Scutellarin protects against diabetic cardiomyopathy via inhibiting oxidative stress and inflammatory response in mice
Introduction
Diabetes is a chronic, progressive disease and is expected to reach large epidemics over the next few decades. Diabetic cardiomyopathy (DCM) is a major complication of diabetes, which the prominent features are cardiac hypertrophy and fibrosis (1). Progressive of cardiac fibrosis will cause diastolic dysfunction in DCM, which leading to reduced myocardial contractility, and ultimately, heart failure (2). Although there are lots of studies on diabetes, the molecular mechanism of DCM remains poorly understood and further research in this area is urgently required.
The mechanisms of DCM are related with fibrosis and apoptosis. Oxidative stress plays a key role in the initial cellular pathogenesis of DCM. Biomarkers of oxidative stress were reported to be altered in DCM, including decrease of glutathione peroxidase (GSH-px), superoxide dismutase (SOD), advanced glycation end-products (AGEs), nicotinamide adenine dinucleotide phosphate (NADPH) activities and increase of reactive oxygen species (ROS) level, which causes cardiomyocyte injury by triggering apoptosis (3). Besides, in responds to long-term hyperglycemia, multiple pathological changes such as over expression of numerous inflammatory cytokines (e.g., tumor necrosis factor (TNF)-α, interleukin-6 (IL-6) and monocyte chemoattractant protein (MCP)-1], induction of transforming growth factor-β (TGF-β) that contributes to enhanced myocardial fibrosis and deposition of myocardial interstitial collagen (3). The cardiac inflammation and fibrosis will eventually induce irreversible structural remodeling and dysfunction of heart.
Inflammasomes are a group of cytosolic protein complexes, which consist of nucleotide-binding oligomerization domain-like receptor with a pyrin domain (NLRP), apoptosis-associated speck-like protein (ASC), and caspase-1 and are formed to mediate host immune responses to microbial infection and cellular damage (4). Up to now, four inflammasomes have been identified: NLRP1, NLRP3, NLRC4, and absent in melanoma 2 (AIM2) (5). The NLRP3 inflammasome has been fully described and is related with a wide range of diseases, including infectious, autoimmune diseases, and auto-inflammatory (6). The NLRP3 interacts with ASC and then activates caspase-1, which induces maturation and secretion of interleukin (IL)-1β and IL-18. Previous studies found that NLRP3 inflammasome plays a key role in DCM and inhibition of NLRP3 limits the diabetes induced heart injury (7). So, NLRP3 inflammasome maybe an important target for preventing DCM.
Erigeron breviscapus (vant.) Hand-Mazz is a traditional Chinese herbal medicine and widely used in the clinic for the treatment of cerebral ischemia and cardiovascular diseases for a long time (8). Scutellarin (Scu, 4,5,6-trihydroxyflavone-7-glucuronide) is a bioactive flavonoid extracted from Erigeron breviscapus (vant.). Studies indicated that Scu shows various pharmacological properties, including alleviating cardiac fibrosis and decreasing the infarct size and dysfunction of rats with myocardial ischemia, suppressing cardiac hypertrophy, protecting against doxorubicin-induced acute cardiotoxicity (8-10). The molecular mechanism of its pharmacological effects may be related with antioxidation and anti-inflammatory activities (11,12). However, the effect of Scu on DCM remains unknow. The present study was conducted to explore the protected effect of Scu on DCM and its underlying mechanism.
Methods
Animals and experimental protocols
Male C57/B6 mice weighing from 18 to 20 g were purchased from Beijing Vital River Laboratory Animal Technology Co., Ltd. (Beijing, China). The mice were housed under standard laboratory conditions (room temperature at 25±1 °C and humidity of 60% with a 12 h light/dark cycle). Briefly, diabetes was induced in the animals via intraperitoneal (i.p.) injection with freshly prepared streptozotocin (STZ) [dissolved in 0.1 mol/L citrate acid buffer (pH 4.5), 50 mg/kg] for 5 consecutive days. Control mice were injected with a vehicle solution (0.1 mol/L citrate acid buffer) (pH 4.5). One week later, mice with hyperglycemia (3-h fasting blood glucose ≥250 mg/dL) were considered diabetic. Then normal and diabetic mice were divided into 6 groups: control, diabetic model group (DM), DM + Scu (5 mg/kg), DM + Scu (10 mg/kg), DM + Scu (20 mg/kg), DM + pioglitazone (Pio). Scu was administered to mice intraperitoneally and Pio was administrated by oral. Mice in control and DM groups were simply treated normal saline. Four weeks later, myocardial function, myocardial fibrosis, and the levels of inflammatory factors and oxidative stress relative factors were detected. All experimental procedures and protocols were conducted in accordance with the American Physiological Society “Guiding Principles in the Care and Use of Animals” and were approved by the Laboratory Animal Ethics Committee of the Institute of Medicinal Plant Development, Peking Union Medical College (No. 20181016). The mice were put to death by cervical vertebra dislocation after the experiment. The death of the mice was confirmed by observing the complete cessation of breathing and pupil dilation.
Echocardiographic measurements
After 4 weeks, cardiac function was analyzed using echocardiography. Briefly, after fasting for 12 h, the mice were anesthetized using 4% chloral hydrate (400 mg/kg, i.p.), and their chests were shaved. M-mode echocardiography was performed using a Vevo 770™ High Resolution Imaging System (VisualSonics Inc., Canada). Fractional shortening (FS), ejection fraction (EF), Left ventricular (LV) end-diastolic volume (LVVd), and LV end-systolic volume (LVVs) were automatically calculated using an ultrasound machine.
Determination of intracellular ROS
Intracellular ROS generation was detected using a fluorometer. The ROS-sensitive dye 2,7-dichlorodihydrofluorescein diacetate (H2DCFDA) enters cells passively, is converted to dichlorofluorescin diacetate (DCFH), reacts with ROS, and ultimately forms the fluorescent product dichlorofluorescin (DCF). Heart tissues of mice were made into single cell suspension by methodology of enzymatic digestion. The cells were incubated with 20 mM H2DCFDA for 30 min. The fluorescence intensity was measured at 480 nm excitation and 530 nm emission using a fluorescence microplate reader.
Measurement levels of creatine kinase MB isoenzyme (CK-MB), lactate dehydrogenase (LDH), troponin (cTnI), and malondialdehyde (MDA) and catalase (CAT), SOD and GSH-Px in serum
Blood samples were obtained from the inner canthus using a capillary tube under chloral hydrate anesthesia. Samples were allowed to clot for 30 min and then centrifuged at 3,000×g for 15 min at 4 °C. Myocardial injury markers, including CK-MB, LDH, and cTnI in serum were measured using assay kits according to the manufacturer’s protocols. The activities of SOD, MDA, GSH-Px, and CAT were detected using the corresponding kits (Nanjing Jiancheng Bioengineering Institute, Nanjing, China).
HE and Masson staining
Heart tissues were fixed in 10% formalin, dehydrated, and then embedded in paraffin. Paraffin-embedded tissues were sectioned to 5 µm and were placed on glass slides, and then stained with hematoxylin and eosin (HE). Masson’s trichrome staining were used to examine extracellular matrix deposition. The structure was observed under a light microscope (CKX41, 170 Olympus, Tokyo, Japan) by a pathologist blinded to the groups under study. In each group, at least 6 randomly selected sections were studied for the histological changes.
Immunohistochemical analyses
For immunohistochemistry, heart sections were dewaxed, rehydrated through graded ethanol washes, and incubated with 3% hydrogen peroxide for 10 min. After antigen retrieval, the sections were obturated by incubation with bovine serum albumin for 60 min. The sections were continuously incubated with the mouse anti-transforming growth factor-β1 (TGF-β1) and anti-collagen I (Col I) monoclonal antibody (Santa Cruz Biotechnology CA, USA). at 4 °C overnight, and then washed in phosphate-buffered saline (PBS) and incubated with the goat anti-mouse IgG secondary antibody for 1 h at room temperature. The sections were then stained with 0.5 g/L diaminobenzidine for 8 min, resulting in brown staining of the left ventricles of the hearts for viewing with an inverted microscope connected to a digital camera (CKX41,170 Olympus, Tokyo, Japan).
ELISA for detection of IL-6, IL-1β, IL-18, interferon-γ (IFN-γ), tumor necrosis factor-α (TNF-α) and macrophage chemoattractant protein 1 (MCP-1) in serum
Blood samples were obtained from the inner canthus using a capillary tube under chloral hydrate anesthesia. Samples were allowed to clot for 30 min and then centrifuged at 3,000×g for 15 min at 4 °C, and the supernatant was collected for subsequent measurements. The levels of TNF-α, IL-1β, IL-18, IFN-γ, IL-6 and MCP-1 were measured with a double-antibody sandwich ELISA according to the manufacturer’s protocol (Beijing Baochen Biotechnology Co., Ltd. Beijing, China).
Protein extraction and western blot analyses
Briefly, total protein of heart tissues was extracted as previously described (13). After quantifying with bicinchoninic acid (BCA), the proteins were separated by 12% sodium dodecyl sulfate-polyacrylamide gel electrophoresis (SDS-PAGE) and transferred to nitrocellulose membranes. After blocking in 5% (w/v) non-fat milk powder in tris-buffer containing 0.05% (v/v) tween-20 (TBST) for 2 h at room temperature, the membranes were incubated with the appropriate primary antibodies (TGFβ1, COL1, IL18, IL1β, NLRP3, Heme Oxygenase 1 (HO-1), protein kinase B (AKT), and P-AKT) (Santa Cruz Biotechnology CA, USA) at 4 °C overnight incubation. The membranes were then washed with TBST three times and incubated with secondary antibodies for 2 h at room temperature. Protein blots were developed using enhanced chemiluminescence solution. The protein expression levels were visualized with Image Lab Software.
Statistical analysis
Data was presented as mean ± SD. The statistical significance of differences between groups were analyzed using one-way ANOVA followed by Student-Newman-Keuls post hoc test. GraphPad Prism software was used (GraphPad Software, Inc., La Jolla, CA, USA), and significant difference was accepted when P<0.05.
Results
Effects of Scu on blood glucose, body weight, heart weight and heart weight/body weight ratio
As shown in Table 1, Compared with control group, mice in DM group showed higher glucose level (P<0.05), Scu had no effect on blood glucose of DM mice. Body weight (BW) and heart weight (HW) of mice in DM group were lower than control group (P<0.05) and the HW/BW ratio of DM mice were higher than control group (P<0.05). Although BW and HW of mice in Scu treatment groups showed a dose-dependent elevation compared to DM group, there has no significant differences. Scu decreased HW/BW ratio significantly at 10 and 20 mg/kg compared DM group (P<0.05).
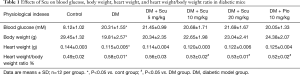
Full table
Effects of Scu on heart function of diabetic mice
We measured cardiac parameters using M-mode echocardiography to investigate the protective effect of Scu on the cardiac function of diabetic mice (Figure 1). Compared with control group, EF, FS, and LVVd significantly decreased in DM group (P<0.05). However, compared with control group, Scu decreased EF and LVVd significantly at 10 and 20 mg/kg (P<0.05) and reduced FS significantly at 20 mg/kg (P<0.05), which proved that Scu could improve heart function of diabetic mice. However, no difference between groups existed as far as LVVs was concerned (Table 2).
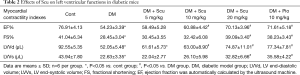
Full table
Effects of Scu on cardiac injury of diabetic mice
As shown in Figure 2A, HE staining of the heart tissues in control group showed regular myocardial fibers and normal morphology of cardiac myocytes. However, myocardial tissue in diabetic model group displayed grievous pathogenic structural changes and remodeling compared to the control group, especially characterized by disordered cellular structures of cardiomyocytes and hypertrophic myocardium. Scu treatment alleviated a majority of those pathologic characteristics. LDH, cTnI, and CK are three important indicators of cardiac injury. As shown in Figure 2B,C,D, the serum levels of LDH, CK-MB, and cTnI significantly increased in DM group (P<0.05). Compared with DM group, Scu decreased levels of LDH and cTnI at 5,10, and 20 mg/kg and inhibited activity of CK-MB at 10 and 20 mg/kg (P<0.05). However, there has no differences between Scu (20 mg/kg) group and Pio group.
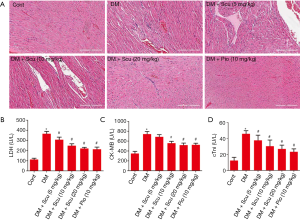
Effects of Scu on myocardial fibrosis of diabetic mice
Masson’s trichrome staining was performed to detected fibrosis and collagen content in diabetic hearts (Figure 3A). The quantitative analysis demonstrated the obvious collagen deposition in the hearts of the DM mice (P<0.05), and was prevented significantly by Scu treatment (P<0.05) (Figure 3B). We further performed immunohistochemical staining to test the expressions of Col I and TGF-β1 in heart tissues. Compared to the control group, the expressions of Col I and TGF-β1 in heart tissues of DM group increased significantly (P<0.05), which were reduced by Scu treatment at 10 and 20 mg/kg (P<0.05) (Figure 4).
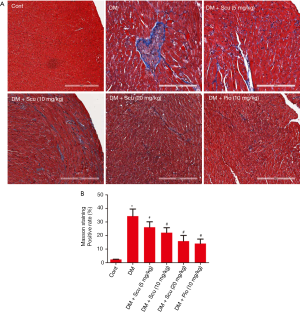
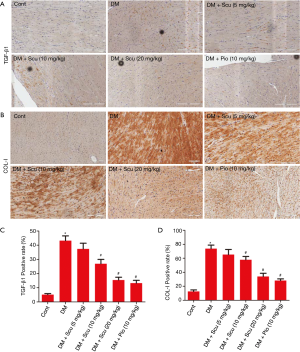
Effects of Scu on ROS level and activities of antioxidant enzymes in diabetic mice
To test if the protective effects of Scu against DCM were related to its antioxidative activity, we measured the oxidative stress associated parameters in serum. We found that mice in DM group showed significant decrease in SOD, CAT, and GSH-Px activities and increase in MDA and ROS production (P<0.05), which were significantly ameliorated by Scu treatment at 10 and 20 mg/kg (P<0.05) (Table 3). These results indicated that Scu can increase the endogenous antioxidant capacity and alleviate oxidative stress.
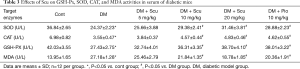
Full table
Effects of Scu on proinflammatory cytokines production in diabetic mice
The levels of proinflammatory cytokines in serum of diabetic mice was determined using ELISA kits. Compared with control group, the levels of TNF-α, IL-1β, IL-18, IFN-γ, IL-6 and MCP-1 in serum of diabetic mice increased significantly (P<0.05). Compared with DM group, Scu decreased levels of IL-1β, IFN-γ, IL-6 and MCP-1 at 10 and 20 mg/kg significantly (P<0.05). Besides, Scu decreased levels of TNF-α and IL-18 at 5, 10, and 20 mg/kg significantly (P<0.05) (Table 4).
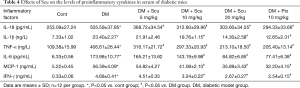
Full table
Scu treatment inhibited NLRP3 signaling pathway in diabetic mice
Western blot showed that the expression of IL-1β, IL-18 and NLRP3 increased in heart tissues of diabetic mice compared with control group (P<0.05). Compared with DM group, Scu decreased the level of IL-1β at 10 and 20 mg/kg and reduced levels of IL-18 and NLRP3 at 5, 10, and 20 mg/kg significantly (P<0.05). These results indicated that Scu may remitted cardiac inflammation in diabetic mice by inhibiting activation of NLRP3 inflammasome. Besides, compared with control group, the protein expression levels of TGF-β1 and Col I in DM group increased significantly (P<0.05) and were inhibited by Scu treatment (P<0.05), which was consistent with the results of immunohistochemical staining (Figure 5).
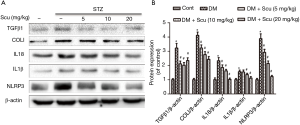
Scu treatment inhibited NF-κB translocation, activated Nrf2/HO-1 pathway and AKT phosphorylation in diabetic mice
Previous study found that NF-κB can regulate activation of NLRP3 inflammasome. We proved that NF-κB nuclear translocation increased significantly in heart tissues of diabetic mice (P<0.05), which were decreased by Scu treatment at 10 and 20 mg/kg (P<0.05) (Figure 6). Nrf2 is a member of the basic leucine zipper transcription factor family and is responsible for upregulating transcriptional antioxidant proteins and phase II detoxifying enzymes. We evaluated the expression of Nrf2 in heart tissues and found that the nuclear Nrf2 expression in DM group increased significantly compared with control group (P<0.05). Compared with the DM group, Scu treatment further increase the nuclear Nrf2 expression significantly (P<0.05). We then examined the protein levels of HO-1, which is well-known target gene of Nrf2. In accordance with the change in Nrf2, the protein expression of HO-1 was moderately increased in DM group and significantly increased in Scu (10, 20 mg/kg) treated mice (P<0.05) (Figure 6). Phosphorylation of AKT decreased significantly in DM group compared with control (P<0.05), which was prevented by Scu treatment at 10 and 20 mg/kg (P<0.05) (Figure 6).
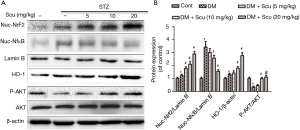
Discussion
In the present study, we found that Scu improved myocardial contractile function, protected against heart injury and fibrosis, and inhibited oxidative stress and inflammation response in STZ-induced diabetic mice, which maybe mediated by inhibiting NF-κB/NLRP3 and activating AKT and Nrf2. Our study showed that Scu might be a potential therapeutic agent to treat DCM.
First, we found that mice in DM group showed lower heart weight and body weight and higher heart/body weight ratio compared with control group. Then we evaluated heart function and M-mode echocardiography showed that LVVd, EF and FS markedly decreased in DM group, which suggested that the myocardial contractile function of mice was impaired after STZ injection. However, Scu treatment decreased heart/body weight ratio and increased myocardial contractile function by elevating levels of LVVd, EF and FS.
CK-MB, LDH, and cTnI are three important biochemical markers, which reflect the injury degree of heart disease (14). Levels of CK-MB, LDH, and cTnI in serum of mice increased significantly in DM group compared with control, which decreased in Scu treatment. HE staining proved that Scu alleviated pathological changes of heart tissues in diabetes mice. These results indicated that Scu attenuated heart injury of diabetic mice.
One of the important pathological structural changes in diabetes is myocardial fibrosis (15,16). TGF-β1 signaling pathway is activated by continuous of hyperglycemia and then increased the level of fibrotic markers in hearts of diabetic mice, including α-SMA, Col I, and Col III (17). The increase of collagen level in left ventricular endomyocardial in diabetes patients may induce decrease of myocardial contractility by reducing EF (18). Inhibition of myocardial fibrosis is one important therapeutic strategy in DCM. In our study, Masson staining showed that STZ injection induced myocardial fibrosis. Immunohistochemistry and western blot proved the activation of TGF-β1 and deposition of Col I in heart tissues of mice in DM group. However, Scu could reduce the myocardial fibrosis and decreased levels of TGF-β1 and deposition of Col I in heart tissues of mice, which indicated that Scu alleviated the fibrosis process in diabetic mice.
Cardiac inflammation plays an important role in the development of DCM. The level of proinflammatory cytokines (IL-1β, TNF-α, IL-18, IL-6, MCP-1 and IFN-γ) increased significantly in DCM, and reducing inflammation can impede DCM development (19,20). Previous studies have demonstrated that the NLRP3 inflammasome complex participates in the progress of cardiovascular diseases, including ischemic heart disease, atherosclerosis, and DCM (19,21). Consistent with other studies, we found that STZ induced higher expression of the NLRP, IL-1β, and IL-18 in heart tissues of diabetic mice. However, Scu treatment significantly inhibited the activation of the NLRP3 inflammasome and the release of proinflammatory cytokines. We also revealed that STZ induced an increase of the level of the nuclear translocation of NF-κB, which inhibited by Scu treatment. These results indicated that Scu may reduce cardiac inflammation and activation of NLRP3 inflammasome by inhibiting nuclear NF-κB translocation.
A lot of studies have shown that the level of ROS increased in diabetes, and DCM is associated with oxidative stress (22). Recent studies proved that Scu provide antioxidant activities in various disease (9,23,24). In our study, we found that Scu reduced oxidative stress in the heart tissues by decreasing levels of ROS and MDA and increasing activities of SOD, CAT and GSH-px. HO-1 is a rate-limiting enzyme that degrades heme (a potent oxidant) to bilirubin (which has antioxidant properties) (25). As an adaptive survival response, HO-1 up-regulated in cells under oxidative stress. Previous studies showed that the expression of HO-1 is regulated by the Nrf2 signaling pathway (26). Nrf2 signaling has an important function in protecting cardiomyocyte from oxidative stress injury (27). Under normal conditions, Nrf2 is located in the cytoplasm and binds with Keap1. Nrf2 can be activated by a range of oxidative and electrophilic stimuli, including ROS, heavy metals, and certain disease processes. The activated Nrf2 is released from Keap1 and translocated to the nucleus, where it activates the transcription of target genes, such as HO-1 (28). In the present study, STZ exposure induced moderate activity of the Nrf2 signaling pathway and caused serious injury to cardiomyocyte. However, treatment with Scu significantly increased Nrf2 translocation, as well as the protein expression of Nrf2 target gene, HO-1, and their enzymatic activity. These results suggested that Scu may protect against DCM via triggering the activation of Nrf2/HO-1 signaling.
PI3K/AKT signaling pathway plays an important role in cell survival. AKT activation could improve contractile function of heart and prevent the develop of DCM (29). Various studies found that PI3K/AKT could inhibit oxidative stress and inflammatory response via regulating Nrf2 and NF-κB (30,31). In our study, the decrease of p-AKT in cardiomyocytes in diabetic mice can be prevented by Scu treatment, indicating that PI3K/AKT may also participate the protecting effect of Scu on DCM.
Conclusions
The results of this study demonstrated that Scu reduced myocardial damage and exerted antifibrotic, anti-inflammatory and antioxidant effects on diabetic mice. The molecular mechanism may be related with activation of AKT and Nrf2/HO-1 and inhibition of NF-κB/NLRP3 signaling pathway. The findings of our study elucidate the potential of Scu for treating DCM.
Acknowledgments
Funding: This study was supported by the National Natural Science Foundation of China (No. 81603334), CAMS Innovation Fund for Medical Sciences (CIFMS) (No. 2019-I2M-1-005), National key research and development program (No. 2018YFC1707408), and Key Laboratory of Chinese Academy of Medical Sciences (No. 2018PT35030).
Footnote
Data Sharing Statement: Available at http://dx.doi.org/10.21037/apm-19-516
Conflicts of Interest: All authors have completed the ICMJE uniform disclosure form (available at http://dx.doi.org/10.21037/apm-19-516). The authors have no conflicts of interest to declare.
Ethical Statement: The authors are accountable for all aspects of the work in ensuring that questions related to the accuracy or integrity of any part of the work are appropriately investigated and resolved. All experimental procedures and protocols were conducted in accordance with the American Physiological Society “Guiding Principles in the Care and Use of Animals” and were approved by the Laboratory Animal Ethics Committee of the Institute of Medicinal Plant Development, Peking Union Medical College (No. 20181016).
Open Access Statement: This is an Open Access article distributed in accordance with the Creative Commons Attribution-NonCommercial-NoDerivs 4.0 International License (CC BY-NC-ND 4.0), which permits the non-commercial replication and distribution of the article with the strict proviso that no changes or edits are made and the original work is properly cited (including links to both the formal publication through the relevant DOI and the license). See: https://creativecommons.org/licenses/by-nc-nd/4.0/.
References
- Jia G, Whaley-Connell A, Sowers JR. Diabetic cardiomyopathy: a hyperglycaemia- and insulin-resistance-induced heart disease. Diabetologia 2018;61:21-8. [Crossref] [PubMed]
- Jin L, Zhang J, Deng Z, et al. Mesenchymal stem cells ameliorate myocardial fibrosis in diabetic cardiomyopathy via the secretion of prostaglandin E2. Stem Cell Res Ther 2020;11:122. [Crossref] [PubMed]
- Palomer X, Román-Azcona MS, Pizarro-Delgado J, et al. SIRT3-mediated inhibition of FOS through histone H3 deacetylation prevents cardiac fibrosis and inflammation. Signal Transduct Target Ther 2020;5:14. [Crossref] [PubMed]
- Chen R, Sun G, Yang L, et al. Salvianolic acid B protects against doxorubicin induced cardiac dysfunction via inhibition of ER stress mediated cardiomyocyte apoptosis. Toxicol Res (Camb) 2016;5:1335-45. [Crossref] [PubMed]
- Platnich JM, Muruve DA. NOD-like receptors and inflammasomes: A review of their canonical and non-canonical signaling pathways. Arch Biochem Biophys 2019;670:4-14. [Crossref] [PubMed]
- Ratajczak MZ, Bujko K, Cymer M, et al. The Nlrp3 inflammasome as a "rising star" in studies of normal and malignant hematopoiesis. Leukemia 2020;34:1512-23. [Crossref] [PubMed]
- Zhang X, Fu Y, Li H, et al. H3 relaxin inhibits the collagen synthesis via ROS- and P2X7R-mediated NLRP3 inflammasome activation in cardiac fibroblasts under high glucose. J Cell Mol Med 2018;22:1816-25. [Crossref] [PubMed]
- Xu LJ, Chen RC, Ma XY, et al. Scutellarin protects against myocardial ischemia-reperfusion injury by suppressing NLRP3 inflammasome activation. Phytomedicine 2020;68:153169 [Crossref] [PubMed]
- Sun XP, Wan LL, Yang QJ, et al. Scutellarin protects against doxorubicin-induced acute cardiotoxicity and regulates its accumulation in the heart. Arch Pharm Res 2017;40:875-83. [Crossref] [PubMed]
- Pan ZW, Zhang Y, Mei DH, et al. Scutellarin exerts its anti-hypertrophic effects via suppressing the Ca2+-mediated calcineurin and CaMKII signaling pathways. Naunyn Schmiedebergs Arch Pharmacol 2010;381:137-45. [Crossref] [PubMed]
- Mo J, Yang R, Li F, et al. Scutellarin protects against vascular endothelial dysfunction and prevents atherosclerosis via antioxidation. Phytomedicine 2018;42:66-74. [Crossref] [PubMed]
- Chledzik S, Strawa J, Matuszek K, et al. Pharmacological Effects of Scutellarin, An Active Component of Genus Scutellaria and Erigeron: A Systematic Review. Am J Chin Med 2018;46:319-37. [Crossref] [PubMed]
- Chen RC, Wang J, Yang L, et al. Protective effects of ginsenoside Re on lipopolysaccharide-induced cardiac dysfunction in mice. Food Funct 2016;7:2278-87. [Crossref] [PubMed]
- Lin R, Duan J, Mu F, et al. Cardioprotective effects and underlying mechanism of Radix Salvia miltiorrhiza and Lignum Dalbergia odorifera in a pig chronic myocardial ischemia model. Int J Mol Med 2018;42:2628-40. [Crossref] [PubMed]
- Wang Y, Li H, Li Y, et al. Coriolus versicolor alleviates diabetic cardiomyopathy by inhibiting cardiac fibrosis and NLRP3 inflammasome activation. Phytother Res 2019; [Crossref] [PubMed]
- Li R, Liu Y, Shan YG, et al. Bailcalin Protects against Diabetic Cardiomyopathy through Keap1/Nrf2/AMPK-Mediated Antioxidative and Lipid-Lowering Effects. Oxid Med Cell Longev 2019;2019:3206542 [Crossref] [PubMed]
- Liao HH, Zhu JX, Feng H, et al. Myricetin Possesses Potential Protective Effects on Diabetic Cardiomyopathy through Inhibiting IkappaBalpha/NFkappaB and Enhancing Nrf2/HO-1. Oxid Med Cell Longev 2017;2017:8370593 [Crossref] [PubMed]
- Li K, Zhai M, Jiang L, et al. Tetrahydrocurcumin Ameliorates Diabetic Cardiomyopathy by Attenuating High Glucose-Induced Oxidative Stress and Fibrosis via Activating the SIRT1 Pathway. Oxid Med Cell Longev 2019;2019:6746907 [Crossref] [PubMed]
- Yang F, Qin Y, Wang Y, et al. Metformin Inhibits the NLRP3 Inflammasome via AMPK/mTOR-dependent Effects in Diabetic Cardiomyopathy. Int J Biol Sci 2019;15:1010-9. [Crossref] [PubMed]
- Li H, Shi Y, Wang X, et al. Piceatannol alleviates inflammation and oxidative stress via modulation of the Nrf2/HO-1 and NF-kappaB pathways in diabetic cardiomyopathy. Chem Biol Interact 2019;310:108754 [Crossref] [PubMed]
- Yu SY, Dong B, Tang L, et al. LncRNA MALAT1 sponges miR-133 to promote NLRP3 inflammasome expression in ischemia-reperfusion injured heart. Int J Cardiol 2018;254:50. [Crossref] [PubMed]
- Li L, Luo W, Qian Y, et al. Luteolin protects against diabetic cardiomyopathy by inhibiting NF-kappaB-mediated inflammation and activating the Nrf2-mediated antioxidant responses. Phytomedicine 2019;59:152774 [Crossref] [PubMed]
- Baluchnejadmojarad T, Zeinali H, Roghani M. Scutellarin alleviates lipopolysaccharide-induced cognitive deficits in the rat: Insights into underlying mechanisms. Int Immunopharmacol 2018;54:311-9. [Crossref] [PubMed]
- Wang Z, Yu J, Wu J, et al. Scutellarin protects cardiomyocyte ischemia-reperfusion injury by reducing apoptosis and oxidative stress. Life Sci 2016;157:200-7. [Crossref] [PubMed]
- Chen YH, Yet SF, Perrella MA. Role of heme oxygenase-1 in the regulation of blood pressure and cardiac function. Exp Biol Med (Maywood) 2003;228:447-53. [Crossref] [PubMed]
- Chen RC, Sun GB, Wang J, et al. Naringin protects against anoxia/reoxygenation-induced apoptosis in H9c2 cells via the Nrf2 signaling pathway. Food Funct 2015;6:1331-44. [Crossref] [PubMed]
- Zhang HJ, Chen RC, Sun GB, et al. Protective effects of total flavonoids from Clinopodium chinense (Benth.) O. Ktze on myocardial injury in vivo and in vitro via regulation of Akt/Nrf2/HO-1 pathway. Phytomedicine 2018;40:88-97. [Crossref] [PubMed]
- Habtemariam S. The Nrf2/HO-1 Axis as Targets for Flavanones: Neuroprotection by Pinocembrin, Naringenin, and Eriodictyol. Oxid Med Cell Longev 2019;2019:4724920 [Crossref] [PubMed]
- Qi K, Zhong J. LncRNA HOTAIR improves diabetic cardiomyopathy by increasing viability of cardiomyocytes through activation of the PI3K/Akt pathway. Exp Ther Med 2018;16:4817-4823. [Crossref] [PubMed]
- Yang HL, Thiyagarajan V, Shen PC, et al. Anti-EMT properties of CoQ0 attributed to PI3K/AKT/NFKB/MMP-9 signaling pathway through ROS-mediated apoptosis. J Exp Clin Cancer Res 2019;38:186. [Crossref] [PubMed]
- Meng T, Fu S, He D, et al. Evodiamine Inhibits Lipopolysaccharide (LPS)-Induced Inflammation in BV-2 Cells via Regulating AKT/Nrf2-HO-1/NF-κB Signaling Axis. Cell Mol Neurobiol 2021;41:115-27. [Crossref] [PubMed]