Application of velocity vector imaging (VVI) in the evaluation of left ventricular myocardial contractile strain and dyssynchrony before and after stent implantation in patients with coronary heart disease
Introduction
In China, there are currently 330 million patients suffering from cardiovascular disease, among which 11 million are coronary heart disease (CHD) patients, the incidence rate of CHD was from 105.716 per 100,000 in 2011 to 111.920 per 100,000 in 2017 (1), the incidence is increasing every year. Cardiovascular disease mortality ranks first, accounting for approximately two out of every five deaths (2). Cardiovascular disease seriously threatens the health of Chinese people and is a significant challenge for medical professionals. CHD refers to myocardial contraction and diastolic disorders caused by coronary artery atherosclerosis and other lesions that cause narrowing of the blood vessel lumen and insufficient blood supply, which may further develop into organic diseases such as myocardial necrosis. The vast majority of CHD patients have typical chest pain during the onset, chest compression and suffocation, which radiate to the neck, arms, back, stomach and other parts. At the same time, some patients will have shortness of breath, nausea, vomiting, sweating, dizziness, and other symptoms. Severe cases can be fatal due to heart failure, and thus, an effective channel should be established promptly to allow the myocardium to be reperfused as soon as possible, improve blood supply to the heart, and effectively reduce the occurrence of myocardial infarction and sudden death.
Percutaneous coronary intervention (PCI) refers to the insertion of an artificial catheter-like structure into the stenosis of the blood vessel through interventional means to play a supporting role, thereby achieving the purpose of recanalizing the blood vessel and restoring blood supply (3), it is suitable for patients with chronic stable CHD with large range of evidence of myocardial ischemia, high-risk patients with unstable angina pectoris and non ST segment elevation myocardial infarction, and patients with acute ST segment elevation myocardial infarction. After decades of development, PCI technology has become increasingly mature and has gradually become the main treatment for CHD (4). More than 560,000 patients underwent PCI in 2015 (5). PCI has played a particularly key role in improving the treatment success rate of patients with acute myocardial infarction, and has greatly improved the prognosis and quality of life of patients with CHD, especially myocardial infarction patients (6). However, evaluation of myocardial reperfusion after PCI remains a major clinical challenge. Among the numerous methods, echocardiography is widely used clinically owing to its advantages including simplicity, non-invasiveness, and reproducibility. However, conventional echocardiography mainly measures the left ventricular ejection fraction (LVEF) and observes the semi-quantitative method of ventricular wall motion abnormality to indirectly reflect the reperfusion of the myocardium and the recovery of vitality after PCI. The analysis of abnormal ventricular wall motion is mainly carried out by visual observation of the movement displacement of the endocardium and the rate of ventricular wall thickening. Measurement results are easily affected by subjective factors such as personal experience and technology, and the accuracy and repeatability of this method are relatively poor, and cannot provide a quantitative analysis result.
Velocity vector imaging (VVI) is a technique for studying myocardial structural mechanics and analyzing local cardiac function. It is based on two-dimensional images, and is not affected by the overall movement of the heart and the passive traction of adjacent myocardial segments. VVI can more accurately estimate the local myocardium. Deformation can more effectively judge the motion function of the ventricular wall. It can quantitatively measure myocardial motion speed, strain (ε), and strain rate (SR) (7). Studies have shown that with VVI, the longitudinal strain, strain rate, and peak time in CAD patients are easy to obtain and reproducible (8). The peak time and strain rate of myocardial contraction speed measured by VVI; the standard deviation of peak time can effectively evaluate the non-homogeneous movement of the myocardium in patients with myocardial infarction with or without heart failure (9). Previous studies have demonstrated the standard deviation of the peak time of myocardial contraction in patients with normal ejection fraction and CHD; the standard deviation of early diastolic velocity peak time in patients with normal ejection fraction was significantly higher than that in the CHD group, and there was asynchrony of myocardial movement (10).
At present, there are few reports on the use of VVI technology to evaluate the efficacy of PCI. The main purpose of the present study was to use VVI technology to observe the left ventricular myocardial contraction strain and asynchronous movement before and after PCI, and to explore the value of VVI technology in evaluating the efficacy, prognosis, and follow-up of CHD patients after PCI.
We present the following article in accordance with the STROBE reporting checklist (available at https://dx.doi.org/10.21037/apm-21-1540).
Methods
Research objects
Patients who underwent PCI for CHD in the Department of Cardiology of Affiliated Hospital of Jiangnan University from March 2019 to October 2020 were enrolled in this study. The inclusion criteria were as follows: (I) patients aged ≥18 years old; (II) diagnosis confirmed as CHD following medical history inquiry, clinical signs, electrocardiogram, myocardial enzyme spectrum, coronary angiography, etc.; (III) >75% stenosis of single or multiple main coronary arteries confirmed by coronary angiography; and (IV) patients who successfully underwent PCI treatment. All patients provided informed consent and were willing to participate and cooperate with the examination.
The exclusion criteria were as follows: (I) patients with congenital heart disease, restrictive cardiomyopathy, severe arrhythmia, dilated cardiomyopathy, atrioventricular block, arrhythmogenic right ventricular cardiomyopathy, severe heart valve disease, hypertrophic cardiomyopathy, myocardial insufficiency, and pericardial disease; (II) patients that had undergone various cardiac surgeries and pacemaker installation; (III) those with severe liver and kidney dysfunction, severe ischemic hypoxic disease, and a history of food and drug allergy; (IV) patients who were unable to cooperate with the examination due to various factors and those who could not follow the study protocol completely.
Finally, 35 CHD patients were included as the case group, which was then divided into 1 day before PCI (group A), 7 days after PCI (group B), and 30 days after PCI (group C). VVI technology was used to analyze the continuous dynamic two-dimensional images of the patients’ left ventricles 1 day before PCI, 7 days after surgery, and 30 days after surgery. The 16-segment myocardial longitudinal, radial, and circumferential strain peak time standard deviation was calculated and corrected with the cardiac cycle time. The 16-segment myocardial longitudinal, radial, and circumferential strain delay index was used as the asynchronous exercise parameter. In addition, 35 cases of 16-segment myocardial longitudinal, radial, and circumferential strain delay indexes from the outpatient health checkup of our hospital from March 2019 to October 2020 were measured as controls (Figure 1). This study was approved by the Ethics Committee of Affiliated Hospital of Jiangnan University (KY20132) and was conducted in accordance with the Declaration of Helsinki (as revised in 2013). All participants provided informed consent.
Instrument
We used the Siemens Acuson SC2000 color ultrasonic diagnostic instrument (Siemens, Munich, Germany), ultrasonic probe 4V1c, probe frequency setting 3.0–4.0 MHz, frame frequency 50–70 frames/sec. The VVI analysis software used was the instrument’s own.
Inspection
The subjects were placed in the left side lying position, connected to the bedside electrocardiograph, and asked to breathe calmly. The left ventricular apical four-chamber heart, two-chamber heart, three-chamber heart long axis view and the mitral valve, papillary muscle, and apical horizontal short axis were collected. Continuous dynamic two-dimensional images of a single cardiac cycle of the cut surface were taken, which measured the patient’s left ventricular end diastolic diameter (LVEDD), left ventricular end systolic diameter (LVESD), and left ventricle ejection fraction (LVEF). Satisfactory images with a clear and complete display of the ventricular endocardium were stored on the workstation, ready to be entered into the VVI software background analysis and processing.
Image analysis
Two-dimensional (apical four-chamber, three-chamber, and two-chamber long axis) images were collected transthoracically for two consecutive cardiac cycles, and VVI software was used to delineate the endocardial surface of the left ventricle. Each section was delineated three times and the average was taken. Myocardial deformation was automatically tracked. We divided the left ventricular myocardium into 16 segments according to the section method of the left ventricular wall of the American Society of Echocardiography (11), and obtained the strain curves of 16 myocardial segments (see Figure 2).
Observation index
After entering the VVI workstation, the images were fixed at the end of systole, and the boundary of the endocardium was manually traced point by point on a single image. In the long axis section, the reference mark was placed at the apex of the heart, and the dynamic VVI was displayed by clicking ‘run’. The longitudinal strain value, radial strain value, and circumferential strain value of each segment of the myocardium were measured. The values obtained are the averages of three cardiac cycles.
Statistical analysis
SPSS 22.0 (SPSS Inc., Chicago, IL, USA) was used for statistical analysis. Measurement data with a normal distribution was expressed by x±s. The t-test of independent samples was used for comparison between groups. The Chi-square test was used for comparison of count data between groups. P<0.05 indicated a statistically significant difference.
Results
Baseline data
There were no statistically significant differences between the case and control groups in terms of age, sex, blood pressure, heart rate, smoking status, and other general data (P>0.05, Table 1).
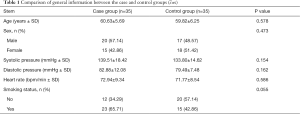
Full table
Comparison of cardiac function parameters between the control and case groups before and after treatment
There were no significant differences in the LVEF, LVEDD, and LVESD between the case and control groups (P>0.05). Furthermore, there were no significant differences in LVEF, LVEDD, and LVESD between the case and control groups 30 days after PCI Treatment (P>0.05). These results showed that the myocardial strain parameters measured before and after PCI could not reflect the changes of ischemic myocardium (Table 2).
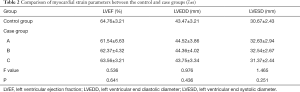
Full table
Comparison of myocardial strain parameters between the control and case groups before and after treatment
The peak systolic longitudinal and radial strain values at 1 month after treatment were higher than those before treatment. The differences among myocardial segments were statistically significant, except for the apical septum, base anterior, apical anterior, and base inferior segments (P<0.05). The peak systolic longitudinal and radial strain values at 1 week after treatment were not significantly different from those at 1 month after treatment, except for the base anterior septum, mid anterior, posterior, and inferior myocardial segments (P>0.05, Tables 3,4).
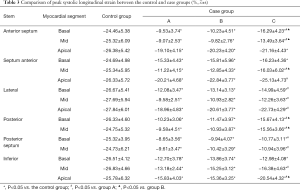
Full table
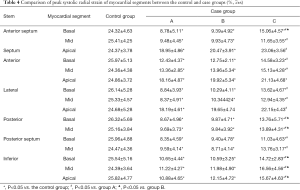
Full table
Discussion
Segmental motion abnormality of the ventricular wall is a characteristic index of myocardial ischemia and infarction. Numerous studies have reported that the ventricular wall segment with reduced contraction speed is well correlated with the supply area of coronary artery stenosis (12). The main trunk of the coronary artery runs along the surface of the heart, and its small branches often penetrate into the myocardium in a direction perpendicular to the surface of the heart and branch into a mesh in the subendocardium. This branching method will cause the subendocardial myocardium to reduce the coronary blood flow (13). When the degree of stenosis of the main coronary artery is greater than 75%, the hemodynamics of the coronary arteries will change, causing myocardial ischemia, decreased pumping function, delayed myocardial contraction, decreased contraction degree, and asynchrony of contraction. At the same time, the electrophysiology will also be abnormal, which is positively correlated with the degree of coronary artery stenosis (14). When the ventricles are not synchronized, the contraction of the normal ventricular muscles causes blood flow to the delayed contraction wall or segment, resulting in local myocardial expansion and non-synchronization, which may ultimately lead to local myocardial dilation and ventricular aneurysm formation. The unsynchronized contraction between the walls of the heart or the different segments in each wall causes hemodynamic disturbances, which has also become the main problem of cardiac systolic dysfunction.
VVI is based on the principle of speckle tracking and uses the best pattern-matching algorithm to track and identify any small piece of myocardium or region of interest (ROI) from the previous frame to the next frame. The scattering, reflection, and interference of ultrasound energy by tissues can form so-called spots in the gray-scale sound image of echocardiography. As a marker of myocardial tissue, this can be tracked image by image throughout the cardiac cycle (15). The spots in the myocardium are randomly arranged scatterers, and their arrangement in the myocardium has a characteristic pattern. This feature is relatively stable from the previous frame to the next frame, and thus, VVI technology can track the two-dimensional ultrasound image. The trajectory of the speckle movement, its movement and deformation are described, and then the purpose of analyzing blood flow and tissue movement is achieved. Amundsen et al. (16) used the sonar micro-measurement method and the nuclear magnetic resonance method as a comparison, and confirmed that the speckle tracking technique can accurately measure myocardial strain without angle dependence. In addition, VVI tracks the intima of the common carotid artery according to the tracing point, automatically determines the center of centripetal motion, and is not affected by the swing caused by arterial pulsation, which improves the repeatability of detection (17). Moreover, VVI can automatically provide a variety of cardiac function parameters, such as systolic and diastolic ventricular volume, segmental ejection score, etc., which are not available in other echocardiographic methods (18). The time to reach peak velocity, peak strain, and strain rate of each segment, as well as the time to reach 75% and 50% strain and strain rate, etc., are of great significance for the evaluation of heart failure resynchronization therapy.
Strain value uses the ischemic myocardium to contract after contraction to evaluate myocardial asynchronous movement (19-21). This study showed that the cardiac function, systolic peak longitudinal, and radial strain were not significantly improved after 1 week compared with those before surgery, which was related to the increase of myocardial edema, cellular bleeding, and myocardial stiffness in some segments after 1 week, as well as the stunning of some myocardium caused by short-term balloon occlusion during PCI and ischemia/reperfusion injury to the myocardium. The peak systolic longitudinal and radial strain values at 1 month postoperatively increased to varying degrees compared with those before the operation, which indicated that partially stunned or hibernating myocardium was reperfused at 1 month after surgery, the blood supply of ischemic myocardium was improved, the myocardial activity of viable myocardium was gradually restored, myocardial contractility was enhanced, motor function was improved, and strain was increased accordingly. However, the strain values of some segments did not improve significantly after 1 month, which was mainly related to the severity of myocardial ischemia, the difference of myocardial tolerance to ischemia, the recovery degree, and time of myocardial function after myocardial ischemia/reperfusion. The early diagnosis and treatment can improve the prognosis effect of CHD patients to the greatest extent. The accuracy and repeatability of VVI detection can diagnose arrhythmia in CHD patients as early as possible, and make effective judgment on its type, which can provide effective basis for the clinical formulation of scientific and reasonable treatment plan and targeted treatment for patients, it is worthy of clinical promotion
Although the asynchronous contraction between the various walls of the heart or the different segments in each wall is the major problem of systolic dysfunction, left ventricular myocardial systolic dysfunction is often caused by a variety of factors. The long-term observation of patients with heart disease after surgery in this study was insufficient to compare and evaluate the contractile motion changes of damaged local myocardial wall segments and the overall left ventricular systolic function at 3, 6, and 12 months after surgery. In future research, the observation time will be extended, the sample size will be expanded, and the influence of the above factors will be excluded to further determine the value of VVI technology in the diagnosis of the asynchronous movement of left ventricular myocardial contraction before and after the treatment of CHD.
Conclusions
VVI can comprehensively and objectively evaluate left ventricular global and regional myocardial function, thereby providing a novel method for the clinical treatment of CHD as well as the evaluation of curative effect and prognosis.
Acknowledgments
Funding: None.
Footnote
Reporting Checklist: The authors have completed the STROBE reporting checklist. Available at https://dx.doi.org/10.21037/apm-21-1540
Data Sharing Statement: Available at https://dx.doi.org/10.21037/apm-21-1540
Conflicts of Interest: All authors have completed the ICMJE uniform disclosure form (available at https://dx.doi.org/10.21037/apm-21-1540). The authors have no conflicts of interest to declare.
Ethical Statement: The authors are accountable for all aspects of this work in ensuring that any questions related to the accuracy or integrity of any part of the work are appropriately investigated and resolved. This study was approved by the Ethics Committee of Affiliated Hospital of Jiangnan University (KY20132) and was conducted in accordance with the Declaration of Helsinki (as revised in 2013). All participants provided informed consent.
Open Access Statement: This is an Open Access article distributed in accordance with the Creative Commons Attribution-NonCommercial-NoDerivs 4.0 International License (CC BY-NC-ND 4.0), which permits the non-commercial replication and distribution of the article with the strict proviso that no changes or edits are made and the original work is properly cited (including links to both the formal publication through the relevant DOI and the license). See: https://creativecommons.org/licenses/by-nc-nd/4.0/.
References
- . Summary of China cardiovascular health and disease report 2019. Chinese Journal of Geriatric Research 2020;7:4-15.
- . Chinese guidelines for cardiovascular risk assessment and management. Chinese Journal of Health Management 2019;7-29.
- Armstrong PW, Gershlick AH, Goldstein P, et al. Fibrinolysis or primary PCI in ST-segment elevation myocardial infarction. N Engl J Med 2013;368:1379-87. [Crossref] [PubMed]
- Bai F, Li B, Zhu L, et al. Current situation and Prospect of cardiac rehabilitation after percutaneous coronary intervention. Chinese Journal of Clinical Health 2015;18:548-51.
- Weiwei C, Runlin G, Lisheng L, et al. Outline of the report on cardiovascular diseases in China, 2014. Eur Heart J Suppl 2016;18:F2-F11. [Crossref] [PubMed]
- Mohr FW, Morice MC, Kappetein AP, et al. Coronary artery bypass graft surgery versus percutaneous coronary intervention in patients with three-vessel disease and left main coronary disease: 5-year follow-up of the randomised, clinical SYNTAX trial. Lancet 2013;381:629-38. [Crossref] [PubMed]
- Vannan MA, Pedrizzetti G, Li P, et al. Effect of cardiac resynchronization therapy on longitudinal and circumferential left ventricular mechanics by velocity vector imaging: description and initial clinical application of a novel method using high-frame rate B-mode echocardiographic images. Echocardiography 2005;22:826-30. [Crossref] [PubMed]
- Shin SH, Hung CL, Uno H, et al. Mechanical dyssynchrony after myocardial infarction in patients with left ventricular dysfunction, heart failure, or both. Circulation 2010;121:1096-103. [Crossref] [PubMed]
- Lee PW, Zhang Q, Yip GW, et al. Left ventricular systolic and diastolic dyssynchrony in coronary artery disease with preserved ejection fraction. Clin Sci (Lond) 2009;116:521-9. [Crossref] [PubMed]
- Yang ZR, Zhou QC, Lee L, et al. Quantitative assessment of left ventricular systolic function in patients with coronary heart disease by velocity vector imaging. Echocardiography 2012;29:340-5. [Crossref] [PubMed]
- Leitman M, Lysyansky P, Sidenko S, et al. Two-dimensional strain-a novel software for real-time quantitative echocardiographic assessment of myocardial function. J Am Soc Echocardiogr 2004;17:1021-9. [Crossref] [PubMed]
- Nguyen TT, Espinoza AW, Hyler S, et al. Myocardial strain measured by epicardial transducers-comparison between velocity estimators. Ultrasound Med Biol 2021;47:1377-96. [Crossref] [PubMed]
- Gorcsan J 3rd, Kanzaki H, Bazaz R, et al. Usefulness of echocardiographic tissue synchronization imaging to predict acute response to cardiac resynchronization therapy. Am J Cardiol 2004;93:1178-81. [Crossref] [PubMed]
- Biering-Sørensen T. Cardiac time intervals by tissue Doppler imaging M-mode echocardiography: reproducibility, reference values, association with clinical characteristics and prognostic implications. Dan Med J 2016;63:B5279. [PubMed]
- Cannesson M, Tanabe M, Suffoletto MS, et al. Velocity vector imaging to quantify ventricular dyssynchrony and predict response to cardiac resynchronization therapy. Am J Cardiol 2006;98:949-53. [Crossref] [PubMed]
- Amundsen BH, Helle-Valle T, Edvardsen T, et al. Noninvasive myocardial strain measurement by speckle tracking echocardiography: validation against sonomicrometry and tagged magnetic resonance imaging. J Am Coll Cardiol 2006;47:789-93. [Crossref] [PubMed]
- Bohs LN, Geiman BJ, Anderson ME, et al. Speckle tracking for multi-dimensional flow estimation. Ultrasonics 2000;38:369-75. [Crossref] [PubMed]
- Zeng S, Jiang T, Zhou QC, et al. Time-course changes in left ventricular myocardial deformation in STZ-induced rabbits on velocity vector imaging. Cardiovasc Ultrasound 2014;12:17. [Crossref] [PubMed]
- Asanuma T, Nakatani S. Myocardial ischaemia and post-systolic shortening. Heart 2015;101:509-16. [Crossref] [PubMed]
- Ishizu T, Seo Y, Baba M, et al. Impaired subendocardial wall thickening and post-systolic shortening are signs of critical myocardial ischemia in patients with flow-limiting coronary stenosis. Circ J 2011;75:1934-41. [Crossref] [PubMed]
- Lim P, Donal E, Lafitte S, et al. Multicentre study using strain delay index for predicting response to cardiac resynchronization therapy (MUSIC study). Eur J Heart Fail 2011;13:984-91. [Crossref] [PubMed]
(English Language Editor: A. Kassem)