The effects of endoplasmic reticulum stress on the expression of exosomes in ventilator-induced lung injury
Introduction
Ventilator-induced lung injury (VILI) is a form of acute lung injury (ALI) associated with mechanical ventilation. It involves pulmonary oedema and hypoxemia, resulting in multi-organ dysfunction and increased mortality.
Endoplasmic reticulum (ER) is an organelle in eukaryocytes that is involved in cell homeostasis, including transport of folding proteins. Only properly folded proteins can be transported from the ER to the Golgi apparatus. Under harmful conditions, the presence of more unfolded proteins leads to ER stress and results in cell apoptosis (1). Extracellular vesicles, including exosomes, also play a role in maintaining cell homeostasis (2). The numbers or profiles of circulating exosomes change in pathophysiologic situations (3-5). ER stress and exosomes are expected to be associated with VILI, but to date this topic has not been investigated.
We hypothesized that ER stress would be associated with exosomes in VILI and that the inhibition of ER stress would be linked to less occurrence of VILI or less damage in VILI. The study was designed to evaluate the association between ER stress and exosomes in VILI in mice. We present the following article in accordance with the ARRIVE Reporting Checklist (available at http://dx.doi.org/10.21037/apm-19-551).
Methods
After approval by the Institutional Animal Care and Use Committee (IACUC) of the Konkuk University (approval number: 9134 & KU9135), all experiments, following the IACUC guidelines, were conducted at the Konkuk University Laboratory Animal Research Center. All experiments were carried out in accordance with the National Institutes of Health (NIH) guidelines for the care and use of laboratory animals. The data used to support the findings of the study are available from the corresponding author upon request.
Preparation of animals
Adult male 12-week-old C57BL/6 mice were purchased from Orient Bio (Seongnam, Korea). The animals were housed in two groups in cages with free access to water and food. The room was maintained with a standard cycle of light and dark every 12 hours (lights on at 7:00 and lights off at 19:00) at a room temperature of 25 °C. All animals were acclimated to the experimental conditions for 7 days before the measurement of VILI. All experiments were performed during the daytime. Animals were anaesthetized by intraperitoneal administration of a mixture of 100 mg/kg ketamine (Ketamine 50, Yuhan, Korea) and 10 m/kg xylazine (Rompun®, Bayer Korea Ltd., Korea). A heating pad was placed on the surgery platform to maintain body temperature during surgery. Mice were secured in the supine position and fastened to the surgery platform with tape. Tracheal intubation was performed on the surgery platform. A 20 G catheter (BD, Franklin Lakes, NJ, USA) was inserted through the larynx to the bronchus. A ventral neck incision was made and the trachea was confirmed. Surgical ties with 5-0 silk around the trachea with the catheter for intubation were tightly installed to prevent air leakage. The correct position of the catheter for intubation was confirmed by checking for symmetrical chest expansion. The right carotid artery was confirmed through the incision, and an A 29 G catheter was placed in the right carotid artery for arterial blood gas analysis (ABGA) and fixed with a 3-0 silk surgical suture. The catheter for intubation was connected to a ventilator (Harvard Apparatus, Holliston, MA USA). The ventilator settings were as follows: (I) fraction of inspired oxygen (FiO2) 1.0; (II) inspired flow rate 0.2 L/min; (III) tidal volume (TV) 8 mL/kg; (IV) inspiration and expiration ratio 1:1; (V) respiration rate 80 breaths/min; and (VI) positive end-expiratory pressure (PEEP) 5 cmH2O. These ventilator settings were maintained for 1 hour. To maintain anaesthesia, isoflurane (Hana Pharm Co. Ltd., Seoul, Korea) 1.5 volume% was inhaled via the catheter for intubation.
After conventional mechanical ventilation for 1 hour, mice were randomly allocated to the control group and Inhibitor group. Normal saline (0.5 mL) was intraperitoneally administered to the control group and 4-phenylbutyric acid (4-PBA; an ER stress inhibitor) (10 mg/kg mixed in 0.5 mL) was administered to the inhibitor group. For allocation, permuted block randomization was carried out by an institutional statistician using a computer-generated list of random numbers and sealed envelopes.
Ventilator settings for VILI
After injection of normal saline for the control group or 4-PBA for the inhibitor group, the ventilator was set as follows: (I) FiO2 1.0; (II) inspired flow rate 0.2 L/min; (III) TV 20 mL/kg; (IV) inspiration and expiration ratio 1:1; (V) respiration rate 80 breaths/min; and (VI) PEEP 5 cmH2O. For anaesthesia maintenance, isoflurane 1.5 volume% was continuously inhaled via the catheter for intubation. Mice were mechanically ventilated with these settings for VILI for 2 hours (6).
Assessment of VILI
Peak inspiration pressure (PIP) and TV were recorded, and dynamic compliance (Cdyn) was calculated every hour after intubation [after intubation (T0), 1 hour after intubation and conventional mechanical ventilation (T1), 1 hour after ventilator settings for VILI (T2), and 2 hours after ventilator settings for VILI (T3)]. Blood (0.2 mL for ABGA) was sampled via the right carotid artery catheter at T0 and at T3.
Preparation of bronchoalveolar lavage (BAL) and lung tissue
After 3 hours of mechanical ventilation, including conventional mechanical ventilation for 1 hour and mechanical ventilation with high TV for VILI for 2 hours, cold phosphate buffered saline (PBS; 1 mL) was flushed into the catheter for intubation with a 1-mL syringe. After waiting 3 seconds, the fluid from BAL was obtained with a syringe. This process was repeated 3 times. The fluid from BAL was transferred in an Eppendorf® tube (1.5 mL) and stored at −80 °C.
After exposure of the thoracic cavity, 4% paraformaldehyde (PFA, Biosesang, Seongnam, Korea) was injected through the left ventricle until the tissues were whitened. The lungs were excised and fixed in 4% paraformaldehyde overnight at 4 °C. The left lung was used to check immunohistochemistry for one of the ER stress markers, protein kinase R-like endoplasmic reticulum (PERK), and Toll-like receptor 4 (TLR4) expression, and the right lung was used to check injury score. They were embedded in paraffin blocks, and the tissues were cut into 5-µm thicknesses using a microtome and then mounted on saline-coated slides.
Exosomes in BAL
After the stored fluid from BAL was melted, it was transferred to an Eppendorf® tube (1.5 ml). It was centrifuged at 400 × g for 10 minutes at 4 °C to remove cell debris. The supernatants were transferred to a new Eppendorf® tube (1.5 mL) and centrifuged at 1,500 ×g for 10 minutes at 4 °C to remove cell debris again. After removal of cell debris, the process for isolation of exosomes was performed. The supernatant was filtered through a 0.2-µm sterile syringe to collect vesicles smaller than 200 nm, and the filtered supernatant was kept on ice. The centrifugal filter units (Amicon®, Merck Millipore, USA) were equilibrated with sterile PBS and centrifuged for 10 minutes at 4 °C to keep them wet until they were used. After equilibration and centrifugation, the PBS was discarded. The filtered supernatant was placed in the devices and centrifuged at 3,000 ×g for 30 minutes at 4 °C. The retentate was washed with sterile PBS by repetitive pipetting and centrifugation at 3,000 ×g for 30 minutes at 4 °C to remove PBS and concentrate exosomes. The concentrated exosomes were collected by pipetting in the devices. The collected exosomes were diluted in PEB staining buffer [PBS + 0.5 mM ethylenediaminetetraacetic acid (UltraPureTM 0.5 M EDTA, Thermo Fisher Scientific, USA) + 0.5% bovine serum albumin (BSA)] (7). They were incubated with blocking solution (10% normal goat serum (ab7481, Abcam, USA) for 10 minutes and then stained with the exosome marker of cluster of differentiation (CD) 63 (PE anti-mouse CD63 Antibody, 143904, USA) in the dark for 30 minutes at room temperature and washed with PEB staining. They were analysed on a BD Accuri C6 flow cytometer (BD Biosciences, USA).
Immunohistochemistry for PERK in the left lung
The sliced left lung tissues were deparaffinised and hydrated. They were immersed in 10% normal horse serum in 1× PBS as blocking solution for 1 hour at room temperature. They were washed in 1× PBS for 5 minutes at three times, and then incubated with PERK antibody (1:200, Bioss Antibodies, Switzerland) in 1× PBS containing 5% normal horse serum for 1 hour at room temperature. Next, they were washed 3 times in 1× PBS for 5 minutes and then incubated using a diluted biotinylated secondary antibody in avidin-biotin-peroxidase complex solution (ABC, Vector Laboratories, USA). After incubation, they were washed in PBS and incubated using avidin-biotin-peroxidase complex solution (ABC, Vector Laboratories, USA) for 1 hour at room temperature. For visualization of PERK staining, they were incubated using DAB solution [DAB (3, 3-diaminobenzidine) peroxidase (HRP) substrate kit (with nickel)] for 3 minutes, according to the technical manual. Slices were stained with hematoxylin as a counterstain, rehydrated through ethanol and xylene, and cover-slipped using mounting medium (Vector Laboratories, USA). Mouse immunoglobulin G (1:200, Biomeda Corporation, USA) was used instead of primary antibody as a negative control. To assess the immunostaining quantification, the optical density of PERK expression was quantified, using NIH ImageJ software.
TLR4 expression in the left lung
The sliced left lung tissues were deparaffinised and hydrated. They were incubated in blocking solution of 10% normal horse serum in 1× PBS for 1 hour and reacted with rabbit TLR4 polyclonal antibodies (1:100, Abcam Inc., USA) overnight at room temperature. After reaction, they were washed 3 times with 1× PBS for 5 minutes and then incubated in horse anti-rabbit secondary antibody with a diluted biotinylated secondary antibody in avidin-biotin-peroxidase complex solution in an ABC kit. After incubation, they were washed and incubated with avidin-biotin-peroxidase complex solution for 1 hour at room temperature. They were incubated with DAB solution and then rehydrated with ethanol and xylene and cover-slipped using mounting medium. Mouse immunoglobulin G (1:200, Biomeda Corporation, USA) was used instead of primary antibody as a negative control. Images were taken using a microscope (Nikon, Tokyo, Japan). The TLR4 intensity was quantified using ImageJ.
Injury score in the right lung
The sliced right lung tissues were deparaffinised and hydrated. They were stained with hematoxylin (Vector Laboratories, USA) and eosin (Sigma-Aldrich, USA) for 3 minutes and then dehydrated and cover-slipped using mounting medium (Vector Laboratories, USA). The tissues were examined, using microscopy, by an investigator who was blind to the study protocol. Lung injury was scored according to the following features: (I) alveolar congestion; (II) haemorrhage; (III) infiltration or aggregation of neutrophils in the air space or the vessel wall; and (IV) thickness of the alveolar wall or hyaline membrane formation. Each item was graded according to the following 5-point scale: 0 for minimal damage, 1 for mild damage, 2 for moderate damage, 3 for severe damage, and 4 for maximal damage. A total score of 0 indicated normal histology and a total score of 16 indicated histology with the most damage (8).
Statistical analyses
The primary outcome was the expression of CD63, the marker for exosomes from BAL. In a pilot study with 3 mice in each group, the expression of CD63 from BAL in the Control and Inhibitor groups was 1.73%±0.16% and 1.21%±0.35%, respectively. The calculated sample size for the primary outcome was 7 in each group used in the pilot study, with an α of 0.05 and power of 0.9.
Analyses were performed using GraphPad Prism 6.0 software (GraphPad Software, La Jolla, USA). Intra-group statistical significance was confirmed by one-way analysis of variance. Inter-group statistical significance between two groups was confirmed by the t-test. All data are expressed as the number of mice and mean ± standard deviation (median, interquartile range). A P value less than 0.05 was considered to indicate statistical significance.
Results
In total, 14 mice were enrolled in the study and evenly allocated into the two groups without any dropout from the study.
PIP after the ventilator settings for VILI was significantly increased and maintained during 2 hours in both groups (Table 1). Similar changes were also observed in TV in both groups (Table 1). In contrast, Cdyn after the ventilator settings for VILI significantly dropped and was maintained at a lower level for 2 hours in both groups (Table 1). The ventilator parameters did not differ significantly between the groups (Table 1).
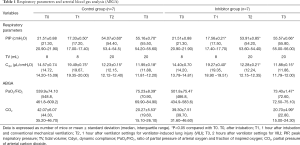
Full table
The ratio of partial pressure of arterial oxygen and fraction of inspired oxygen (PaO2/FiO2), and partial pressure of arterial carbon dioxide (CO2) from ABGA dropped significantly after the ventilator settings for VILI were initiated in both groups, without any significant difference between groups (Table 1).
The expression of CD63, the marker for exosomes from BAL, was significantly lower (P=0.017) in the inhibitor group [0.967%±0.283% (0.870, 0.810–1.227)] compared with the control group [1.559%±0.489% (1.355, 1.259–2.008)] in Control group (Figure 1).

The expressions of immunohistochemistry for PERK and TLR4 from the lung were also significantly lower in the inhibitor group than in the control group: PERK, 0.27±0.05 (0.25, 0.23–0.31) in the inhibitor group vs. 0.75±0.06 (0.77, 0.71–0.80) in the control group (P<0.001); TLR4, 0.30±0.08 (0.32, 0.21–0.34) in the inhibitor group vs. 0.58±0.17 (0.65, 0.39–0.74) in the control group (P=0.002); (Figures 2 and 3).
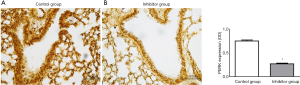
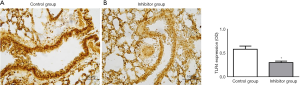
The lung injury score was significantly lower (P=0.001) in the inhibitor group [6.00±1.61 (5.75, 5.00–7.63)] compared with the control group [10.88±1.44 (11.00, 9.50–12.13)] (Figure 4).
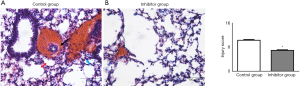
Discussion
Our findings revealed that inhibition of ER stress did not improve ventilator parameters such as PIP, TV, and Cdyn, and ABGA in mice with VILI. However, inhibition of ER stress was associated with less release of exosomes from BAL. It was also associated with less expression of PERK and TLR4 and less damage to the lung.
Dolinay et al. reported that alveolar inflammation was improved after inhibiting the expression of PERK in VILI (9). They concluded that PERK was a mediator for alveolar epithelium injury signals in VILI and that a selective inhibitor for PERK phosphorylation in VILI might be used as a therapeutic intervention (9). Zeng et al. reported that the ER stress inhibitor PBA-4 inhibited ER stress and decreased the release of pro-inflammatory mediators by preventing activation of the nuclear factor kappa light-chain enhancer of activated B cells (NF-κB) pathway in the mouse with lipopolysaccharide-induced ALI (10). Li et al. reported that treatment with PBA-4 significantly improved histopathological findings from the lung, whereas an ER stress inducer, thapsigargin, had the opposite effect in rats with ALI (11). Therefore, PERK was used as an ER stress marker and PBA-4 was used as an ER stress inhibitor in the present study.
Exosomes play a key role in maintaining homeostasis in the lung: exosomes from epithelial cells participate in innate mucosal defence; exosomes from macrophages can modulate inflammatory signals; and exosomes from endothelial cells also participate in the anti-inflammatory process (12). The membrane of exosomes is enriched with endosome-specific tetraspanins (13). One of the tetraspanins, CD63, is expressed in epithelial cells, macrophages, and endothelial cells from the lung (14). Kanemoto et al. demonstrated that a PERK-mediated pathway was involved in the release of exosomes with CD63 in an in vitro study (15). Moreover, CD63 is easily detected by flow cytometry (16). Therefore, CD63 was used as the marker for exosomes in the present study.
Exosomes are known to play key roles in the process of ALI. Yuan et al. reported that the secreted exosomes in BAL had packaged micro-ribonucleic acid (miRNA) and cytokines and regulated inflammation in mice with ALI (17). Moon et al. reported that large amounts of exosomes were generated and released into BAL after lung injury; these exosomes were derived from epithelial cells as the result of hyperoxia-associated ER stress, and they activated a macrophage-mediated inflammatory response via Rho-associated protein kinase 1 (18). Therefore, we might conclude that ER stress was involved with the release of exosomes in mice with VILI.
We found that the inhibition of ER stress produced less damage in the lung, with less release of exosomes from BAL and less expression of PERK and TLR4. Unfortunately, these changes did not improve ventilator parameters and ABGA findings. PaO2/FiO2 is commonly used to evaluate pulmonary function because it is an easily obtained result with reasonably reliable and predictable values, but its accuracy is influenced by several factors (19,20). Several studies have reported better outcomes for pulmonary complications using a specific intervention without any significant improvement of pulmonary oxygenation, and even with lower values (21-23). Moreover, Ferrer et al. demonstrated that adding PaO2/FiO2 ≤240 mmHg to clinical and radiographic criteria did not help diagnosis in ventilator-associated pneumonia (VAP) and PaO2/FiO2 >240 mmHg did not exclude VAP (24). Therefore, the results of the present study might be meaningful, although there was no improvement in pulmonary oxygenation.
In the present study, the expression of ER stress from BAL was not investigated because the specimen from BAL was not sufficient for assessment of both ER stress and exosomes. If both were obtained with confirmation of origins of exosomes, the correlation between ER stress and exosomes from BAL would give more information.
In conclusion, the release of exosomes in mice with VILI was associated with ER stress. Inhibition of ER stress reduced the release of exosomes from the lung with less expression of PERK and TLR4 and improved pulmonary damage in mice with VILI, although it did not improve ventilator parameters or ABGA findings.
Acknowledgments
Funding: This research was supported by Basic Science Research Program through the National Research Foundation of Korea (NRF) funded by the Ministry of Education (NRF-2018R1D1A1B07047066).
Footnote
Reporting Checklist: The authors have completed the ARRIVE Reporting Checklist. Available at http://dx.doi.org/10.21037/apm-19-551
Data Sharing Statement: Available at http://dx.doi.org/10.21037/apm-19-551
Conflicts of Interest: All authors have completed the ICMJE uniform disclosure form (available at http://dx.doi.org/10.21037/apm-19-551). The authors have no conflicts of interest to declare.
Ethical Statement: The authors are accountable for all aspects of the work in ensuring that questions related to the accuracy or integrity of any part of the work are appropriately investigated and resolved. Experiments were performed under a project license (KU9134 & KU9135) granted by Konkuk University Institutional Animal Care and Use Committee, in compliance with the National Institutes of Health (NIH) guidelines for the care and use of laboratory animals.
Open Access Statement: This is an Open Access article distributed in accordance with the Creative Commons Attribution-NonCommercial-NoDerivs 4.0 International License (CC BY-NC-ND 4.0), which permits the non-commercial replication and distribution of the article with the strict proviso that no changes or edits are made and the original work is properly cited (including links to both the formal publication through the relevant DOI and the license). See: https://creativecommons.org/licenses/by-nc-nd/4.0/.
References
- Guzel E, Arlier S, Guzeloglu-Kayisli O, et al. Endoplasmic reticulum stress and homeostasis in reproductive physiology and pathology. Int J Mol Sci 2017;18:792. [Crossref] [PubMed]
- Stahl PD, Raposo G. Extracellular vesicles:exosomes and microvesicles, integrators of homeostasis. Physiology 2019;34:169-77. [Crossref] [PubMed]
- Zhang Y, Liu Y, Liu H, et al. Exosomes:biogenesis, biologic function and clinical potential. Cell Biosci 2019;9:19. [Crossref] [PubMed]
- Shimada Y, Minna JD. Exosome mediated phenotypic changes in lung cancer pathophysiology. Transl Cancer Res 2017;6:S1040-2. [Crossref] [PubMed]
- Jan AT, Rahman S, Khan S, et al. Biology, pathophysiological role, and clinical implications of exosomes: A critical appraisal. Cells 2019;8:99. [Crossref] [PubMed]
- Kneyber MC, Zhang H, Slutsky AS. Ventilator-induced lung injury. Similarity and differences between children and adults. Am J Respir Crit Care Med 2014;190:258-65. [Crossref] [PubMed]
- Parimon T, Garrett NE 3rd, Chen P, et al. Isolation of Extracellular vesicles from murine bronchoalveolar lavage fluid using an ultrafiltration centrifugation technique. J Vis Exp 2018.141. [Crossref] [PubMed]
- Zhang Y, He H, Zhang B, et al. Amelioration of lipopolysaccharide-induced acute lung injury in rats by Na-H exchanger-1 inhibitor amiloride is associated with reversal of ERK mitogen-activated protein kinase. Biomed Res Int 2018;2018:3560234. [Crossref] [PubMed]
- Dolinay T, Aonbangkhen C, Zacharias W, et al. Protein kinase R-like endoplasmatic reticulum kinase is a mediator of stretch in ventilator-induced lung injury. Respir Res 2018;19:157. [Crossref] [PubMed]
- Zeng M, Sang W, Chen S, et al. 4-PBA inhibits LPS-induced inflammation through regulating ER stress and autophagy in acute lung injury models. Toxicol Lett 2017;271:26-37. [Crossref] [PubMed]
- Li PC, Wang BR, Li CC, et al. Seawater inhalation induces acute lung injury via ROS generation and the endoplasmic reticulum stress pathway. Int J Mol Med 2018;41:2505-16. [Crossref] [PubMed]
- Lanyu Z, Feilong H. Emerging role of extracellular vesicles in lung injury and inflammation. Biomed Pharmacother 2019;113:108748. [Crossref] [PubMed]
- Khushman M, Bhardwaj A, Patel GK, et al. Exosomal markers (CD63 and CD9) expression pattern using immunohistochemistry in resected malignant and nonmalignant pancreatic specimens. Pancreas 2017;46:782-8. [Crossref] [PubMed]
- Li ZG, Scott MJ, Brzóska T, et al. lung epithelial cell-derived IL-25 negatively regulates LPS-induced exosome release from macrophages. Mil Med Res 2018;5:24. [Crossref] [PubMed]
- Kanemoto S, Nitani R, Murakami T, et al. Multivesicular body formation enhancement and exosome release during endoplasmic reticulum stress. Biochem Biophys Res Commun 2016;480:166-72. [Crossref] [PubMed]
- Andreu Zoraida, Yáñez-Mó María. Tetraspanins in extracellular vesicle formation and function. Front Immunol 2014;5:442. [Crossref] [PubMed]
- Yuan Z, Bedi B, Sadikot RT. Bronchoalveolar lavage exosomes in lipopolysaccharide-induced septic lung injury. J Vis Exp 2018.57737. [Crossref] [PubMed]
- Moon HG, Cao Y, Yang J, et al. Lung epithelial cell-derived extracellular vesicles activate macrophage-mediated inflammatory responses via ROCK1 pathway. Cell Death Dis 2015;6:e2016. [Crossref] [PubMed]
- Quispe-Laime AM, Bracco JD, Barberio PA, et al. H1N1 influenza a virus-associated acute lung injury:response to combination oseltamivir and prolonged corticosteroid treatment. Intensive Care Med 2010;36:33-41. [Crossref] [PubMed]
- Kuiper JW, Plötz FB, Groeneveld AJ, et al. High tidal volume mechanical ventilation-induced lung injury in rats is greater after acid instillation than after sepsis-induced acute lung injury, but does not increase systemic inflammation:an experimental study. BMC Anesthesiol 2011;11:26. [Crossref] [PubMed]
- Ahn HJ, Kim JA, Yang M, et al. Comparison between conventional and protective one-lung ventilation for ventilator-assisted thoracic surgery. Anaesth Intensive Care 2012;40:780-8. [Crossref] [PubMed]
- Pi X, Cui Y, Wang C, et al. Low tidal volume with PEEP and recruitment expedite the recovery of pulmonary function. Int J Clin Exp Pathol 2015;8:14305-14. [PubMed]
- Su F, Nguyen ND, Creteur J, et al. Use of low tidal volume in septic shock may decrease severity of subsequent acute lung injury. Shock 2004;22:145-50. [Crossref] [PubMed]
- Ferrer M, Sequeira T, Cilloniz C, et al. Ventilator-associated pneumonia and PaO2/FIO2 diagnostic accuracy: changing the paradigm? J Clin Med 2019;8:1217. [Crossref] [PubMed]