Analysis of the influencing factors and nursing strategy for acute atelectasis after traumatic brain injury surgery
Introduction
Traumatic brain injury (TBI) refers to cranial and brain injuries caused by external forces on the cranium, typically due to traffic accidents, falls from heights, slips, and falls (1). Upon suffering a TBI, patients usually exhibit symptoms such as a loss of consciousness, increased intracranial pressure, vomiting, and decreased sputum reflex. These symptoms can easily induce vomit and respiratory secretions to be inhaled into the lungs or block the airway, resulting in atelectasis (2,3). Clinical observations have found that acute atelectasis in patients with TBI is primarily manifested within 24–48 hours postoperatively. Atelectasis can cause cerebral ischemia and hypoxia, which will increase the risk of mortality in patients. Determining the influencing factors for acute atelectasis after TBI will help strengthen clinical monitoring, encourage the adoption of targeted intervention measures, reduce the incidence of acute atelectasis after TBI, and improve the prognosis of patients with TBI.
However, there are few reports regarding the factors affecting acute atelectasis after TBI. Therefore, this article will retrospectively analyze the clinical data of 93 patients with craniocerebral injury who underwent surgical treatment in our hospital, aiming to identify the influencing factors for acute atelectasis after craniocerebral injury. This study also aims to propose a corresponding nursing strategy to provide a reference for clinically reducing the incidence of acute atelectasis after TBI. The research results are reported as follows.
We present the following article in accordance with the STARD reporting checklist (available at http://dx.doi.org/10.21037/apm-21-790).
Methods
General information
A retrospective study design was employed to investigate patients treated for craniocerebral injury in Department of Neurosurgery, Shanghai Punan Hospital from April 2015 to October 2019. All procedures performed in this study involving human participants were in accordance with the Declaration of Helsinki (as revised in 2013). The study was approved by the Medical Ethics Expert Committee of Punan Hospital in Pudong New Area of Shanghai (No.: 2020004). Individual consent for this retrospective analysis was waived. The inclusion criteria were as follows: (I) patients diagnosed with TBI by imaging; (II) patients without lung injury, surgical contraindications, or severe underlying diseases prior to surgery; (III) a craniocerebral injury Glasgow Coma Scale (GCS) of 3–8 points; and (IV) an admission time <6 h after trauma. Patients were excluded based on the following criteria: (I) those with primary brain disease, pulmonary infection, bronchial injury, chronic obstructive pulmonary disease, and other related diseases that affect the study; (II) those who abandoned treatment or transferred in the middle; (III) those with poor compliance; (IV) dying patients; and (V) those with incomplete clinical data.
A total of 93 patients satisfied the above inclusion and exclusion criteria, and were grouped according to whether they had acute atelectasis during the postoperative hospital stay. Twenty-two patients with acute atelectasis were included in the acute atelectasis group, and 71 patients without acute atelectasis were included in the non-acute atelectasis group.
Study methods
Data collection
By consulting the electronic medical records, we obtained clinical data including the patient’s age, gender, GCS score, admission time, injury site, surgical procedure, mannitol usage, operation time, history of vomiting and aspiration (including whether this was combined with underlying diseases such as myasthenia gravis, symmetry Polynervitis, acute myelitis, etc.), mechanical ventilation, tracheotomy, hypoalbuminemia, use of analgesics, inflammatory factors [tumor necrosis factor-α (TNF-α) and interleukin-6 (IL-6)], serum hypoxia-inducible factor-1α (HIF-1α) level, and other information.
Diagnosis of acute atelectasis
The patients’ symptoms and lung radiographic imaging were collected during the postoperative hospitalization period. For symptoms, acute atelectasis patients can have various chest tightness, dyspnea, palpitation, dry cough, weakened lungs, breath sounds. Abnormal lungs in percussion examination (due to solid sound) have lung consolidation and decreased gas. For lung radiographic imaging, we may observe patients’ increased opacity of the affected lobe, bronchovascular crowding, narrowing of the ipsilateral intercostal spaces, compensatory hyperinflation, the compensatory shift of adjoining structures and/or diaphragm, and silhouette sign affecting contiguous mediastinal structures and so on (4).
Statistical analysis
SPSS 17.0 (SPSS Science Inc., Chicago, IL, USA) was used to analyze the data. Classification data was expressed by n (%), and the chi-square (χ2) test was used. Measurement data was expressed by the mean ± standard deviation, and the t-test was used. The clinical data of the patients were analyzed by univariate analysis, and the best cut-off value of related influencing factors was obtained by the area under the receiver operating characteristic curve (ROC) curve (AUC). Multivariate logistic regression analysis was used to test the influencing factors for acute atelectasis after TBI. P<0.05 was considered to be indicative of statistical significance.
Results
Univariate analysis of acute atelectasis after TBI
Of the 93 patients, 22 patients (23.66%) had acute atelectasis during the hospitalization observation period, while the remaining 71 patients (76.34%) did not develop acute atelectasis. In these two groups of patients, there were statistically significant differences in the GCS score, history of vomiting and aspiration, mannitol usage, mechanical ventilation, hypoalbuminemia, and serum HIF-1α levels (P<0.05) (Table 1).
ROC curve analysis of HIF-1α level predicting the risk of acute atelectasis
The AUC area of HIF-1α level predicting acute atelectasis was 0.896 [95% confidence interval (CI): 0.814–0.978, P=0.042], and the cut-off value was 2.12 mmol/L, with a sensitivity of 76.9% and a specificity of 93.3% (Figure 1).
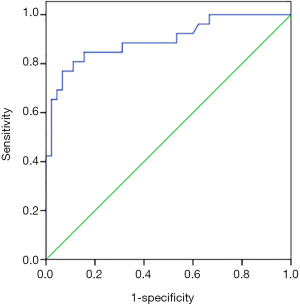
Multivariate logistic regression analysis of acute atelectasis after TBI
Logistic multivariate analysis was performed with acute atelectasis as the dependent variable, and the GCS score, history of vomiting and aspiration, mannitol usage, mechanical ventilation, tracheotomy, hypoalbuminemia, and HIF-1α level as the independent variables, combined with the cut-off value in Figure 1. Logistic multivariate regression analysis showed that a history of vomiting and aspiration [odds ratio (OR) 3.908, 95% CI: 1.201–12.712], mechanical ventilation (OR 3.250, 95% CI: 1.139–9.271), hypoalbuminemia (OR 5.741, 95% CI: 1.926–17.113), HIF-1α ≥2.12 mmol/L (OR 6.623, 95% CI: 2.364–16.346) were independent risk factors for acute atelectasis after TBI (Table 2).
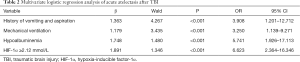
Full table
Discussion
The injured brainstem in TBI may direct damage to respiratory function. Besides, TBI patients often have varying degrees of impaired consciousness and airway self-protection ability. Vomiting and aspiration events are frequent for TBI patients, which can easily lead to airway occlusion and lung infection, leading to atelectasis and severely reducing the patient’s oxygenation level (5). These reasons all increase the duration of mechanical ventilation in TBI patients, thereby increasing the risk of ventilator-induced lung injury.
Partial or complete reversible occlusion of airways can cause atelectasis. The degree of lung collapse and the prolonged time is related to the severity. Atelectasis can cause mechanical vascular occlusion and reactive vasoconstriction, leading to increased right ventricular afterload, making hemodynamic instability (6). Besides, atelectasis can cause the alveolar-blood exchange of CO2 and O2 to be hindered. Arterial blood gas abnormalities may affect brain metabolisms, such as hypercapnia and severe hypoxemia, which can reduce cerebrovascular resistance. Increased cerebral blood volume and intracranial pressure will result in unstable intracranial conditions and affect a lousy prognosis.
There is no consensus on the prevention and treatment of atelectasis (7). Atelectasis can be caused by various mechanisms. Airway obstruction can cause obstructive atelectasis. Large pleural effusion can cause passive atelectasis. Increased intra-abdominal pressure can cause compressive atelectasis. Increased alveolar surface tension can cause adhesive atelectasis. Therefore, atelectasis can be classified and treated according to the cause.
Reducing the secretory burden should focus on the recovery and maintenance of airway patency. Atomization and fiberoptic bronchoscope can restore obstructive atelectasis caused by mucus. Exercise, sighing, and deep breathing can help the sober patient. We can use a lung recruitment strategy to treat passive and adhesive atelectasis for patients with mechanical ventilation.
For TBI patients who need mechanical ventilation support, the lung-protective ventilation strategy may not protect the lungs while maintaining arterial partial pressure of oxygen (PaO2) in the range needed by TBI patients, nor can it prevent and treat atelectasis in TBI patients. The use of a lung-protective ventilation strategy in acute respiratory distress syndrome (ARDS) patients can significantly reduce ventilator-associated lung injury incidence (8). Lung-protective ventilation strategy advocates the appropriate reduction of ventilation (lower tidal volume and plateau pressure) in ARDS patients and acceptance of the resulting low partial pressure of blood oxygen (55–80 mmHg) (9). However, due to TBI characteristics, patients often need a higher level of PaO2 to make oxygen enter the mitochondria of nerve cells across the concentration gradient to participate in aerobic metabolism. Dellazizzo et al. found that the minimum PaO2 required to maintain partial pressure of brain tissue oxygen above 20 mmHg in TBI patients is 94 mmHg (10), which was much higher than the PaO2 >60 mmHg in patients with TBI proposed in the Guidelines for the Management of Severe Traumatic Brain Injury (11). PaO2 =94 mmHg is only the lowest value that can maintain the essential oxygen supply of brain tissue. It may mean that TBI patients need a higher PaO2 than 94 mmHg to ensure that the brain gets enough oxygen for a good prognosis. Simultaneously, some researchers have reported that high blood oxygen concentration will also increase the mortality of patients with TBI (12). TBI patients’ respiratory support strategies need to prevent and treat atelectasis and maintain PaO2 within a reasonable range remain to be widely explored.
Active preventive measures for atelectasis can reduce the risk of atelectasis. It helps maintain the expected levels of arterial partial pressure of carbon dioxide and PaO2, maintain the expected level of aerobic metabolism of nerve cells and stabilize the intracranial pressure. Therefore, timely detection and effective treatment of atelectasis are significant for severe neurological patients. This article analyzed the epidemiology of whether acute atelectasis occurred in 93 cases of TBI, and found that 22 cases of acute atelectasis occurred [an incidence rate of 23.66% (22/99)].
At present, the mechanism of acute atelectasis after TBI has not been clarified and may be caused by the interaction of multiple factors. We conducted a univariate analysis of the clinical data of 93 patients with TBI, and found that there were significant differences in the GCS score, history of vomiting and aspiration, mannitol usage, mechanical ventilation, tracheotomy, hypoalbuminemia, and serum HIF-1α level between patients with and without acute atelectasis. Multivariate logistic regression analysis found that a history of vomiting and aspiration, mechanical ventilation, hypoalbuminemia, and HIF-1α ≥2.12 mmol/L were independent risk factors for acute atelectasis after TBI.
Pandian et al. reported that tracheotomy is a risk factor for lung infection in patients with TBI (13). The reasons for this are as follows. Firstly, atelectasis caused by vomiting is primarily a result of blockage of the bronchial ducts by food or respiratory secretions. Therefore, it is clinically recommended for patients with craniocerebral injury and swallowing dysfunction to consume foods with uniform density, appropriate viscosity, and foods that are not easy to disperse (such as viscous semi-liquid, semi-solid, concentrated liquid food, etc.) as much as possible (14-16). At the same time, patients with TBI should be guided to avoid excessive mouth volume during feeding to prevent excessive food from leaking out of the mouth or causing aspiration. If the patient’s swallowing function is weak, nasal feeding care can be administered. Before nursing, the head of the bed should be raised appropriately, and nasal feeding should be given for 0.5 h after sputum suction. It is important to note that sucking sputum is strictly forbidden after nasal feeding. If the patient has a swallowing reflex or coughing reaction, nasal feeding should be stopped immediately, and appropriate sputum expectoration measures should be taken.
Secondly, during mechanical ventilation, if the intubation is too deep, it can quickly cause the entire lung to collapse. At the same time, it causes unilateral lung ventilation, leading to shrinkage of alveoli on the other side due to reduced or no ventilation, which will induce atelectasis (17). Therefore, it is recommended that before operating on patients who use mechanical ventilation, nursing staff should be fully trained in relevant knowledge to ensure that they master the relevant nursing methods and precautions of artificial airways for mechanically-ventilated patients. In order to prevent lung atrophy due to excessive suction of negative pressure, negative pressure should be controlled at 150–200 mmHg. If the suction time is too long, it is easy to interrupt positive pressure ventilation and cause iatrogenic atelectasis, and thus, the suction time should be less than 15 s. At the same time, nursing staff should not leave the ventilator during sputum suction to avoid airway pressure imbalance and interruption of ventilation.
Thirdly, the albumin (ALB) level can be used to assess the prognosis of cranial injuries (18). Under conditions of stress, such as head trauma or surgery, the body’s immune function declines. Also, especially after Enterobacteriaceae infection, the expression of ALB mRNA in patients is inhibited, and hypoproteinemia is prone to occur. Hypoproteinemia will weaken respiratory defenses and immune function, making patients with TBI more vulnerable to pathogenic microorganisms, viruses, and various inflammatory cells (19). The invasion of a large number of bacteria can aggravate lung infections, promote alveolar exudation, increase secretions, block alveoli, and induce atelectasis. Therefore, the patient’s recovery should be closely followed-up postoperatively and nutritional support should be strengthened according to individual circumstances. The intake of high-protein, high-calorie, and high-vitamin foods should also be increased. Based on the patient’s recovery, amino acid preparations, hydrolyzed protein, glucose, etc. should be administered to improve the immune function of the patient’s respiratory tract and prevent the occurrence of atelectasis.
Fourthly, HIF-1α plays an important regulatory role in erythropoiesis, glycolysis, and cell proliferation (20,21). Studies have confirmed that HIF-1α is closely related to the progression of lung disease under hypoxia (22-24). Intracranial hypoxia caused by TBI can enable HIF-1α to regulate the tolerance of epithelial cells by affecting the transcription and expression of nitric oxide synthase under hypoxic conditions (25,26). Decreased release of nitric oxide will cause continuous vasoconstriction and increase airway resistance. In this paper, it was found that the HIF-1α cut-off value of 2.12 mmol/L had a high sensitivity and specificity in predicting the occurrence of acute atelectasis after TBI. Therefore, for patients with abnormally elevated serum HIF-1α levels, we must closely monitor whether their airways are blocked. Diluting and draining respiratory secretions via airway humidification, ultrasonic atomization, vibration sputum, and other methods to keep the airway unobstructed. High-flow oxygen therapy is given to patients who are evacuated from the ventilator. Adequate oxygen therapy can effectively increase lung ventilation, lung volume, oxygen and ventilation, and prevent alveolar atrophy and atelectasis.
Even though our findings are interesting, there are two limitations. Firstly, this study is not a large sample size study. Although the current results have a particular significance, in the future, we plan to expand the sample size or join the multicenter study further to explore the study of TBI patients with atelectasis. Secondly, this study is a retrospective study. The data that could be collected was limited and prone to selection bias or recalls bias. However, this study’s result provides a basis for further design of prospective research to improve the reliability of the research results.
In summary, a history of vomiting and aspiration, mechanical ventilation, hypoalbuminemia, and high expression of HIF-1α are all independent risk factors for acute atelectasis after TBI. Medical staff should strengthen the monitoring of the postoperative condition of patients with TBI, instruct patients to swallow properly, keep the airway unobstructed, offer balanced nutrition, and regularly check related indicators.
Acknowledgments
Funding: This study was funded by the Outstanding Clinical Discipline Project of Shanghai Pudong (PWYgy2018-04) and the National Natural Science Fund (No. 81571883).
Footnote
Reporting Checklist: The authors have completed the STARD reporting checklist. Available at http://dx.doi.org/10.21037/apm-21-790
Data Sharing Statement: Available at http://dx.doi.org/10.21037/apm-21-790
Conflicts of Interest: All authors have completed the ICMJE uniform disclosure form (available at http://dx.doi.org/10.21037/apm-21-790). All authors report funding from the Outstanding Clinical Discipline Project of Shanghai Pudong (PWYgy2018-04) and the National Natural Science Fund (No. 81571883).
Ethical Statement: The authors are accountable for all aspects of the work in ensuring that questions related to the accuracy or integrity of any part of the work are appropriately investigated and resolved. All procedures performed in this study involving human participants were in accordance with the Declaration of Helsinki (as revised in 2013). The study was approved by the Medical Ethics Expert Committee of Punan Hospital in Pudong New Area of Shanghai (No.: 2020004). Individual consent for this retrospective analysis was waived.
Open Access Statement: This is an Open Access article distributed in accordance with the Creative Commons Attribution-NonCommercial-NoDerivs 4.0 International License (CC BY-NC-ND 4.0), which permits the non-commercial replication and distribution of the article with the strict proviso that no changes or edits are made and the original work is properly cited (including links to both the formal publication through the relevant DOI and the license). See: https://creativecommons.org/licenses/by-nc-nd/4.0/.
References
- Svedung Wettervik T, Howells T, Hillered L, et al. Mild Hyperventilation in Traumatic Brain Injury-Relation to Cerebral Energy Metabolism, Pressure Autoregulation, and Clinical Outcome. World Neurosurg 2020;133:e567-e575. [Crossref] [PubMed]
- Zhang X, Fu Z, Gong G, et al. Implementation of diffusion-weighted magnetic resonance imaging in target delineation of central lung cancer accompanied with atelectasis in precision radiotherapy. Oncol Lett 2017;14:2677-82. [Crossref] [PubMed]
- Hu PJ, Pittet JF, Kerby JD, et al. Acute brain trauma, lung injury, and pneumonia: more than just altered mental status and decreased airway protection. Am J Physiol Lung Cell Mol Physiol 2017;313:L1-L15. [Crossref] [PubMed]
- Marini JJ. Acute Lobar Atelectasis. Chest 2019;155:1049-58. [Crossref] [PubMed]
- Peroni DG, Boner AL. Atelectasis: mechanisms, diagnosis and management. Paediatr Respir Rev 2000;1:274-8. [Crossref] [PubMed]
- Enjeti S, Terry PB, Menkes HA, et al. Mechanical factors and the regulation of perfusion through atelectatic lung in pigs. J Appl Physiol Respir Environ Exerc Physiol 1982;52:647-54. [Crossref] [PubMed]
- Schindler MB. Treatment of atelectasis: where is the evidence? Crit Care 2005;9:341-2. [Crossref] [PubMed]
- Ladha K, Vidal Melo MF, McLean DJ, et al. Intraoperative protective mechanical ventilation and risk of postoperative respiratory complications: hospital based registry study. BMJ 2015;351:h3646. [Crossref] [PubMed]
- Brower RG, Matthay MA, Morris A, et al. Ventilation with lower tidal volumes as compared with traditional tidal volumes for acute lung injury and the acute respiratory distress syndrome. N Engl J Med 2000;342:1301-8. [Crossref] [PubMed]
- Dellazizzo L, Demers SP, Charbonney E, et al. Minimal PaO2 threshold after traumatic brain injury and clinical utility of a novel brain oxygenation ratio. J Neurosurg 2018; [Crossref] [PubMed]
- Carney N, Totten AM, O'Reilly C, et al. Guidelines for the Management of Severe Traumatic Brain Injury, Fourth Edition. Neurosurgery 2017;80:6-15.
- Alali AS, Temkin N, Vavilala MS, et al. Matching early arterial oxygenation to long-term outcome in severe traumatic brain injury: target values. J Neurosurg 2019;132:537-44. [Crossref] [PubMed]
- Pandian V, Datta M, Nakka S, et al. Intensive Care Unit Readmission in Patients With Primary Brain Injury and Tracheostomy. Am J Crit Care 2019;28:56-63. [Crossref] [PubMed]
- Messing BP, Ward EC, Lazarus CL, et al. Prophylactic Swallow Therapy for Patients with Head and Neck Cancer Undergoing Chemoradiotherapy: A Randomized Trial. Dysphagia 2017;32:487-500. [Crossref] [PubMed]
- Steele CM, Alsanei WA, Ayanikalath S, et al. The influence of food texture and liquid consistency modification on swallowing physiology and function: a systematic review. Dysphagia 2015;30:2-26. Erratum in: Dysphagia 2015;30:272-3. [Crossref] [PubMed]
- Umemoto G, Furuya H. Management of Dysphagia in Patients with Parkinson's Disease and Related Disorders. Intern Med 2020;59:7-14. [Crossref] [PubMed]
- Griffin GD. The injured brain: TBI, mTBI, the immune system, and infection: connecting the dots. Mil Med 2011;176:364-8. [Crossref] [PubMed]
- Chen D, Bao L, Lu SQ, et al. Serum albumin and prealbumin predict the poor outcome of traumatic brain injury. PLoS One 2014;9:e93167 [Crossref] [PubMed]
- Qin J, Qin Y, Wu Y, et al. Application of albumin/globulin ratio in elderly patients with acute exacerbation of chronic obstructive pulmonary disease. J Thorac Dis 2018;10:4923-30. [Crossref] [PubMed]
- Betensley A, Sharif R, Karamichos D. A Systematic Review of the Role of Dysfunctional Wound Healing in the Pathogenesis and Treatment of Idiopathic Pulmonary Fibrosis. J Clin Med 2016;6:2. [Crossref] [PubMed]
- Guo L, Xu JM, Liu L, et al. Hypoxia-Induced Epithelial-Mesenchymal Transition Is Involved in Bleomycin-Induced Lung Fibrosis. Biomed Res Int 2015;2015:232791 [Crossref] [PubMed]
- Hua Q, Mi B, Xu F, et al. Hypoxia-induced lncRNA-AC020978 promotes proliferation and glycolytic metabolism of non-small cell lung cancer by regulating PKM2/HIF-1α axis. Theranostics 2020;10:4762-78. [Crossref] [PubMed]
- Zhou F, Du J, Wang J. Albendazole inhibits HIF-1α-dependent glycolysis and VEGF expression in non-small cell lung cancer cells. Mol Cell Biochem 2017;428:171-8. [Crossref] [PubMed]
- Wohlkoenig C, Leithner K, Olschewski A, et al. TR3 is involved in hypoxia-induced apoptosis resistance in lung cancer cells downstream of HIF-1α. Lung Cancer 2017;111:15-22. [Crossref] [PubMed]
- De la Garza MM, Cumpian AM, Daliri S, et al. COPD-Type lung inflammation promotes K-ras mutant lung cancer through epithelial HIF-1α mediated tumor angiogenesis and proliferation. Oncotarget 2018;9:32972-83. [Crossref] [PubMed]
- Chen WG, Sun J, Shen WW, et al. Sema4D expression and secretion are increased by HIF-1α and inhibit osteogenesis in bone metastases of lung cancer. Clin Exp Metastasis 2019;36:39-56. [Crossref] [PubMed]
(English Language Editor: A. Kassem)