Effects of different positive end-expiratory pressure titrating strategies on oxygenation and respiratory mechanics during one-lung ventilation: a randomized controlled trial
Introduction
During one-lung ventilation (OLV) for thoracic surgery, the management of ventilation is still challenging (1). To reduce the risk of atelectasis, hypoxemia, and mismatch of ventilation to perfusion ratio (V/Q), positive end-expiratory pressure (PEEP) is widely used to minimize lung collapse and prevent the alveoli from repeated opening and collapse, and thus improve oxygenation (2,3). However, inappropriate PEEP settings may increase pulmonary vascular resistance and shift blood flow to the non-dependent lung, which can lead to oxygenation impairment during OLV (4). So far, the optimal PEEP level and PEEP titrating strategy during OLV remains to be controversial (1,2,5-8), and processes of maintaining adequate gas exchange and minimizing lung injury may be contradictory at sometimes (9). For seeking the optimal PEEP value, we planned to apply different procedures of PEEP titration, to investigate the effects on oxygenation and respiratory mechanics during OLV.
It has been reported that, when the PEEP value is set to be close to the pressure of lower inflection point (LIP), oxygenation is likely to improve, whereas when the PEEP value increases beyond the pressure of LIP, oxygenation is likely to deteriorate (10). The portion of the pressure-volume (P-V) curve above LIP and below the upper inflection point (UIP) is proposed as the safety zone to avoid cyclic collapse (via setting adequate PEEP) and overdistension (via setting lower VT) (11). However, the intrapulmonary shunt changes during OLV were not considered in previous studies. Furthermore, recent studies have demonstrated that an individualized PEEP adjustment is associated with better oxygenation (2), decreased intrapulmonary shunt (6), lower driving pressure (7), lower or no inflammatory responses (12,13) and lower incidence of postoperative pulmonary complications (14,15). Conversely, an individualized PEEP titration, will not completely prevent atelectasis formation in the particular population (16,17). Thus, we presumed whether there was an optimal pressure between the pressure of LIP and UIP that would keep the optimal amount of alveoli open, and improve oxygenation, intrapulmonary shunt, or respiratory mechanics during OLV.
Therefore, we conducted a randomized controlled trial for OLV in thoracic surgery and compared clinical measurements among PEEP titration groups. Our study aimed to investigate which PEEP titrating method during OLV can improve oxygenation and respiratory mechanics. Thus, we applied different PEEP titrating strategies and measured hemodynamic parameters, respiratory mechanics, intrapulmonary shunt, dead space to tidal volume ratio, and oxygenation index (OI) in patients undergoing thoracic surgery during OLV. We present the following article in accordance with the CONSORT reporting checklist (available at http://dx.doi.org/10.21037/apm-19-441).
Methods
Study design and populations
This study was conducted in accordance with the Declaration of Helsinki (as revised in 2013). This study was approved by the Medical Ethics Committee of Shanghai General Hospital (No. 2016KY109) and registered at the chictr.org.cn website (ChiCTR-OPC-16008292). The study was performed from January 2015 to January 2016 in the Department of Anesthesiology, Shanghai General Hospital. Written informed consent was obtained from all patients before surgery.
Patients scheduled for elective pulmonary resection or esophagectomy under general anesthesia were enrolled if they were aged 18–70 years with American Society of Anesthesiologists (ASA) physical status I or II. The exclusion criteria were body mass index (BMI) <20 or >28 kg/m2, acute pulmonary infection, uncompensated cardiac disease, or a preoperative pulmonary function test of forced expiratory volume in the first second (FEV1) <60% of the predictive value. Drop-out criteria were intraoperative bleeding more than 500 mL, severe hypoxemia, or severe hemodynamic instability during the operation.
Patients were randomly allocated to one of five groups: the “zero PEEP” group (P0, as the control group), the “PEEP set to 2 cmH2O above the pressure of LIP of P-V curve” group (PLIP2), the “PEEP titration according to LIP of P-V curve” group (PLIPS), the “PEEP titration according to static compliance” group (PSTAT), or the “PEEP titration according to dynamic compliance” group (PDYN). The intraoperative mechanical ventilation procedure was performed according to group assignment.
Anesthesia management
Anesthesia management and intraoperative care were standardized. After transferred to the operating room, patients were monitored for electrocardiography, pulse oximetry, and noninvasive blood pressure measurements using an S/5 monitor (Datex-Ohmeda, GE, Finland). For hemodynamic measurements and blood sampling, a 20-G radial artery catheter was introduced under local anesthesia with lidocaine, which was flushed using a pressure bag with 500 mL of heparinized saline dilution. Also, a 14-G right internal jugular vein catheter was placed under local anesthesia with lidocaine. Blood gas values were determined by a blood gas analyzer (ABL 80, Radiometer, Denmark).
After 5 minutes of facemask preoxygenation (100% oxygen), anesthesia was induced with midazolam 0.1 mg/kg, fentanyl 4 µg/kg, propofol 1.5–2 mg/kg, and rocuronium 0.6 mg/kg. After the patients were anesthetized, tracheal intubation was conducted with an appropriate double-lumen tube (ShileyTM Endobronchial Suction Catheters with Color Coded Connectors, Covidien, Ireland). The tube position was confirmed by visual bronchoscopy in the supine and lateral positions. Sevoflurane inhalation was administered to maintain the minimal alveolar concentration (MAC) between 1.0 and 1.2. Additional fentanyl (50 µg each time) and rocuronium (10 mg each time) were administered as necessary.
Ventilation settings
Pulmonary ventilation in all patients was supported by the anesthesia machine (WATO EX-55, Mindray, China) using volume-controlled ventilation mode. The inspiratory-to-expiratory ratio was 1:1.5, and the frequency was adjusted to maintain end-tidal carbon dioxide partial pressure (PetCO2) between 35 and 50 mmHg. During two-lung ventilation (TLV), all patients of five groups were ventilated with the same protocol (VT: 8 mL/kg, FiO2: 60%, and PEEP: 0). During OLV, VT was set to 6 mL/kg, FiO2 was set as 100% to avoid hypoxemia and exclude interference in the measurement of PaO2, and PEEP varied according to the experimental protocol (see PEEP titrating strategies). After OLV switched to TLV and before extubation, the bilateral lung of all patients was treated with sustained manual expansion of the reservoir bag (30 cmH2O for 10 seconds) three times.
PEEP titrating strategies
In the five groups, the same procedures were applied except for the PEEP titration of the OLV period (Figure 1). During the TLV period, the P-V curve was measured in the lateral position by the GE E-sCAiOVX respiratory module and determined three times, and then the mean value of the LIP pressure of the P-V curve was recorded. In PLIP2 group, the PEEP value during OLV was set to 2 cmH2O above the pressure of LIP; in PLIPS group, taking the averaged value of the LIP pressure as fundamental titration value, we gradually increased PEEP value during OLV in 1 cmH2O steps until static compliance was achieved to the maximum, while PEEP was held at each step for 5 minutes; in group PSTAT, the incremental PEEP value during OLV was titrated to achieve maximum static compliance in 1 cmH2O steps and held at each step for 5 minutes; in group PDYN, the incremental PEEP value during OLV was titrated to achieve maximum dynamic compliance from 4 cmH2O in 1 cmH2O steps and held at each step for 5 minutes; in P0 group, zero PEEP level was set as control.
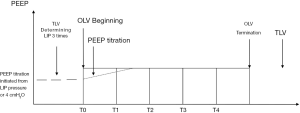
Measurements
The heart rate (HR), mean arterial pressure (MAP), pulse oxygen saturation (SpO2), PetCO2, VT, respiratory rate (RR), peak inspiratory pressure (PIP), plateau inspiratory pressure (Pplat), and I: E were monitored continuously with an S/5 monitor (Datex-Ohmeda, Finland). The gas sampling tube (sidestream technique) was connected to the Y-junction of the respiratory circuit. Each measurement was obtained and recorded immediately at the beginning of OLV (T0), OLV 15 min (T1), OLV 30 min (T2), OLV 45 min (T3), and OLV 60 min (T4). Arterial and venous blood samplings were performed for blood gas analyses at T0, T1, T2, T3, and T4, then arterial carbon dioxide partial pressure (PaCO2), arterial oxygen partial pressure (PaO2), arterial oxygen saturation (SaO2), and venous oxygen saturation (SvO2) were recorded.
Driving pressure was calculated using the equation (18):
ΔP = Pplat – PEEP [1]
Dynamic compliance of the respiratory system was calculated with the standard formula:
C = VT/(PIP – PEEP) [2]
The alveolar dead space/tidal volume ratio (VD/VT) was calculated using the equation (19):
VD/VT = 1.14 × (PaCO2 – PetCO2)/PaCO2 – 0.005 [3]
The intrapulmonary shunt (Qs/Qt) was calculated with the formula (see Supplement I).
Sample size and randomization
Based on our pilot study, it was estimated that a total of 75 patients were needed to detect a difference of at least a 20% difference in PaO2 between any study group and the control group at the time of OLV 30 min, with a 5% significance level, 80% power. Sample size analysis was performed using PASS software (version 11.0.7, NCSS, USA).
Randomization was done by computer-generated random numbers (randomization lists). The patients were randomly assigned with a 1:1:1:1:1 ratio to one of the five groups: PLIP2, PLIPS, PSTAT, PDYN, or P0 group. The randomization schedule was generated by NCSS PASS software (see Supplement II) and concealed from the investigators. The administrator prepared sequentially numbered envelopes that were sealed and opaque to maintain allocation concealment until the time of randomization. The investigators enrolled the study subjects after evaluating eligibility and assigned patients to different groups by opening the randomization envelopes just before the beginning of anesthesia.
Statistical analysis
Statistical analysis was performed using SPSS Statistics for Windows (version 20.0, IBM, USA). Continuous data were evaluated with the Kolmogorov-Smirnov test. Normally distributed continuous data were expressed as means ± standard deviation. Nonnormally distributed continuous variables were presented using medians (range). Differences between measurements at each time point using different PEEP titrating strategies were analyzed with multivariate ANOVA (General Linear Model), while post hoc analyses were performed using Bonferroni’s correction. Categorical data were presented as frequencies and analyzed with a χ2 test. We considered a value of P<0.05 to be statistically significant.
Results
Eighty-two patients were assessed for eligibility in this study. Seventy-five patients consented to participate in this study and were randomly assigned to groups (Table S1). Finally, 15 patients in each group were analyzed (Figure 2). None of the patients demonstrated protocol-related complications or adverse events, such as intraoperative bleeding more than 500 mL, severe hypoxemia during OLV, or severe hemodynamic instability during the operation. None of the patients was affected by severe postoperative pulmonary complications. There were no significant differences in patients’ characteristics and clinical data among the five groups (Table 1).
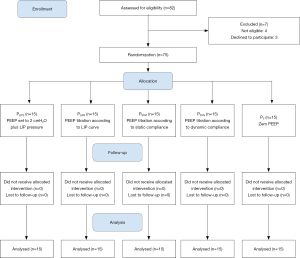
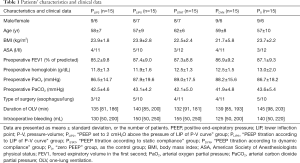
Full table
Comparisons of hemodynamic measurements are presented in Table 2. During OLV, MAP decreased at every time point when compared with the baseline value. In PLIP2 and PSTAT group, there were significant differences at T1 and T2 when compared with T0. In the PLIPS group, MAP decreased significantly at T1, T2, T3, and T4 when compared with T0.
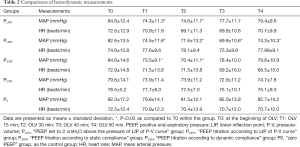
Full table
Comparisons of respiratory mechanics are presented in Table 3. PIP and Pplat significantly increased after PEEP titration when compared with the basal levels at T0 (P<0.05). PIP showed no differences among groups despite the different PEEP strategies. In groups PLIP2, PLIPS, and PSTAT, Pplat significantly increased after PEEP titration when compared with group P0 (P<0.05). Pplat in group PDYN showed no differences compared to P0 (P>0.05). In each PEEP titration group, the PEEP values at T4 were close (PLIP2, 7.7±0.8; PLIPS, 8.1±0.7; PSTAT, 7.5±1.2; PDYN, 7.7±0.7; P>0.05).
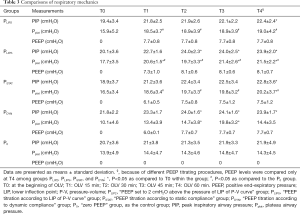
Full table
Comparisons of driving pressure are presented in Figure 3. Driving pressure significantly decreased after PEEP titration when compared with the basal levels at T0 (P<0.05). In group PDYN, driving pressure significantly decreased after PEEP titration when compared with group P0 (P<0.05).
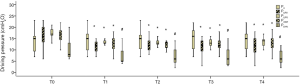
Comparisons of dynamic compliance are presented in Figure 4. Dynamic compliance significantly increased after PEEP titration when compared with the basal levels at T0 (P<0.05). In group PLIP2, PSTAT, and PDYN, dynamic compliance significantly increased after PEEP titration when compared with group P0 (P<0.05).
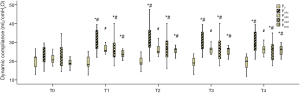
Comparisons of VD/VT are presented in Figure 5. VD/VT significantly increased in group PLIP2 at T1 and T2, and in group PLIPS at T3 and T4 when compared with T0 (P<0.05). However, VD/VT did not change significantly in group PDYN.
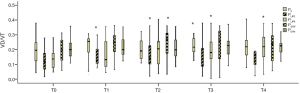
Comparisons of Qs/Qt and OI are presented in Figure 6 and Figure 7. After PEEP titration, there was a slight decrease in Qs/Qt during OLV. However, there were no significant changes at T1, T2, T3, and T4 when compared with T0 in any group. There were no significant changes in groups PLIP2, PLIPS, PSTAT, and PDYN when compared with group P0.
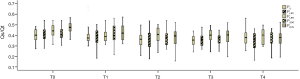
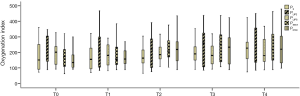
Discussion
Our study demonstrated that dynamic compliance, VD/VT, PIP, and Pplat significantly increased, and driving pressure significantly decreased after PEEP titration when compared with the basal levels, whereas the intrapulmonary shunt and OI showed no significant changes. PIP, Qs/Qt, and OI showed no differences among groups despite the different PEEP titrating strategies. The optimal PEEP values were close in each PEEP titration group. When PEEP titrated according to dynamic compliance, respiratory mechanics showed lower Pplat, lower driving pressure, and higher dynamic compliance, while the intrapulmonary shunt, dead space ratio, and OI did not change significantly.
A low tidal volume ventilation strategy combined with PEEP, as an essential component of a protective lung ventilation protocol, can prevent ventilator-induced lung injury (VILI). The mechanism of PEEP involves maintaining alveoli open at the end of expiration, improving functional residual capacity, lung compliance, and oxygenation, and decreasing intrapulmonary shunt (2,3). In clinical studies, PEEP is most commonly set near to the pressure of LIP of the P-V curve to prevent end-expiratory collapse (10). Although this seems to be physiological, its clinical application value is still unclear and controversial. First of all, the pressure of LIP is hard to be defined on the P-V curve, especially in the existence of extensive lung lesions. Secondly, the gravity gradient of pleural pressure in patients implies that there will not be a single pressure open up collapsed regions of the recruitable lung. Lastly, the approach of P-V curve analysis may be affected by observers’ variability, which makes it difficult to compare results from different studies. Therefore, there is no standard method for PEEP titration individualized according to LIP. For group PLIP2, we intended to decide a point of P-V curve between LIP and UIP according to our clinical experience and previous studies (11). For group PLIPS, we gradually increased PEEP value from the basal LIP pressure until static compliance achieved maximum value. PEEP titration according to LIP (group PLIP2 and PLIPS), showed no significant changes in the intrapulmonary shunt and not improved oxygenation. However, the dead space ratio significantly increased when compared with the basal level. We considered the reason to be that the airway pressure increased suddenly and was not maintained at a sufficient degree to open adequate alveoli.
It is reported that the optimal PEEP should be the pressure value of optimal lung compliance (20). Thus, our study also involved another two PEEP titration groups (PSTAT and PDYN), in which PEEP was titrated according to static or dynamic compliance. Dynamic lung compliance was affected by the elasticity of lung tissue, airway resistance, and the change in internal chest pressure, while static lung compliance was affected by the elasticity of the lung tissue alone. We assumed that the PEEP titrating strategy according to dynamic lung compliance might regulate whole chest homeostasis, while the PEEP titrating strategy according to static lung compliance only regulated the lung volume. We also considered that PEEP titration procedures made the airway resistance gradually increase, which wound not markedly decrease the venous return. Our study demonstrated that respiratory mechanics showed lower Pplat, lower driving pressure, and higher dynamic compliance in group PDYN. Our results showed that although PEEP titrating strategy according to dynamic compliance can improve respiratory mechanics, it does not adequately improve arterial oxygenation, which seemed to be inconsistent with other studies (2-4,6). Besides this, we found no significant effects on dead space ratio and intrapulmonary shunt. In the literature, the intrapulmonary shunt is an essential reason for hypoxemia in OLV during thoracic surgical operations (6). Particularly in the lateral position, it is the result of gravity, as well as the mismatching of ventilation and blood flow. The existence of dead space is associated with the intrapulmonary shunt. Besides, the alveolar recruitment maneuver during OLV may play a vital role in the improvements of oxygenation and lung mechanics (21-24).
In our study, MAP and HR showed statistically significant differences at some time points, but this has no clinical meaning because of all changes within the physiological range. Considering the changes related to surgical operations, the use of anesthetic drugs, anesthesia depth changes, individual differences, and other factors, we generally thought PEEP titrating strategies had no significant effects on hemodynamic measurements (25). We also found that PIP and Pplat values significantly increased after PEEP titration. One report suggested that PEEP would increase airway pressure and decrease airway resistance, and would not result in increased inflammatory mediators (13). Thus, we consider that increased airway pressure would not injure the lung tissues. Furthermore, driving pressure (defined as tidal volume divided by respiratory system compliance), significantly decreased after PEEP titration. Therefore, ventilation at the lowest driving pressure and the highest respiratory system compliance has been recommended for thoracic surgery (14). During OLV, driving pressure can be reduced after individualized PEEP titration, which may be related to a reduced incidence of postoperative pulmonary complications.
Study limitations
Our study has some limitations. First, our patients ranged from ASA I to II, whose lung function had not deteriorated severely. Further study is still needed to determine whether the PEEP titrating strategies according to dynamic lung compliance valuable to severe patients. Second, FiO2 was set to 100% during OLV and 60% during TLV. It is appropriate to keep the FiO2 at the lowest possible level and increase if necessary. The incidence of postoperative pulmonary complications was not recorded. Last, in our study, the intrapulmonary shunt was estimated and calculated from several equations, which may be less accurate than direct measurements (6).
Conclusions
The PEEP titrating strategy according to dynamic compliance can improve respiratory mechanics, whereas it has no significant effects on oxygenation, dead space ratio, and intrapulmonary shunt, suggesting that it is better during OLV for thoracic surgery.
Acknowledgments
The authors thank Department of Anesthesiology, Shanghai General Hospital.
Funding: This work was supported by the Key Discipline and Specialty Foundation in Anesthesiology of Shanghai General Hospital (No. 040526).
Footnote
Reporting Checklist: The authors have completed the CONSORT reporting checklist. Available at http://dx.doi.org/10.21037/apm-19-441
Data Sharing Statement: Available at http://dx.doi.org/10.21037/apm-19-441
Conflicts of Interest: All authors have completed the ICMJE uniform disclosure form (available at http://dx.doi.org/10.21037/apm-19-441). The authors have no conflicts of interest to declare.
Ethical Statement: The authors are accountable for all aspects of the work in ensuring that questions related to the accuracy or integrity of any part of the work are appropriately investigated and resolved. This study was conducted in accordance with the Declaration of Helsinki (as revised in 2013). This study was approved by the Medical Ethics Committee of Shanghai General Hospital (No. 2016KY109) and registered at the chictr.org.cn website (ChiCTR-OPC-16008292). All patients were provided written informed consent before participation.
Open Access Statement: This is an Open Access article distributed in accordance with the Creative Commons Attribution-NonCommercial-NoDerivs 4.0 International License (CC BY-NC-ND 4.0), which permits the non-commercial replication and distribution of the article with the strict proviso that no changes or edits are made and the original work is properly cited (including links to both the formal publication through the relevant DOI and the license). See: https://creativecommons.org/licenses/by-nc-nd/4.0/.
References
- Karzai W, Schwarzkopf K. Hypoxemia during one-lung ventilation: Prediction, prevention, and treatment. Anesthesiology 2009;110:1402-11. [Crossref] [PubMed]
- Licker M, Diaper J, Villiger Y, et al. Impact of intraoperative lung-protective interventions in patients undergoing lung cancer surgery. Crit Care 2009;13:R41. [Crossref] [PubMed]
- Michelet P, Roch A, Brousse D, et al. Effects of PEEP on oxygenation and respiratory mechanics during one-lung ventilation. Br J Anaesth 2005;95:267-73. [Crossref] [PubMed]
- Rozé H, Lafargue M, Perez P, et al. Reducing tidal volume and increasing positive end-expiratory pressure with constant plateau pressure during one-lung ventilation: effect on oxygenation. Br J Anaesth 2012;108:1022-7. [Crossref] [PubMed]
- Della Rocca G, Coccia C. Ventilatory management of one-lung ventilation. Minerva Anestesiol 2011;77:534-6. [PubMed]
- Spadaro S, Grasso S, Karbing DS, et al. Physiologic evaluation of ventilation perfusion mismatch and respiratory mechanics at different positive end-expiratory pressure in patients undergoing protective one-lung ventilation. Anesthesiology 2018;128:531-8. [Crossref] [PubMed]
- Belda J, Ferrando C, Garutti I, et al. The effects of an open-lung approach during one-lung ventilation on postoperative pulmonary complications and driving pressure: a descriptive multicenter national study. J Cardiothorac Vasc Anesth 2018;32:2665-72. [Crossref] [PubMed]
- Shi ZG, Geng WM, Gao GK, et al. Application of alveolar recruitment strategy and positive end-expiratory pressure combined with autoflow in the one lung ventilation during thoracic surgery in obese patients. J Thorac Dis 2019;11:488-94. [Crossref] [PubMed]
- Bernasconi F, Piccioni F. One-lung ventilation for thoracic surgery: current perspectives. Tumori 2017;103:495-503. [Crossref] [PubMed]
- Slinger PD, Kruger M, McRae K, et al. Relation of the static compliance curve and positive end-expiratory pressure to oxygenation during one-lung ventilation. Anesthesiology 2001;95:1096-102. [Crossref] [PubMed]
- Blanch L, López-Aguilar J, Villagra A. Bedside evaluation of pressure-volume curves in patients with acute respiratory distress syndrome. Curr Opin Crit Care 2007;13:332-7. [Crossref] [PubMed]
- Kim HJ, Seo JH, Park KU, et al. Effect of combining a recruitment maneuver with protective ventilation on inflammatory responses in video-assisted thoracoscopic lobectomy: a randomized controlled trial. Surg Endosc 2019;33:1403-11. [Crossref] [PubMed]
- Fiorelli S, Defraia V, Cipolla F, et al. Short-term one-lung ventilation does not influence local inflammatory cytokine response after lung resection. J Thorac Dis 2018;10:1864-74. [Crossref] [PubMed]
- Park M, Ahn HJ, Kim JA, et al. Driving pressure during thoracic surgery: a randomized clinical trial. Anesthesiology 2019;130:385-93. [Crossref] [PubMed]
- Okahara S, Shimizu K, Suzuki S, et al. Associations between intraoperative ventilator settings during one-lung ventilation and postoperative pulmonary complications: a prospective observational study. BMC Anesthesiol 2018;18:13. [Crossref] [PubMed]
- Young CC, Harris EM, Vacchiano C, et al. Lung-protective ventilation for the surgical patient: international expert panel-based consensus recommendations. Br J Anaesth 2019;123:898-913. [Crossref] [PubMed]
- Kiss T, Wittenstein J, Becker C, et al. Protective ventilation with high versus low positive end-expiratory pressure during one-lung ventilation for thoracic surgery (PROTHOR): study protocol for a randomized controlled trial. Trials 2019;20:213. [Crossref] [PubMed]
- Amato MB, Meade MO, Slutsky AS, et al. Driving pressure and survival in the acute respiratory distress syndrome. N Engl J Med 2015;372:747-55. [Crossref] [PubMed]
- Hardman JG, Aitkenhead AR. Estimating alveolar dead space from the arterial to end-tidal CO2 gradient: a modeling analysis. Anesth Analg 2003;97:1846-51. [Crossref] [PubMed]
- Carvalho AR, Jandre FC, Pino AV, et al. Positive end-expiratory pressure at minimal respiratory elastance represents the best compromise between mechanical stress and lung aeration in oleic acid induced lung injury. Crit Care 2007;11:R86. [Crossref] [PubMed]
- Ferrando C, Mugarra A, Gutierrez A, et al. Setting individualized positive end-expiratory pressure level with a positive end-expiratory pressure decrement trial after a recruitment maneuver improves oxygenation and lung mechanics during one-lung ventilation. Anesth Analg 2014;118:657-65. [Crossref] [PubMed]
- Unzueta C, Tusman G, Suarez-Sipmann F, et al. Alveolar recruitment improves ventilation during thoracic surgery: a randomized controlled trial. Br J Anaesth 2012;108:517-24. [Crossref] [PubMed]
- Park SH, Jeon YT, Hwang JW, et al. A preemptive alveolar recruitment strategy before one-lung ventilation improves arterial oxygenation in patients undergoing thoracic surgery: a prospective randomised study. Eur J Anaesthesiol 2011;28:298-302. [Crossref] [PubMed]
- Blank RS, Colquhoun DA, Durieux ME, et al. Management of one-lung ventilation: Impact of tidal volume on complications after thoracic surgery. Anesthesiology 2016;124:1286-95. [Crossref] [PubMed]
- Fernández-Pérez ER, Keegan MT, Brown DR, et al. Intraoperative tidal volume as a risk factor for respiratory failure after pneumonectomy. Anesthesiology 2006;105:14-8. [Crossref] [PubMed]