Vitamin C attenuates vancomycin induced nephrotoxicity through the reduction of oxidative stress and inflammation in HK-2 cells
Introduction
Vancomycin (VCM) is a very effective antibiotic for severe infections caused by Gram-positive bacteria. Clinical guidelines recommend that the trough concentration of VCM in adults with ordinary infections should be maintained at 10–15, and 15–20 mg/L would be needed in severe or complex infections to ensure its antibacterial effect (1,2). However, with rise in the trough concentration, risk of VCM related nephrotoxicity increased significantly. The incidence of nephrotoxicity was 29.6% and 8.9%, respectively, when trough concentrations of VCM were >15 and ≤15 mg/L (3). Patients receiving more than 4 g/day of VCM were 35% likely to occur renal injury according to the research by Lodise et al. (4). In vitro studies revealed that when concentration of VCM in the renal proximal tubule increases, reactive oxygen species (ROS) generates in the epithelial cells and activates the oxidative stress response, which leads to DNA damage, cell apoptosis, mitochondrial disorder and cell necrosis (5,6). Use of antioxidants significantly inhibited VCM induced oxidative stress and reduced the incidence of renal toxicity (7,8). However, there are currently few drugs clinically available to relieve nephrotoxicity in severe ill patients receiving high dose VCM.
Vitamin C (VC) is an important and classic antioxidant. Intravenous supplement of VC in large doses (100–200 mg/kg/day) has proven to be particularly effective in treatments of tumors and critical illnesses associated with oxidative stress (9,10) by rapidly improving the body’s antioxidant capacity. Several studies (11,12) have found that in addition to eliminating ROS directly, high dose VC can ameliorate organ damage in hemorrhagic shock rats by inducing heme oxygenase HO-1 and deacetylase SIRT1, which indicated a possible mechanism of high dose VC. An analysis of 188 patients using VCM found that 2 g/day VC oral intake reduced the incidence of acute kidney injury (AKI) (13). A very recent study by Takigawa et al. (14) showed that 4 g/kg/day VC injection reduced VCM induced nephrotoxicity in mice by decreasing apoptosis of renal cell. However, overdose of VC intake may lead to oxalate nephropathy and aggravation of renal injury (15,16). Besides, the mechanisms of VC in attenuating oxidative stress, cell apoptosis or inflammatory response were not mentioned in these articles, the underlying molecular mechanism remains to be elucidated. Thus, the present study investigated the protective role of high dose VC against oxidative stress and inflammation induced by VCM in HK-2 renal tubular epithelial cells.
Methods
Materials
VCM (CAS: 1404-90-6) was purchased from Selleck (Shanghai, China). VC was purchased from Sangon Biotech (Shanghai, China). DME/F-12 was purchased from Hyclone (Logan, USA). Fetal bovine serum, penicillin and streptomycin were purchased from Gibco (NY, USA).
Cell culture
The immortalized proximal tubule epithelial cell line from normal adult human kidney (HK-2, RRID: CVCL_0302, ATCC: CRL-2190) was gifted from Nephrology Department of our hospital. Cells were grown in medium DME/F-12 supplemented with 10% fetal bovine serum, 100 µg/mL penicillin and 100 µg/mL streptomycin in a humidified atmosphere of 5% CO2 at 37 °C. Cells were seeded in cell culture plates and used for experiments at 70–80% confluence.
Measurement of cell viability
Cell viability of HK-2 cells under VC were tested first, results showed that cell proliferation inhibition began to occur when VC ≥2 mM. Previously sub-cultured cells (104 cells/mL) were exposed to various concentrations of VCM in 0.1% DMSO and VC (4, 6, 8 mM of VCM and 0.5, 1 mM of VC), and 0.1% DMSO only as control group in a 96-well plate and incubated them for 24 h at 37 °C in 5% CO2. Then the supernatants were removed, 10 µL CCK8 (APExBIO Technology, Houston, USA) was added to each well and the cells were incubated for another 4 h. We measured the optical density at 450 nm as the reference (Thermo, USA).
Measurement of MDA level and superoxide dismutase 2 (SOD2) activity
We exposed previously sub-cultured cells (105 cells/mL) to 4 mM VCM and 0.5 mM VC in a 24-well plate and incubated them for 24 h at 37 °C in 5% CO2. Cells were lysed and the supernatants obtained from 3,000 rpm at 4 °C for 10 min centrifugation were used to measure SOD2 activity (Beyotime Biotechnology, Shanghai, China) and malondialdehyde (MDA) level (Nanjing Jiancheng Bioengineering, Nanjing, China) using commercial assay kits according to the manufacturer’s instructions.
Real-time PCR
Cells were harvested for RNA extraction using TRIzol. The reverse transcription reaction was conducted using RevertAid First Strand cDNA Synthesis Kit (Thermo Scientific, California, USA) to generate the single-strand cDNA. Quantitative real-time PCR was performed on the ABI 7300 Real-Time PCR System (Thermo Scientific, California, USA) using the FastStart Universal SYBR Green Master (Roche, Mannheim, Germany). The relative mRNA expression levels were normalized to GAPDH and calculated using the ΔΔCt method. The mRNA levels were expressed relative to the control group. The sequences of the upstream and downstream primers for nuclear factor-κB (NF-κB), tumor necrosis factor-α (TNF-α), interleukin-6 (IL-6) and GAPDH are shown in Table 1.
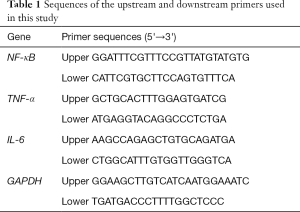
Full table
Western-blot analysis
Cells were lysed and the supernatants obtained from 12,000 rpm at 4 °C for 10 min centrifugation were loaded onto a 12% resolving gel for electrophoresis. The proteins were transblotted onto a 0.45 µm PVDF membrane (Millipore, CA, USA). The membranes were blocked by incubation in phosphate-buffered saline containing 0.1%. Tween 20 and 5% non-fat milk for 1 h at room temperature. The blot was immuneprobed with IL-6 antibody (1:750 dilution, RRID: AB_10732306, Servicebio, Wuhan, China) and GAPDH antibody (1:45,000 dilution, RRID: AB_1616725, Proteintech Group, Rosemont, USA) overnight at 4 °C. The blots were then incubated with an HRP-conjugated secondary antibody (Servicebio, Wuhan, China) for 1 hr at room temperature and then reacted with an enhanced chemiluminescence substrate (Servicebio, Wuhan, China). The resulting chemiluminescence was recorded with an imaging system (EPSON V300, Shanghai, China). To quantify the protein expression, the enhanced chemiluminescence signals were digitized using the Photoshop CS6 software (Adobe, USA).
Statistical analysis
Numerical variables are summarized as mean ± standard deviation (SD). Data were analyzed using SPSS statistical software (version 11.0, SPSS Inc., Chicago). Determination of the statistical differences in parameters between groups was performed by either Student’s t-test or the Mann-Whitney U test. A P value <0.05 was considered to be statistically significant.
Results
VC reduced VCM induced cell injury in HK-2
CCK8 assay was performed to determine the protection effect of VC on cell death induced by VCM of HK-2 cells. VCM inhibited the cell viability of HK-2 cells to 34–66% in a dose-dependent manner, and VC reduced the cell toxicity of VCM, as shown in Figure 1A. Cell viability in all VC co-exposure groups were significantly increased from the relative VCM groups (P<0.05), 0.5 and 1 mM VC increased cell viability of 6 mM VCM by 30% and 37%, respectively. The viability of cells between groups of DMSO and 4 mM VCM combined 0.5 mM VC showed no difference (P>0.05), so we chose 4 mM VCM and 0.5 mM VC for the following tests.
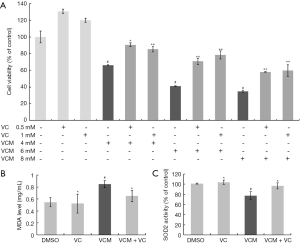
VC reversed VCM induced oxidative stress in HK-2 cells
MDA is one of the major secondary products of lipid peroxidation, and also a reflection of ROS-induced cell damage. The antioxidant activity of SOD2 was reduced and the MDA level was increased in the 4 mM VCM group (P<0.05 compared to DMSO group), which revealed significant induction of VCM on oxidative stress. Compared with the 4 mM VCM only group, SOD2 activity was significantly increased by 25% and MDA level was significantly reduced by 23% (P<0.05) in the 0.5 mM VC combined group. MDA level and SOD2 activity showed no difference between the DMSO, VC and VCM+VC group (Figure 1B,C).
VC attenuated protein expression of NF-κB and pro-inflammatory cytokines in HK-2 cells activated by VCM
VCM treatment on HK-2 cells resulted in significant increases in NF-κB mRNA level, a threefold increase in pro-inflammatory cytokines TNF-α, and a 13.9-fold in IL-6 (P<0.05 compared to DMSO group). With 0.5 mM VC combined, the mRNA levels of NF-κB and TNF-α greatly reduced to no difference from control, and IL-6 level significant decreased compared to the VCM group (P<0.05). There were no obvious changes in NF-κB, TNF-α and IL-6 mRNA levels in the VC only group compared with control (Figure 2A,B,C). According to the western-blot test of IL-6, 0.5 mM VC significantly attenuated the protein expression activated by 4 mM VCM (Figure 2D). These results verified the inhibitory effect of VC on pro-inflammatory cytokines activation induced by VCM.
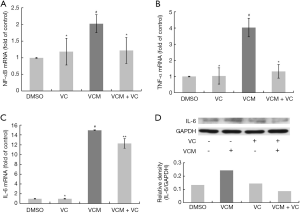
Discussion
VCM is considered as the first-line treatment against infections with Gram-positive bacteria (17), but its use is mostly limited by the nephrotoxicity. Animal experiments showed that VCM leads to pathological changes in kidney tissue in connection with oxidative stress (18,19). The antioxidant effects of high doses (100–200 mg/kg/day) of VC were effectively confirmed in tumor, severe diseases treatment and organ injuries in vivo (10,11). Overdose VC (4 g/kg) pretreatment had protective effects on VCM induced pathological changes in kidney tissue of mice by reducing renal cell apoptosis (14). However, the underlying mechanism is not clear. In the present study, we demonstrated that VC treatment led to a marked improvement in oxidative stress and inflammatory response in HK-2 cells, and the protective effect of VC might relate to its inhibition of ROS and NF-κB.
HK-2 cells incubated at 4, 6 and 8 mM VCM for 24 hrs appeared a dose‐dependent decrease in cell viability from 66% to 34%. Co-treatment with 0.5 and 1 mM VC attenuated VCM induced cell death and increased the cell viability to 58–90%. 4 mM VCM exposure also resulted in a significant increase in oxidative stress indicator MDA and reduction in SOD2 according to our results, and was significantly reversed by 0.5 mM VC. These results indicated that VC attenuated VCM induced oxidative stress and prevented cell death.
The non-homeostatic states “oxidative stress” is caused by the over production of ROS (20). Superoxide dismutase SOD2 can effectively convert superoxide (one type of ROS) to less active hydrogen peroxide, the loss of SOD2 activity will lead to some metabolic diseases (21). ROS generated in cell can damage cellular macromolecules, including nucleic acids, phospholipids and proteins. ROS stimulation of mitochondrial lipid peroxidation may lead to inhibition of mitochondrial metabolism and mitochondrial dysfunction, such as abnormal in mitochondrial membrane potential and Ca2+ buffering capacity which plays a key role in certain modes of cell death (20,22). Overexpressed of lipid peroxidation product MDA and downregulation of SOD2 were found in many studies on VCM induced nephrotoxicity (18,23), and also in our results. Meanwhile, we found for the first time that the VC combination reduced cell death and MDA levels, and increased SOD2 activity in HK-2 cells under VCM treatment. This effect may be related to the antioxidant activity of VC.
Interstitial inflammations were found in histopathological examinations of kidney tissues from animals treated with VCM (19,24). Studies suggested that increased ROS, membrane lipid peroxidation and production of inflammatory chemokines may be the pathogenesis of VCM nephrotoxicity (25). According to the researches we found, NF-κB activated by ROS, the transcription factor that has pivotal roles in the immune response, inflammation, cell proliferation and apoptosis (26), may be involved in the inflammatory chemokines overexpression and also cell death. Phosphorylation and deactivation on inhibitors of NF‐κB (IκB) caused by ROS stimulation of tyrosine protein kinase (27), and stimulation of NF‐κB modulators IKKα and IKKβ through TNF-α induction (28) were considered as possible mechanisms for ROS acted as a messenger in NF-κB activation. The increased expression of NF-κB activates IL-6 promoter (29) and other pro-inflammatory cytokines including IL-1β and TNF-α, to promote inflammatory cascade and relative cell death (30). NF-κB signaling pathway has now become one of the potential targets for tumors associated with chronic inflammation (29,31). Inflammation response and upregulation of inflammatory cytokines were widely discovered in the researches on VCM induced nephrotoxicity (6,19,32). In our results, 4 mM VCM markedly induced the mRNA expression of NF-κB, IL-6, TNF-α and protein expression of IL-6 in HK-2 cells. The addition of VC reversed this effect with the inhibition of ROS. These findings suggested that VC can inhibit the inflammatory cascade associated with NF-κB through the reduced of oxidative stress.
In conclusion, we confirmed that VCM caused cell death by inducing oxidative stress and inflammatory response in HK-2 cells. VC attenuated the nephrotoxicity through decreasing lipid peroxidation and expression of inflammatory cytokines, and increasing SOD2 activity. This study showed for the first time that VC may alleviate VCM related renal cell injury by regulating ROS/NF-κB in vitro. Further studies are needed to discover the signals involved in the pathway. VC treatment may be a promising and effective method for the prevention of VCM induced nephrotoxicity.
Acknowledgments
Funding: This work was supported by the Shanghai “Rising Stars of Medical Talent” Youth Development Program -Youth Medical Talents - Clinical Pharmacist Program SHWRS (20200120).
Footnote
Data Sharing Statement: Available at http://dx.doi.org/10.21037/apm-20-694
Conflicts of Interest: All authors have completed the ICMJE uniform disclosure form (available at http://dx.doi.org/10.21037/apm-20-694). The authors have no conflicts of interest to declare.
Ethical Statement: The authors are accountable for all aspects of the work in ensuring that questions related to the accuracy or integrity of any part of the work are appropriately investigated and resolved.
Open Access Statement: This is an Open Access article distributed in accordance with the Creative Commons Attribution-NonCommercial-NoDerivs 4.0 International License (CC BY-NC-ND 4.0), which permits the non-commercial replication and distribution of the article with the strict proviso that no changes or edits are made and the original work is properly cited (including links to both the formal publication through the relevant DOI and the license). See: https://creativecommons.org/licenses/by-nc-nd/4.0/.
References
- Filippone EJ, Kraft WK, Farber JL. The Nephrotoxicity of Vancomycin. Clin Pharmacol Ther 2017;102:459-69. [Crossref] [PubMed]
- Liang X, Fan Y, Yang M, et al. A Prospective Multicenter Clinical Observational Study on Vancomycin Efficiency and Safety With Therapeutic Drug Monitoring. Clin Infect Dis 2018;67:S249-55. [Crossref] [PubMed]
- Bosso JA, Nappi J, Rudisill C, et al. Relationship between vancomycin trough concentrations and nephrotoxicity: a prospective multicenter trial. Antimicrob Agents Chemother 2011;55:5475-9. [Crossref] [PubMed]
- Lodise TP, Lomaestro B, Graves J, et al. Larger vancomycin doses (at least four grams per day) are associated with an increased incidence of nephrotoxicity. Antimicrob Agents Chemother 2008;52:1330-6. [Crossref] [PubMed]
- Arimura Y, Yano T, Hirano M, et al. Mitochondrial superoxide production contributes to vancomycin-induced renal tubular cell apoptosis. Free Radic Biol Med 2012;52:1865-73. [Crossref] [PubMed]
- Mergenhagen KA, Borton AR. Vancomycin nephrotoxicity: a review. J Pharm Pract 2014;27:545-53. [Crossref] [PubMed]
- Nishino Y, Takemura S, Minamiyama Y, et al. Targeting superoxide dismutase to renal proximal tubule cells attenuates vancomycin-induced nephrotoxicity in rats. Free Radic Res 2003;37:373-9. [Crossref] [PubMed]
- Sakamoto Y, Yano T, Hanada Y, et al. Vancomycin induces reactive oxygen species-dependent apoptosis via mitochondrial cardiolipin peroxidation in renal tubular epithelial cells. Eur J Pharmacol 2017;800:48-56. [Crossref] [PubMed]
- Oudemans-van Straaten HM, Spoelstra-de Man AM, de Waard MC. Vitamin C revisited. Crit Care 2014;18:460. [Crossref] [PubMed]
- Long CL, Maull KI, Krishnan RS, et al. Ascorbic acid dynamics in the seriously ill and injured. J Surg Res 2003;109:144-8. [Crossref] [PubMed]
- Zhao B, Fei J, Chen Y, et al. Vitamin C treatment attenuates hemorrhagic shock related multi-organ injuries through the induction of heme oxygenase-1. BMC Complement Altern Med 2014;14:442. [Crossref] [PubMed]
- Qi MZ, Yao Y, Xie RL, et al. Intravenous Vitamin C attenuates hemorrhagic shock-related renal injury through the induction of SIRT1 in rats. Biochem Biophys Res Commun 2018;501:358-64. [Crossref] [PubMed]
- Akundi S, Lee YR, Perry GK, et al. Nephrotoxicity in recipients of vancomycin vs. vancomycin with vitamin C. Int J 2015;3:1-15.
- Takigawa M, Yatsu T, Takino Y, et al. High-Dose Vitamin C Preadministration Reduces Vancomycin-Associated Nephrotoxicity in Mice. J Nutr Sci Vitaminol (Tokyo) 2019;65:399-404. [Crossref] [PubMed]
- Buehner M, Pamplin J, Studer L, et al. Oxalate Nephropathy After Continuous Infusion of High-Dose Vitamin C as an Adjunct to Burn Resuscitation. J Burn Care Res 2016;37:e374-9. [Crossref] [PubMed]
- Gurm H, Sheta MA, Nivera N, et al. Vitamin C-induced oxalate nephropathy: a case report. J Community Hosp Intern Med Perspect 2012;2. [Crossref] [PubMed]
- Levine DP. Vancomycin: a history. Clin Infect Dis 2006;42 Suppl 1:S5-12. [Crossref] [PubMed]
- Im DS, Shin HJ, Yang KJ, et al. Cilastatin attenuates vancomycin-induced nephrotoxicity via P-glycoprotein. Toxicol Lett 2017;277:9-17. [Crossref] [PubMed]
- Dalaklioglu S, Tekcan M, Gungor NE, et al. Role of the poly(ADP-ribose)polymerase activity in vancomycin-induced renal injury. Toxicol Lett 2010;192:91-6. [Crossref] [PubMed]
- Ryter SW, Kim HP, Hoetzel A, et al. Mechanisms of cell death in oxidative stress. Antioxid Redox Signal 2007;9:49-89. [Crossref] [PubMed]
- Flynn JM, Melov S. SOD2 in mitochondrial dysfunction and neurodegeneration. Free Radic Biol Med 2013;62:4-12. [Crossref] [PubMed]
- Hajnóczky G, Csordás G, Das S, et al. Mitochondrial calcium signalling and cell death: approaches for assessing the role of mitochondrial Ca2+ uptake in apoptosis. Cell Calcium 2006;40:553-60. [Crossref] [PubMed]
- Elyasi S, Khalili H, Hatamkhani S, et al. Prevention of vancomycin induced nephrotoxicity: a review of preclinical data. Eur J Clin Pharmacol 2013;69:747-54. [Crossref] [PubMed]
- Uckun Z, Guzel S, Canacankatan N, et al. Potential protective effects of naringenin against vancomycin-induced nephrotoxicity via reduction on apoptotic and oxidative stress markers in rats. Drug Chem Toxicol 2020;43:104-11. [Crossref] [PubMed]
- Fouad AA, Albuali WH, Zahran A, et al. Protective effect of naringenin against gentamicin-induced nephrotoxicity in rats. Environ Toxicol Pharmacol 2014;38:420-9. [Crossref] [PubMed]
- Gloire G, Legrand-Poels S, Piette J. NF-kappaB activation by reactive oxygen species: fifteen years later. Biochem Pharmacol 2006;72:1493-505. [Crossref] [PubMed]
- Schoonbroodt S, Ferreira V, Best-Belpomme M, et al. Crucial role of the amino-terminal tyrosine residue 42 and the carboxyl-terminal PEST domain of I kappa B alpha in NF-kappa B activation by an oxidative stress. J Immunol 2000;164:4292-300. [Crossref] [PubMed]
- Kamata H, Manabe T. Hydrogen peroxide activates IkappaB kinases through phosphorylation of serine residues in the activation loops. FEBS Lett 2002;519:231-7. [Crossref] [PubMed]
- Paule B, Terry S, Kheuang L, et al. The NF-kappaB/IL-6 pathway in metastatic androgen-independent prostate cancer: new therapeutic approaches? World J Urol 2007;25:477-89. [Crossref] [PubMed]
- Baker RG, Hayden MS, Ghosh S. NF-κB, inflammation, and metabolic disease. Cell Metab 2011;13:11-22. [Crossref] [PubMed]
- Wang S, Liu Z, Wang L, et al. NF-kappaB signaling pathway, inflammation and colorectal cancer. Cell Mol Immunol 2009;6:327-34. [Crossref] [PubMed]
- Qu S, Dai C, Lang F, et al. Rutin Attenuates Vancomycin-Induced Nephrotoxicity by Ameliorating Oxidative Stress, Apoptosis, and Inflammation in Rats. Antimicrob Agents Chemother 2018;63:e01545-18. [Crossref] [PubMed]