The expression levels of plasma dimethylglycine (DMG), human maternally expressed gene 3 (MEG3), and Apelin-12 in patients with acute myocardial infarction and their clinical significance
Introduction
Acute myocardial infarction (AMI) has a sudden onset, with severe and long-lasting posterior sternal pain as the main clinical symptom. The disease often progresses rapidly and has many complications, and the mortality rate is extremely high (1). With the increased aging of the population, AMI has become one of the main causes of sudden death in middle-aged and elderly people, and it has been reported that about 8 million people die from AMI every year (2). Early diagnosis and identification of AMI in patients with chest pain, as well as the rapid restoration of blood perfusion to the vessel responsible for the infarct are keys to saving the lives of AMI patients and improving prognosis. Traditional myocardial injury markers, including cardiac troponin I (cTnI) and creatine phosphokinase-isoenzyme-MB (CK-MB), present limitations for the diagnosis of early AMI, such as their low sensitivity and short detection window. Furthermore, these biomarkers can also show an increasing trend in some cardiac or non-cardiac diseases (3,4). Therefore, it is necessary to explore new markers for the early diagnosis of AMI with high sensitivity and specificity.
Currently, the role of plasma dimethylglycine (DMG), human maternally expressed gene 3 (MEG3), and Apelin-12 in cardiovascular disease has attracted increasing clinical attention (5,6). DMG is independently related to incident acute myocardial infarction and enhances risk prediction in patients with stable angina pectoris (7). Elevated DMG is a risk marker of mortality in patients with coronary heart disease (8). Previous research have showed that MEG3 modulates transient receptor potential cation channel subfamily V member 4 (TRPV4) expression to aggravate hypoxia-induced injury in rat cardiomyocytes by sponging miR-325-3p (9). MEG3 knockdown exerts cardioprotection by reducing endoplasmic reticulum stress (ERS)-mediated apoptosis through targeting p53 post-myocardial infarction (10). MEG3 accelerates apoptosis of hypoxic myocardial cells via fork head box O1 (FoxO1) signaling pathway (11). The structural analogue of Apelin-12 [MeArg (1), NLe (10)]-A12 may be a promising basis to create a new drug for the treatment of acute coronary syndrome (12). Patients with ST-segment elevation myocardial infarction (STEMI) receiving primary percutaneous coronary intervention (pPCI) with lower Apelin-12 are more likely to suffer major adverse cardiovascular events (MACEs) in hospital and 2.5 years postprocedure, particularly in those with normal estimated glomerular filtration rate (eGFR) levels (13). Plasma Apelin-12 levels predicts in-hospital major adverse cardiac events in ST-elevation myocardial infarction and the relationship between apelin-12 and the neutrophil/lymphocyte ratio in patients undergoing primary coronary intervention (14). Therefore, this study aimed to analyze the expression and clinical significance of the above indicators in AMI patients and to provide a reference for the early diagnosis of AMI.
We present the following article in accordance with the STARD reporting checklist (available at http://dx.doi.org/10.21037/apm-21-122).
Methods
General information
This study was a prospective observational study. One hundred and ten patients with chest pain suspected of AMI who were admitted to our hospital between December 2018 and June 2020 were included. The inclusion criteria were as follows: (I) patients with a duration of chest pain or discomfort ≥5 min; (II) patients aged >18 years old; (III) patients with suspected AMI who were planning to receive myocardial injury marker testing; and (IV) patients or their family members were informed of the research content and voluntarily signed the informed consent. Patients were excluded based on the following criteria: (I) those with a duration of chest pain >3 h; (II) those with renal insufficiency; (III) those with high or suspected non-coronary chest pain; (IV) those with a clear diagnosis of AMI in another hospital; (V) those with malignant tumors; and (VI) those who had received major surgery or muscle injury before enrollment, which may affect the level of myogenic long noncoding RNAs (lncRNAs). All patients agreed to participate in this study and signed an informed consent form. The study was approved by the medical ethics committee of the Sanya Central Hospital. The study conformed to the provisions of the Declaration of Helsinki (as revised in 2013). According to the inclusion and exclusion criteria, 110 patients were finally included, aged between 46 and 77 years old, with an average of 60.22±11.34 years old. There were 48 males and 62 females, with an average body mass index (BMI) of 23.27±3.45 kg/m2. Eighteen patients had a history of smoking, 67 cases had hypertension, 24 cases had diabetes, and 41 cases had hyperlipidemia. Among all 110 patients, 45 cases had previous coronary heart disease, 17 cases had suffered a myocardial infarction, 21 cases had revascularization, 12 cases had suffered a stroke, 10 cases had peripheral artery disease, 22 cases had arrhythmia, and seven cases had congestive heart failure. The average onset of chest pain in all of the patients was 3.09 (1.77, 5.02) h.
Study method
Basic inspection
The medical history, physical condition, electrocardiogram (ECG), as well as the central laboratory myocardial injury markers and other laboratory tests were routinely collected for all enrolled patients.
Main instruments and kits
Light Cycler480II Fluorescence Quantitative PCR Instrument (Roche, Switzerland), Thermo Fisher high-performance liquid chromatography (HPLC) (Thermo Fisher, USA), and ultra-high-performance liquid chromatography (UHPLC) systems (Thermo Fisher) were used. Apelin-12 enzyme-linked immunosorbent assay (ELISA) Kit was purchased from Geidan Biotechnology Co., Ltd. (Beijing). The Trizol kit, miRcute enhanced microRNA (miRNA) complementary deoxyribonucleic acid (cDNA) first-strand synthesis kit, and miRNA fluorescence quantitative detection kit were purchased from Beijing Tiangen Biochemical Technology Co. Ltd. (Beijing). The primers were purchased from Biotech (Shanghai) Co. Ltd., including MEG3: Upstream primer: 5'-AGAAGGCGAAGAACTGGAATAGAG-3', 5'-AGTTAAAACAAGAAACATTTATTGAAA GCAC-3'; U6: Upstream primer: 5'-GCTTCGGCAGCACATATACTAAAAT-3', Downstream primer: 5'-CGCTTCACGAATTTGCGTGTCAT-3'.
Blood sample collection and determination of DMG, MEG3, Apelin-12 levels
Blood sample collection
Upon admission to the hospital, 6 mL of venous blood was collected from the patients, and was divided into two tubes. One tube was routinely centrifuged to separate the serum and the supernatant was collected and stored in a refrigerator at −80 °C for testing, while the other tube was stored at 4 °C for 30 minutes for the measurement of MEG3.
Determination of DMG, MEG3, and Apelin-12 levels
HPLC was used to measure plasma DMG levels, and ELISA was used to determine Apelin-12 levels. Another blood sample, which was refrigerated at 4 °C for 30 minutes, was centrifuged at 3,000 rpm at room temperature for 15 minutes. The upper layer of plasma was then collected and soaked in 1% diethyl pyrocarbonate (DEPC)water overnight, and then sterilized and dried for subsequent use. The plasma was melted on ice, and 250 µL of the plasma sample was then taken and 750 µL Trizol was added. Next, the samples were mixed and allowed to stand for 5 min. Following this, 200 µL of chloroform was added and the samples were mixed again and allowed to stand for another 10 min. Subsequently, the samples were centrifuged at 12,000 rpm for 10 min at room temperature and the supernatant was obtained. The supernatant was then transferred to a RNase-free Eppendorf (EP) tube, and an equal volume of isopropanol was added to mix with the supernatant. After mixing, the samples were centrifuged, and 1 mL of 75% ethanol was added before centrifuging and drying the samples. The extracted RNA (using the above method) was then dissolved and the total RNA concentration and purity were measured with a spectrophotometer (Thermo Fisher Scientific, USA). The reagent kit in the RNase-free reaction tube was allowed to stand for 5 minutes in a 68 °C water bath. The reaction solution was then taken out and after short centrifugation, it was mixed well, and cDNA was synthesized in a 37 °C water bath for 60 minutes. 2× qPCR SuperMix, upstream primers, and downstream primers were melted in the refrigerator, and an appropriate amount of each was taken out to produce the reaction system (10 µL of 2× qPCR SuperMix; 0.5 µL of each of the upstream and downstream primers; 0.5 µL of each of the upstream and downstream primers of the internal control U6; 1 µLof the cDNA template; 8 µL of doble distilled water (dd H2O)], and centrifuged briefly to mix it. The reaction was set according to the following conditions: 1 min at 95 °C, 45 cycles of 20 s at 95 °C, 31 s at 61 °C and 30 s at 72 °C. The level of lncRNA was then measured, and the relative expression of MEG3 mRNA was expressed as 2−△△Ct.
Diagnostic criteria
All of the clinical data obtained from the patients’ first admission to 90 days of follow-up were collected. Two clinicians then used the double-blind method to read the film and provide the final diagnosis. The diagnosis was AMI, unstable angina (UA), non-coronary cardiogenic chest pain (myocarditis, tachycardia, etc.), non-cardiac chest pain (not involving coronary aortic dissection, pulmonary embolism, etc.), or unexplained chest pain. The consensus diagnosis opinion was then taken as the final diagnosis, and in cases of disagreement, the final judgment was made by the superior physician. The diagnostic criteria of the third edition of the Global Unified Definition Guide for Myocardial Infarction in 2012 was used to diagnose AMI (15). Laboratory tests were used to verify that the myocardial injury markers were either gradually increasing or decreasing, thereby providing clear evidence of acute myocardial ischemia.
Statistical analysis
SPSS 21.0 software (SPSS Inc., Chicago, IL, USA) was used for statistical analysis. Measurement data conforming to a normal distribution was described by (
Results
Comparison of the two groups in terms of their general information
Among the 110 patients with chest pain suspected of AMI, 34 were clinically diagnosed with AMI (15 cases of ST-segment elevation myocardial infarction), and there were 76 non-AMI patients (27 cases of UA, 10 cases of non-coronary chest pain, eight cases of non-cardiogenic chest pain, and 31 cases of unexplained chest pain). The proportion of men, smoking, history of myocardial infarction, and history of congestive heart failure was higher in the AMI group compared to the non-AMI group, and the difference was statistically significant (P<0.05, Table 1).
Comparison of routine examination results between the two groups at admission
The proportion of systolic blood pressure, ECG ST-segment elevation, and ECG dynamic change at admission in the AMI group was higher than that of the non-AMI group, and the difference was statistically significant (P<0.05, Table 2).
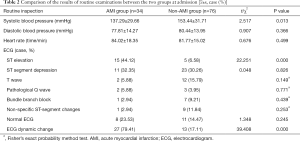
Full table
Comparison of plasma DMG, MEG3, and Apelin-12 levels between the two groups at admission
The levels of plasma DMG, MEG3, and Apelin-12 of patients in the AMI group were higher than those in the non-AMI group upon admission, and the difference was statistically significant (P<0.05, Table 3).

Full table
The diagnostic value of plasma DMG, MEG3, and Apelin-12 levels at admission in the two groups for the diagnosis of AMI
Plasma DMG, MEG3, and Apelin-12 showed diagnostic value for AMI diagnosis at admission. The AUC of MEG3 was higher than that of DMG and Apelin-12, however the difference was not statistically significant (Z=1.378, 0.934, P=0.168, 0.350). Using 0.015 as the cut-off value for MEG3-mRNA, the sensitivity and specificity for diagnosing AMI were 85.29% and 81.58%, respectively (Table 4, Figure 1).

Full table
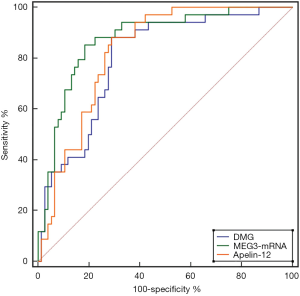
Discussion
AMI is a serious type of coronary heart disease. The main clinical manifestations of AMI are chest pain, acute circulatory disturbance, myocardial injury, and myocardial ischemic necrosis, accompanied by ECG changes. In recent years, although the clinical treatment of coronary heart disease has become more standardized, the mortality rates for patients with AMI remain at a high level (16). Early diagnosis is the key to reducing the mortality rate of AMI. Currently, the clinical diagnosis of AMI is primarily based on the patient’s clinical symptoms, ECG, myocardial injury markers, and other examinations to confirm the diagnosis. The combined detection of traditional myocardial injury markers, such as CK-MB and cTnI, plays an important role in the early diagnosis of AMI. However, although CK-MB and cTnI can increase to a certain extent 3 h after the occurrence of AMI, the increase is only significant around 7 h after the occurrence of AMI and reaches a peak after 12 h. Therefore, in the early stage of AMI, the diagnostic value of traditional myocardial injury markers, such as CK-MB and cTnI, is limited (17,18). In recent years, the clinical application value of DMG in cardiovascular diseases has attracted clinical attention. Studies have pointed out that the plasma DMG level of early AMI patients increases, and the direct catabolite sarcosine level of DMG is also closely related to restenosis after percutaneous coronary intervention (PCI) (19). However, research on the diagnostic value of DMG for early AMI is rare. Our study showed that DMG levels in the AMI group was higher than those in the non-AMI group, suggesting that DMG may potentially be used as a biomarker for the early diagnosis of AMI. ROC curve analysis also confirmed the aforementioned perspective; using 6.833 as the cut-off, the sensitivity and specificity for the early diagnosis of AMI were 88.24% and 71.05%, respectively.
Furthermore, with the continuous development of molecular biology, the role of lncRNA in the occurrence and development of human diseases has also attracted clinical attention. Previous studies have reported that the expression of lncRNA in patients with malignant tumor diseases is dysregulated. Related animal experiments have also confirmed that lncRNAs Steroid receptor RNA Activator (SRA) can serve as a co-activated factor for nuclear receptor signaling, and can thus cause dysfunction of ventricular muscle contraction when lncRNAs expression in zebrafish is down-regulated. Therefore, it is speculated that lncRNAs can be used as a potential biomarker for the diagnosis of early AMI (20,21). MEG3 is the first long-chain RNA that has exhibited a tumor suppressor function. It is highly expressed in the brain, pituitary and adrenal glands, as well as other tissues, and plays an important role in the occurrence and development of tumors (22). Our study showed that the expression of MEG3 mRNA in the AMI group was higher than that in the non-AMI group, suggesting that MEG3 mRNA is similar to DMG and can be used as a serum marker for the early diagnosis of AMI. ROC curve analysis showed that when 0.015 was used as the MEG3 mRNA cut-off, its sensitivity and specificity for diagnosing AMI were 85.29% and 81.58%, respectively, which was slightly better than DMG in terms of specificity.
Apelin is a new type of fat cell factor, which is mainly secreted by adipose tissue and expressed in endothelial cells. It is a specific ligand for angiotensin receptor-like protein J receptor (AP) (23). Studies have shown that Apelin can exert a variety of biological effects such as heart-strengthening, reduction of blood pressure, and reduction of myocardial cell membrane damage through phosphorylation of endothelial nitric oxide synthase (24). Apelin-12 is a subtype of Apelin, which is involved in angiogenesis, oxidative stress, glucose and lipid metabolism, as well as other processes. It can also increase the responsiveness of non-myofibrils to calcium ions via the transient calcium influx of cardiomyocytes so that the damaged myocardium can continue to exert positive muscle strengths. Therefore, its downregulation often leads to a decrease in myocardial function (25). Studies on the expression levels and clinical significance of plasma DMG, MEG3, and Apelin-12 in patients with AMI are relatively rare. Our study showed that Apelin-12 in the AMI group was higher than that in the non-AMI group; when 1.347 µg/L was used as the cut-off, its sensitivity and specificity for diagnosing AMI were 88.24%, and 71.05%, respectively. It is therefore consistent with the sensitivity and specificity of DMG in the early diagnosis of AMI. Based on the results of this study, it can be seen that for patients with suspected AMI and a duration of chest pain less than 6 h, plasma DMG, MEG3, and Apelin-12 can all play an important role in early differential diagnosis. In particular, the level of MEG3 mRNA achieved the best early diagnostic performance. However, this study also had certain limitations that should be noted. There were differences in some baseline data between the AMI and non-AMI groups, which may have had an impact on the research conclusions. Therefore, the subsequent study will continue the direction of the present study with a large sample size.
In summary, for suspected AMI patients with an onset of chest pain of less than 6 h, AMI patients have higher levels of plasma DMG, MEG3, and Apelin-12, which can be used as early diagnostic indicators for AMI. Of these, MEG3 had the best diagnostic efficiency.
Acknowledgments
Funding: Hainan Province Health and Family Planning Industry Research Project (Subject Number: 19A200072).
Footnote
Reporting Checklist: The authors have completed the STARD reporting checklist. Available at http://dx.doi.org/10.21037/apm-21-122
Data Sharing Statement: Available at http://dx.doi.org/10.21037/apm-21-122
Conflicts of Interest: Both authors have completed the ICMJE uniform disclosure form (available at http://dx.doi.org/10.21037/apm-21-122). The authors have no conflicts of interest to declare.
Ethical Statement: The authors are accountable for all aspects of the work in ensuring that questions related to the accuracy or integrity of any part of the work are appropriately investigated and resolved. All patients agreed to participate in this study and signed an informed consent form. The study was approved by the medical ethics committee of the Sanya Central Hospital. The study conformed to the provisions of the Declaration of Helsinki (as revised in 2013).
Open Access Statement: This is an Open Access article distributed in accordance with the Creative Commons Attribution-NonCommercial-NoDerivs 4.0 International License (CC BY-NC-ND 4.0), which permits the non-commercial replication and distribution of the article with the strict proviso that no changes or edits are made and the original work is properly cited (including links to both the formal publication through the relevant DOI and the license). See: https://creativecommons.org/licenses/by-nc-nd/4.0/.
References
- Polak JF, Szklo M, O'Leary DH. Carotid Intima-Media Thickness Score, Positive Coronary Artery Calcium Score, and Incident Coronary Heart Disease: The Multi-Ethnic Study of Atherosclerosis. J Am Heart Assoc 2017;6:e004612. [Crossref] [PubMed]
- Sanchis-Gomar F, Perez-Quilis C, Leischik R, et al. Epidemiology of coronary heart disease and acute coronary syndrome. Ann Transl Med 2016;4:256. [Crossref] [PubMed]
- McEvoy JW, Chen Y, Ndumele CE, et al. Six-Year Change in High-Sensitivity Cardiac Troponin T and Risk of Subsequent Coronary Heart Disease, Heart Failure, and Death. JAMA Cardiol 2016;1:519-28. [Crossref] [PubMed]
- Shouval R, Hadanny A, Shlomo N, et al. Machine learning for prediction of 30-day mortality after ST elevation myocardial infraction: An Acute Coronary Syndrome Israeli Survey data mining study. Int J Cardiol 2017;246:7-13. [Crossref] [PubMed]
- Fan Z, He J, Fu T, et al. Arsenic trioxide inhibits EMT in hepatocellular carcinoma by promoting lncRNA MEG3 via PKM2. Biochem Biophys Res Commun 2019;513:834-40. [Crossref] [PubMed]
- McKinnie SMK, Wang W, Fischer C, et al. Synthetic Modification within the "RPRL" Region of Apelin Peptides: Impact on Cardiovascular Activity and Stability to Neprilysin and Plasma Degradation. J Med Chem 2017;60:6408-27. [Crossref] [PubMed]
- Svingen GF, Ueland PM, Pedersen EK, et al. Plasma dimethylglycine and risk of incident acute myocardial infarction in patients with stable angina pectoris. Arterioscler Thromb Vasc Biol 2013;33:2041-8. [Crossref] [PubMed]
- Svingen GF, Schartum-Hansen H, Ueland PM, et al. Elevated plasma dimethylglycine is a risk marker of mortality in patients with coronary heart disease. Eur J Prev Cardiol 2015;22:743-52. [Crossref] [PubMed]
- Zhou Y, Li X, Zhao D, et al. Long non-coding RNA MEG3 knockdown alleviates hypoxia-induced injury in rat cardiomyocytes via the miR-325-3p/TRPV4 axis. Mol Med Rep 2021;23:18.
- Li X, Zhao J, Geng J, et al. Long non-coding RNA MEG3 knockdown attenuates endoplasmic reticulum stress-mediated apoptosis by targeting p53 following myocardial infarction. J Cell Mol Med 2019;23:8369-80. [Crossref] [PubMed]
- Zhao LY, Li X, Gao L, et al. LncRNA MEG3 accelerates apoptosis of hypoxic myocardial cells via FoxO1 signaling pathway. Eur Rev Med Pharmacol Sci 2019;23:334-40. [PubMed]
- Pisarenko OI, Serebryakova LI, Studneva IM, et al. Effects of structural analogues of apelin-12 in acute myocardial infarction in rats. J Pharmacol Pharmacother 2013;4:198-203. [Crossref] [PubMed]
- Yang L, Zheng T, Wu H, et al. Predictive value of apelin-12 in patients with ST-elevation myocardial infarction with different renal function: a prospective observational study. BMJ Open 2017;7:e018595. [Crossref] [PubMed]
- Topuz M, Oz F, Akkus O, et al. Plasma apelin-12 levels may predict in-hospital major adverse cardiac events in ST-elevation myocardial infarction and the relationship between apelin-12 and the neutrophil/lymphocyte ratio in patients undergoing primary coronary intervention. Perfusion 2017;32:206-13. [Crossref] [PubMed]
- Thygesen K, Alpert JS, Jaffe AS, et al. Third universal definition of myocardial infarction. Eur Heart J 2012;33:2551-67. [Crossref] [PubMed]
- Reed GW, Rossi JE, Cannon CP. Acute myocardial infarction. Lancet 2017;389:197-210. Erratum in: Lancet 2017;389:156. [Crossref] [PubMed]
- Marenzi G, Cosentino N, Boeddinghaus J, et al. Diagnostic and Prognostic Utility of Circulating Cytochrome c in Acute Myocardial Infarction. Circ Res 2016;119:1339-46. [Crossref] [PubMed]
- Hoshino T, Hanai K, Tanimoto K, et al. Development and Evaluation of a New Creatine Kinase MB Mass Determination Assay Using a Latex Agglutination Turbidimetric Immunoassay with an Automated Analyzer. Clin Lab 2016;62:877-85. [Crossref] [PubMed]
- Bai K, Xu W, Zhang J, et al. Assessment of Free Radical Scavenging Activity of Dimethylglycine Sodium Salt and Its Role in Providing Protection against Lipopolysaccharide-Induced Oxidative Stress in Mice. PLoS One 2016;11:e0155393. [Crossref] [PubMed]
- Liu CY, Zhang YH, Li RB, et al. LncRNA CAIF inhibits autophagy and attenuates myocardial infarction by blocking p53-mediated myocardin transcription. Nat Commun 2018;9:29. [Crossref] [PubMed]
- Sarangdhar MA, Chaubey D, Bhatt A, et al. A Novel Long Non-coding RNA, durga Modulates Dendrite Density and Expression of kalirin in Zebrafish. Front Mol Neurosci 2017;10:95. [Crossref] [PubMed]
- Yang Z, Li H, Li J, et al. Association Between Long Noncoding RNA MEG3 Polymorphisms and Lung Cancer Susceptibility in Chinese Northeast Population. DNA Cell Biol 2018;37:812-20. [Crossref] [PubMed]
- Topuz M, Oz F, Akkus O, et al. Plasma apelin-12 levels may predict in-hospital major adverse cardiac events in ST-elevation myocardial infarction and the relationship between apelin-12 and the neutrophil/lymphocyte ratio in patients undergoing primary coronary intervention. Perfusion 2017;32:206-13. [Crossref] [PubMed]
- Tatin F, Renaud-Gabardos E, Godet AC, et al. Apelin modulates pathological remodeling of lymphatic endothelium after myocardial infarction. JCI Insight 2017;2:e93887. [Crossref] [PubMed]
- Sans-Roselló J, Casals G, Rossello X, et al. Prognostic value of plasma apelin concentrations at admission in patients with ST-segment elevation acute myocardial infarction. Clin Biochem 2017;50:279-84. [Crossref] [PubMed]
(English Language Editor: A. Kassem)