Effect of ex vivo lung perfusion on storage of isolated lungs
Introduction
Lung transplantation is the ultimate treatment option for patients with multiple end-stage lung disease, such as chronic obstructive pulmonary disease (1) and idiopathic pulmonary fibrosis (2). However, the shortage of qualified donor lungs is one of the main limiting factors for clinical lung transplantation. It is estimated that only approximately 15% of donor lungs in China are currently suitable for lung transplantation (3). Many potential donor lungs have primary infections or are not adequately maintained after they are harvested, leading to problems such as infection, barotrauma and pulmonary edema in the donor lung. These lungs ultimately cannot be used for transplantation or lead to transplantation failure.
Ex vivo lung perfusion (EVLP) is a method to preserve and repair marginal donor lungs and shows great potential. EVLP can provide a real-time assessment of donor lungs and repair marginal donor lungs to a certain extent. EVLP was first used for in vitro evaluation of donor lungs in 2001 (4) and first used for transplantation after marginal donor lung repair in 2007 (5). The first large-scale clinical trial was carried out in 2011 (6). At present, EVLP is an available procedure in several foreign large lung transplant centers, including the Toronto Lung Transplant Program, University Health Network, University of Toronto (7), Royal Brompton & Harefield NHS Foundation Trust, Harefield Hospital (8), and the University of Maryland Medical Center (9). However, the current EVLP research in China is still in its infancy, and very few studies have reported on the use of EVLP in clinical lung transplantation or basic research. To date, only EVLP studies involving rats or dogs have been reported (10,11). To that end, this study evaluated the effectiveness of an EVLP system that was co-developed with the Guangdong Shunde Innovation Design and Research Institute to perfuse human nonacceptable donor lungs and porcine lungs in vitro. Lung function and blood gas content were determined to assess the preservation effect of EVLP on a donor lung in vitro and to provide a reference for subsequent research and clinical application of EVLP in China.
Methods
Human nonacceptable donor lungs
Nonacceptable donor lungs were obtained from three donors because they did not meet the conditions for lung transplantation after harvesting. EVLP was performed at room temperature in vitro after consent was obtained from the donor’s family and approval of the study by the ethical committee.
The conditions of the three donor lungs are described as follows: (I) in November 2018, A 33-year-old male (Chinese nationality) voluntarily donated his lungs. His blood type was A, and his weight was 72 kg. He was diagnosed with brain death after severe brain injury. Bronchoscopy showed a large amount of sputum in the lungs, which indicated that the lungs could not be used for clinical transplantation. The duration of intubation was 5 days, and the oxygenation index was 293 mmHg. A chest radiography was unremarkable. Bronchoscopy showed edema of the airway and a moderate amount of yellow sticky sputum in the posterior segment of the right lower lobe; (II) in November 2018, a 45-year-old male (Chinese nationality) voluntarily donated his lungs. His blood type was AB, and his weight was 80 kg. He was diagnosed with brain death after cerebral hemorrhage. Bronchoscopy showed a large amount of sputum in the lungs, indicating that the lungs could not be used for clinical transplantation. The duration of intubation was 6 days, and the oxygenation index was 357 mmHg. A chest radiography was unremarkable. Bronchoscopy showed a moderate amount of white sputum in the right upper lobe and the basal segment of the right lower lobe; (III) in November 2018, a 37-year-old male (Chinese nationality) voluntarily donated his lungs. His blood type was B, and his weight was 70 kg. He was diagnosed with brain death after cerebral hemorrhage. He tested positive for syphilis antibody. The duration of intubation was 3 days, and the oxygenation index was 480 mmHg. A chest radiography was unremarkable. Bronchoscopy showed edema of the airway and a small amount of white sputum in the opening of each segment of both lungs.
Experimental animals
Three-breed cross, male swine weighing 25±1.5 kg were purchased from Jiangsu Yadong Experimental Animal Research Institute. The swine were fasted for 12 h before the experiment.
Equipment
The equipment included a customized EVLP mechanical perfusion system, Savina ventilator (Drager, Germany), PM-9000A multi-parameter patient monitor (Hunan Ruibo Technology Co., Ltd.) and i-STAT blood gas analyzer (including CG8 + blood gas test card, Abbott, USA).
Drugs
Dextran 40 [Biotech Bioengineering (Shanghai) Co., Ltd.], 10% human serum albumin (Shanghai Laishi Blood Products Co., Ltd.), methylprednisolone injection (Pfizer Pharmaceuticals Ltd, USA), Cefazolin injection (Suzhou Erye Pharmaceutical Co., Ltd.), heparin sodium injection (Maanshan Fengyuan Pharmaceutical Co., Ltd.), Propofol injection (Xi’an Libang Pharmaceutical Co., Ltd.), Atropine sulfate injection (Shanghai Quanyu Biotechnology) Zhumadian Animal pharmaceutical Co., Ltd.), Shutai injection (Virbac, France), Sumianxin injection (Dunhua Shengda Animal Pharmaceutical Co., Ltd.), sodium chloride, potassium chloride, calcium chloride, disodium hydrogen phosphate, magnesium sulfate, magnesium chloride, sodium bicarbonate, anhydrous glucose (GLU) and mannitol were purchased from Shanghai McLean Biochemical Technology Co., Ltd. Tromethamine was purchased from Sigma Aldrich, USA, and prepared as a 3.3 mmol/mL solution before use.
Study methods
Preparation of customized perfusion solution
The perfusion solution contained the following (per liter): 5 g Dextran-40, 3.8 g sodium chloride, 0.34 g potassium chloride, 0.12 g calcium chloride, 0.17 g disodium hydrogen phosphate, 0.12 g magnesium chloride, 1.26 g sodium bicarbonate, and 2 g GLU. The solution was filtered through a 0.22 µm filter before use. After filtration, 350 mL of 10% albumin, 1 g of methylprednisolone, 1 g of cefazolin sodium and 25,000 µL of heparin sodium were added to each 750 mL of filtrated solution.
Preparation of raffinose-modified low potassium dextran (R-LPD) solution
Each 1 L of R-LPD solution contained 50 g Dextran-40, 8 g sodium chloride, 0.4 g potassium chloride, 0.12 g calcium chloride, 0.046 g disodium hydrogen phosphate, 0.063 g potassium dihydrogen phosphate, 0.1 mg magnesium sulfate, 1.818 g GLU and 0.3 mL tromethamine. The solution was filtered through a 0.22 µm filter before use.
Human donor lung harvest
Under the supervision and participation of the Red Cross and with the consent of the donor’s family and ethical discussions, we harvested the donor lung and performed EVLP at room temperature to repair the donor lung. The donor lung harvests were performed with reference to the Technical specification for donor lung procurement and protection of lung transplantation in China (2019 edition) (12) issued by the Organ Transplantation Branch of the Chinese Medical Association.
EVLP for nonacceptable donor lungs
In this study, a customized ex vivo lung normal temperature mechanical perfusion system was used based on a Toronto lung EVLP protocol.
The donor lungs were transported to our central organ transplantation laboratory after harvest and stored at 4 °C for 12 h. Right lung EVLP was performed in each case. The perfusion tubes were primed with the customized solution before starting. The perfusion system was examined to ensure no fluid leakage was occurring and no air bubbles were present in the tubes. Then, the EVLP system was connected to the arterial end of the donor lung while the venous end was left open. The water bath system was used to control the perfusion temperature. Initially, the perfusion temperature was set to 12 °C and then was raised to 32 °C within 20 min. Pressure-controlled ventilation mode was used for ventilator ventilation, with the following parameters: tidal volume 6–8 mL/kg, inhaled oxygen concentration (FiO2) 21%, ventilation frequency 7 times/min, positive end-expiratory pressure (PEEP) 5 cmH2O and inspiratory ratio 1:2. Deoxygenated gas (86% N2, 8% CO2, 6% O2) was used for deoxygenation of the arterial perfusate. Blood gas was measured and used as the basic value when mechanical ventilation had been performed for 10 min and the perfusion temperature had risen to 37 °C. A centrifugal pump was used for perfusion at a constant flow rate. The perfusion rate began at 10% of the full flow rate (estimated to be 40% of cardiac output), was increased by 10% every 10 min during the first 20 min, and then was increased by 50%, 80%, and 100% at 30, 40, and 50 min after start, respectively (13).
EVLP of the isolated porcine lungs
The perfusion tubes were primed with the customized solution before starting. The perfusion system was examined to ensure no fluid leakage was occurring and no air bubbles were present in the tubes. Then, the EVLP system was connected to the donor porcine lungs. The water bath system was used to control the perfusion temperature. Initially, the perfusion temperature was set to 12 °C and then was raised to 32 °C within 20 min. After the temperature reached 32 °C, mechanical ventilation was started. The ventilator parameters were as follows: tidal volume 7 mL/kg, FiO2 21%, ventilation frequency 7 times/min, PEEP 5 cmH2O, and inspiratory ratio 1: 2. Deoxygenated gas (86% N2, 8% CO2, and 6% O2) was used to deoxygenate the perfusion fluid at the arterial end. At the same time, the temperature continued rising to 37 °C. A centrifugal pump was used for perfusion at a constant flow rate. The perfusion rate was started at 10% of the full flow rate [body weight (kg) ×0.11 L/kg/min ×40%], was increased by 10% every 10 min during the first 20 min, and then increased to 100% within 50 min after start. The adjustment of the perfusion rate followed the same pattern as that described in section 1.5.4.
Lung re-expansion and examination of parameters
The blood gas index was measured every 1 h during the 4 h of EVLP perfusion. Before sampling, the FiO2 was adjusted to 100%, the tidal volume was adjusted to 10 mL/kg, and the ventilation frequency was adjusted to 10 times/min. Sampling was performed after 5 min of ventilation with the above parameters. The parameters were adjusted back to those before sampling. Pulmonary re-expansion was performed 30 min after each blood gas analysis sampling. When lung re-expansion was performed, the tidal volume was adjusted to 14 mL/kg, the ventilation frequency was adjusted to 7 times/min, and the remaining ventilator parameters remained unchanged. Continuous ventilation was performed for 20 seconds and then once more. The airway peak pressure did not exceed 25 cmH2O, and the oxygen concentration was 100%. The blood gas analysis and lung re-expansion procedures were the same in both the nonacceptable donor lung and porcine lung experiments.
Data analysis
SPSS 22.0 was used for statistical analysis. A normal distribution test and homogeneity test of variance were performed before mean comparison. Data that met the normal distribution and homogeneity of variance criteria were analyzed via ANOVA. Otherwise, a Kruskal-Wallis rank sum test was used.
Results
Figures 1 and 2 present photographs of EVLP of a human nonacceptable donor lung and a porcine lung. The lung function and blood gas analysis results are shown in Tables 1 and 2.
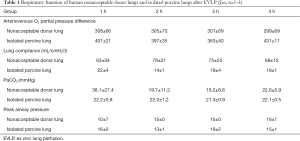
Full table
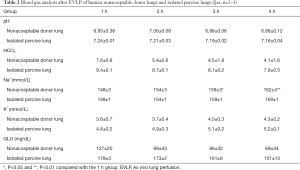
Full table
According to Figures 1 and 2, the basic morphology of the lungs was maintained in both the experiments with nonacceptable donor lungs and with isolated porcine lungs. No obvious large areas of congestion were noted, which suggests that EVLP did not cause significant damage to the isolated lungs.
According to Table 1, during EVLP, the arterial and venous oxygen partial pressure difference of a human nonacceptable donor lung can be basically maintained at approximately 300 mmHg, and the lung compliance can be maintained above 60 mL/cmH2O. There were no significant differences at any time point. Good and stable arteriovenous oxygen partial pressure difference and lung compliance were also maintained during EVLP perfusion of the porcine lungs, and no significant differences were observed at any time points. The peak pressure of the airways in human nonacceptable donor lungs and in porcine lungs during EVLP was basically stable. The above results show good oxygen exchange and compliance in the normal physiological range during EVLP. This finding suggests that EVLP can maintain the respiratory function of isolated lungs.
As seen from Tables 1,2, during the EVLP perfusion, PaCO2, pH, and HCO3- in the human nonacceptable donor lungs were lower than the normal physiological range. There were no significant differences at any time point. Although the in vitro porcine lung had normal PaCO2 during EVLP perfusion, the pH and HCO3- were lower than the normal physiological values (14). This result suggests that the human nonacceptable donor lung and in vitro porcine lung presented with metabolic acidosis during the entire course of EVLP.
The levels of Na+ and K+ in the human nonacceptable donor lungs and isolated porcine lungs were within the normal physiological range before the start of EVLP. Over the course of EVLP, the levels of Na+ in the isolated lungs gradually increased. The Na+ levels in the human nonacceptable donor lung group were significantly higher 3 h after perfusion than before EVLP. The Na+ level in the isolated porcine lungs also gradually increased during EVLP. In addition, although the K+ levels in both the human nonacceptable donor lungs and isolated porcine lungs remained within the normal physiological range after 4 h of EVLP, a gradual upward trend was noted. Notably, the changes in the levels of Na+ and K+ suggest that electrolyte disorders may occur in the isolated lungs during EVLP.
Before the start of EVLP, the GLU level in the human nonacceptable donor lungs and the isolated porcine lungs was higher than the normal value (based on the molecular weight of GLU (180 g/mol) and a GLU conversion factor of 18, i.e., 1 mg/dL =18 mmol/L; the fasting GLU physiological range is 70–110 mg/dL in a normal person). The abnormal increase in GLU levels may be related to the stress response produced by the body when the lungs were harvested. However, as the EVLP progressed, the GLU levels in the isolated lungs gradually decreased and became normal, suggesting that EVLP can decrease the high GLU level in the lungs caused by the stress response. The decline in the GLU level may also be related to the gradual improvement of isolated lung function. The lungs gradually return to normal energy metabolism during EVLP, and GLU consumption during respiration might lead to a decline in GLU levels.
Discussion
EVLP has a wide range of application prospects. According to the literature, compared with traditional cold preservation, EVLP can prolong the preservation time of isolated donor lungs (15). The one-year survival rate and cumulative survival rate of patients receiving EVLP repair of a marginal donor lung for transplantation are not significantly different from those of conventional lung transplant recipients (16), suggesting that EVLP can be used to select marginal donor lungs that have the potential to be repaired and then to repair the donor lungs. This process can improve the quality of marginal donor lungs and increase the donor lung utilization rate. EVLP provides a repair platform for isolated donor lungs and can effectively eliminate atelectasis via mechanical perfusion, reduce the degree of hypoxic vasoconstriction, and remove small blood clots from the pulmonary micro vessels through retrograde perfusion to reduce embolism of donor pulmonary vessels. In addition, the EVLP system is expected to be able to evaluate and interfere with the immune regulation of donor lungs in vitro. Tissue, cell, and cytokine-related transplant immunology research based on the EVLP system is gradually becoming a hotspot and research trend in the field of lung transplantation (17). However, research on transplant immunology based on the EVLP platform is still lacking in China. Because immune regulation is affected by a variety of factors, such as protein expression levels, gene polymorphisms, and the in vivo microenvironment, the related factors can show significant differences among different races. Therefore, before promotion and application of the EVLP technique in China, large animal experiments and preclinical nonacceptable donor lung experiments should be conducted in China to explore the adaptability of EVLP in the field of lung transplantation in China and determine the suitable protocol for Chinese donor lungs.
The basic principle of the EVLP technique is to simulate the gas exchange process of the lung blood circulation in vitro. Deoxygenated perfusion fluid is sent to the isolated lung for oxygenation to evaluate the functionality of the isolated lung through real-time monitoring of lung function and blood gas indicators (Figure 3). In the mechanical perfusion system, a leukocyte filter and a microemboli filter can be added to further purify the perfusion fluid returned to the isolated lung to improve the perfusion effect.
At present, the EVLP system and its related supplies used in China are imported, and the high cost of EVLP has become a hurdle for promotion and application of this technique in China. Therefore, in this study, we used an isolated lung normal temperature mechanical perfusion system (a research engineering machine for research and development) developed by Wuxi People’s Hospital and Guangdong Shunde Innovative Design Institute for EVLP perfusion. To confirm the stability of the mechanical perfusion function of this system, nonacceptable donor lungs and isolated porcine lungs were tested with EVLP perfusion. The results of the human nonacceptable donor lung experiments and large animal experiments showed that the independently developed system could continuously and mechanically perfuse the isolated lungs for at least 4 h.
EVLP can effectively maintain the respiratory function of isolated lungs. The isolated lungs can maintain good oxygen uptake and lung compliance. The reason may be that during EVLP, a ventilation environment suitable for the physiology of the lungs is maintained, and the physiological substances needed for lung metabolism are provided by the perfusion solution. Therefore, the isolated lung can maintain its respiratory function under good conditions. In this study, a protective ventilation strategy and a gradient-increasing flow treatment method were used in EVLP to avoid lung damage that may be caused by an excessive tidal volume or perfusion flow rate. In addition, glucocorticoids and antibiotics were added to the perfusate in this study in order to reduce potential inflammatory reactions and donor lung infections that may occur during EVLP. These supplements can help achieve a good perfusion effect.
Metabolic acidosis and electrolyte disturbance were noted in both the human nonacceptable donor lungs and isolated porcine lungs during EVLP. The reason may be that a higher degree of anaerobic respiration occurred in the isolated lungs. The glycolysis rate accelerated, leading to production of a large amount of lactic acid byproduct and a decreased pH value (18). To maintain ion exchange between the inside and outside of the cell membrane, the body needs to take up Na+ and K+ to compensate, eventually causing higher Na+ and K+ levels. Adding a white blood cell removal filter (19) or a cytokine removal filter (20) to the EVLP system has been reported to reduce pulmonary edema, stabilize the pH, and improve electrolyte disturbance.
Prior to the start of EVLP, the GLU levels in human nonacceptable donor lungs and isolated porcine lungs were higher than normal. This phenomenon may be caused by the body’s stress response when the lungs are harvested. According to the literature, GLU level is one of the indicators of lung function, and a higher GLU level in isolated lungs is associated with lower function (21). The results of the present study show that EVLP can bring GLU levels in isolated lungs to normal levels, suggesting that EVLP can improve energy metabolism in isolated lungs and may play a role in the repair of isolated lung function.
In addition to mechanical perfusion techniques, the perfusate solution is also an important aspect of EVLP. To date, the EVLP systems reported worldwide can be divided into three systems: (I) Toronto system; (II) Lund system; and (III) Organ care systemTM (OCSTM) system. The EVLP system used in this study is a modified system based on the Toronto system. Steen solution is used in the Toronto system and the Lund system, while the independently developed OCSTM solution is used in the OCSTM system. Although the formulation of the perfusate solution varies in different systems, to avoid acute lung injury, both the Steen and OCSTM solutions protect endothelial cells by using dextran-40 to maintain osmotic pressure and play antioxidant roles (22). Thus, EVLP itself may not relieve lung injury and edema, but the formulation of the perfusate can be adjusted to repair the donor lung, reduce lung injury, and reduce pulmonary edema. In practical applications, different drugs can be added to the perfusion solution to obtain the corresponding repair and treatment effects. For example, the addition of α1 antitrypsin to the perfusate solution has been reported to improve the degree of apoptosis of donor lung cells and reduce pulmonary edema (23); the addition of vitamin C can improve mitochondrial function and reduce oxidative damage to donor lungs (24); and the addition of antibiotics can reduce infection. These modifications can improve donor lung respiratory function (25).
In summary, EVLP is a good method for preserving donor lungs; to a certain extent, EVLP can maintain the respiratory function of isolated lungs and repair donor lungs. This study demonstrated the feasibility of EVLP for clinical application. The EVLP technique is expected to be used as a replacement and extension of the traditional cold storage method and has become an effective means to improve the rate of qualified donor lungs. Moreover, EVLP has good application prospects in China, where there is a shortage of qualified donor lungs.
Acknowledgments
Funding: This work was supported by Wuxi Health and Family Planning Commission No. ZDRCPY020.
Footnote
Conflicts of Interest: The authors have no conflicts of interest to declare.
Ethical Statement: The authors are accountable for all aspects of the work in ensuring that questions related to the accuracy or integrity of any part of the work are appropriately investigated and resolved. The experiments were approved by the Ethics Committee of the Nanjing Medical University (NJMU-EA-2019-420). All procedures involving animals were approved by the Ethics Committee for Animal Research of Nanjing Medical University.
Open Access Statement: This is an Open Access article distributed in accordance with the Creative Commons Attribution-NonCommercial-NoDerivs 4.0 International License (CC BY-NC-ND 4.0), which permits the non-commercial replication and distribution of the article with the strict proviso that no changes or edits are made and the original work is properly cited (including links to both the formal publication through the relevant DOI and the license). See: https://creativecommons.org/licenses/by-nc-nd/4.0/.
References
- Patel N, Decamp M, Criner GJ. Lung transplantation and lung volume reduction surgery versus transplantation in chronic obstructive pulmonary disease. Proc Am. Thorac Soc 2008;5:447-53. [Crossref] [PubMed]
- Brown AW, Shlobin OA, Weir N, et al. Dynamic patient counseling: a novel concept in idiopathic pulmonary fibrosis. Chest 2012;142:1005-10. [Crossref] [PubMed]
- Mao W, Chen J. Clinical application of the isolated lung perfusion technique. Organ Transplantation (Chinese) 2018;9:334-9.
- Steen S, Sjöberg T, Pierre L, et al. Transplantation of lungs from a non-heart-beating donor. Lancet 2001;357:825-9. [Crossref] [PubMed]
- Steen S, Ingemansson R, Eriksson L, et al. First human transplantation of a nonacceptable donor lung after reconditioning ex vivo. Ann. Thorac. Surg 2007;83:2191-4. [Crossref] [PubMed]
- Cypel M, Yeung JC, Liu M, et al. Normothermic Ex Vivo Lung Perfusion in Clinical Lung Transplantation. N Engl J Med 2011;364:1431-40. [Crossref] [PubMed]
- Cypel M, Yeung JC, Machuca T, et al. Experience with the first 50 ex vivo lung perfusions in clinical transplantation. J Thorac Cardiovasc Surg 2012;144:1200-6. [Crossref] [PubMed]
- Zeriouh M, Sabashnikov A, Mohite PN, et al. Utilization of the organ care system for bilateral lung transplantation: preliminary results of a comparative study. Interact Cardiovasc Thorac Surg 2016;23:351-7. [Crossref] [PubMed]
- Sanchez PG, Davis RD, D’ovidio F, et al. The NOVEL Lung Trial One-Year Outcomes. J Heart Lung Transpl 2014;33:S71-2. [Crossref]
- Pan X, Li J, Pan Y, et al. Comparison of ex vivo lung perfusion with cold storage in rat donor lung preservation. Journal of Shanghai Jiaotong University 2018;38:151-4. (Medical Science) (Chinese).
- Zhu Y. Comparative study on the protective effects of three different ex vivo lung perfusion techniques on isolated dog lungs (Chinese). Kunming Medical University Doctoral Dissertation, 2017.
- Chinese Medical Association Organ Transplantation Society. Technical specifications for the harvest and protection of donor lungs for transplantation in China. Chinese Journal of Transplantation (Chinese) 2019;13:87-90.
- Liu F, Mao W, Chen J, et al. Application of an ex vivo lung repair technique in donor lung repair: report of 2 cases. Chinese Journal of Organ Transplantation (Chinese) 2017;38:51-2.
- Li W, Su Q, Zhao G, et al. Determination of Routine Physiological and Biochemical Parameters in Bama Miniature Porcines under Anaesthesia Condition. Chinese Journal of Veterinary Medicine (Chinese) 2018;54:113-7.
- Machuca TN, Mercier O, Collaud S, et al. Lung transplantation with donation after circulatory determination of death donors and the impact of ex vivo lung perfusion. Am J Transplant 2015;15:993-1002. [Crossref] [PubMed]
- Wallinder A, Riise GC, Ricksten SE, et al. Transplantation after ex vivo lung perfusion: A midterm follow-up. J Heart Lung Transplant 2016;35:1303-10. [Crossref] [PubMed]
- China Lung Transplant Alliance. Summary of 2019 Annual Conference of International Society of Heart and Lung Transplantation. Chinese Journal of Transplantation 2019;13:195-200.
- Koike T, Yeung JC, Cypel M, et al. Kinetics of lactate metabolism during acellular normothermic ex vivo lung perfusion. J Heart Lung Transplant 2011;30:1312-9. [Crossref] [PubMed]
- Nilsson T, Hansson C, Wallinder A, et al. Hemofiltration in ex vivo lung perfusion—a study in experimentally induced pulmonary edema. J Thorac Cardiov Surg 2016;151:570-5.e1. [Crossref] [PubMed]
- Iskender I, Cosgun T, Arni S, et al. Cytokine filtration modulates pulmonary metabolism and edema formation during ex vivo lung perfusion. J Heart Lung Transpl 2018;37:283-91. [Crossref]
- Valenza F, Rosso L, Pizzocri M, et al. The Consumption of Glucose During Ex Vivo Lung Perfusion Correlates With Lung Edema. Transplantation Proceedings 2011;43:993-6. [Crossref] [PubMed]
- Van Raemdonck D, Neyrinck A, Cypel M, et al. Ex-vivo lung perfusion. Transpl Int 2015;28:643-56. [Crossref] [PubMed]
- Lin H, Chen M, Tian F, et al. α1-Anti-trypsin improves function of porcine donor lungs during ex-vivo lung perfusion. J Heart Lung Transplant 2018;37:656-66. [Crossref] [PubMed]
- Shaghaghi H, Kadlecek S, Siddiqui S, et al. Ascorbic acid prolongs the viability and stability of isolated perfused lungs: A mechanistic study using 31P and hyperpolarized 13C nuclear magnetic resonance. Free Radic Biol Med 2015;89:62-71. [Crossref] [PubMed]
- Nakajima D, Cypel M, Bonato R, et al. Ex Vivo Perfusion Treatment of Infection in Human Donor Lungs. J Heart Lung Transpl 2014;33:S28. [Crossref]