number of Falls in People With Chronic Stroke. PM R 2018;10:806-16. [Crossref] [PubMed]
- Turcu A, Toubin S, Mourey F, et al. Falls and depression in older people. Gerontology 2004;50:303-8. [Crossref] [PubMed]
- Rafsten L, Danielsson A, Sunnerhagen KS. Self-perceived postural balance correlates with postural balance and anxiety during the first year after stroke: a part of the randomized controlled GOTVED study. BMC Neurol 2020;20:410. [Crossref] [PubMed]
- Schmid AA, Arnold SE, Jones VA, et al. Fear of falling in people with chronic stroke. Am J Occup Ther 2015;69:6903350020. [Crossref] [PubMed]
- Torkia C, Best KL, Miller WC, et al. Balance Confidence: A Predictor of Perceived Physical Function, Perceived Mobility, and Perceived Recovery 1 Year After Inpatient Stroke Rehabilitation. Arch Phys Med Rehabil 2016;97:1064-71. [Crossref] [PubMed]
- Schmid AA, Van Puymbroeck M, Knies K, et al. Fear of falling among people who have sustained a stroke: a 6-month longitudinal pilot study. Am J Occup Ther 2011;65:125-32. [Crossref] [PubMed]
- Cuevas-Trisan R. Balance Problems and Fall Risks in the Elderly. Clin Geriatr Med 2019;35:173-83. [Crossref] [PubMed]
- Barnett A, Smith B, Lord SR, et al. Community-based group exercise improves balance and reduces falls in at-risk older people: a randomised controlled trial. Age Ageing 2003;32:407-14. [Crossref] [PubMed]
- Bloch F, Blandin M, Ranerison R, et al. Anxiety after a fall in elderly subjects and subsequent risk of developing post traumatic stress disorder at two months. A pilot study. J Nutr Health Aging 2014;18:303-6. [Crossref] [PubMed]
- Park HJ, Lee NG, Kang TW. Fall-related cognition, motor function, functional ability, and depression measures in older adults with dementia. NeuroRehabilitation 2020;47:487-94. [Crossref] [PubMed]
- Kim JH. Experiences of falling and depression: Results from the Korean Longitudinal Study of Ageing. J Affect Disord 2021;281:174-82. [Crossref] [PubMed]
- Balaban CD. Neural substrates linking balance control and anxiety. Physiol Behav 2002;77:469-75. [Crossref] [PubMed]
- Adkin AL, Carpenter MG. New Insights on Emotional Contributions to Human Postural Control. Front Neurol 2018;9:789. [Crossref] [PubMed]
- Johnson KJ, Zaback M, Tokuno CD, et al. Exploring the relationship between threat-related changes in anxiety, attention focus, and postural control. Psychol Res 2019;83:445-58. [Crossref] [PubMed]
- Polatajko HJ, Cantin N. Developmental coordination disorder (dyspraxia): an overview of the state of the art. Semin Pediatr Neurol 2005;12:250-8. [Crossref] [PubMed]
- Thilarajah S, Mentiplay BF, Bower KJ, et al. Factors Associated With Post-Stroke Physical Activity: A Systematic Review and Meta-Analysis. Arch Phys Med Rehabil 2018;99:1876-89. [Crossref] [PubMed]
- Thilarajah S, Bower KJ, Pua YH, et al. Modifiable Factors Associated With Poststroke Physical Activity at Discharge From Rehabilitation: Prospective Cohort Study. Phys Ther 2020;100:818-28. [Crossref] [PubMed]
- Einstad MS, Saltvedt I, Lydersen S, et al. Associations between post-stroke motor and cognitive function: a cross-sectional study. BMC Geriatr 2021;21:103. [Crossref] [PubMed]
- Ben Assayag E, Shenhar-Tsarfaty S, Korczyn AD, et al. Gait measures as predictors of poststroke cognitive function: evidence from the TABASCO study. Stroke 2015;46:1077-83. [Crossref] [PubMed]
- Critchley HD. Psychophysiology of neural, cognitive and affective integration: fMRI and autonomic indicants. Int J Psychophysiol 2009;73:88-94. [Crossref] [PubMed]
- Bullock HE, Harlow LL, Mulaik SA. Causation issues in structural equation modeling research. Struct Equ Modeling 1994;1:253-67. [Crossref]
(English Language Editor: B. Draper)