A novel synthetic compound shows antioxidant and anti-inflammatory activity and alleviates cognitive deficits in rats for the treatment of Alzheimer’s disease
Introduction
Alzheimer’s disease (AD) is the most common fatal neurodegenerative disease of older people, and is characterized by loss of memory, cognitive decline, and behavioral abnormalities, eventually leading to death (1). Although the pathogenesis of AD is not fully known, certain hallmarks including β-amyloid (Aβ) deposits, which form extracellular senile plaques, along with cerebral atrophy and intracellular neurofibrillary tangles (NFT) are reported to play prominent roles in the development of AD (2). In the past decades, scientists have developed many approaches to treat AD. However, there is no effective medicine that can definitively cure or delay the process of AD (3).
Aβ accumulation is widely regarded as the primary pathogenesis of AD (4). There is also considerable evidence to support the toxic role of Aβ oligomers in neuronal death (5). Recent studies reported that increased toxic Aβ oligomers induced inflammation in glial cells, as well as increased the expression and production of inflammatory mediators, such as nitric oxide (NO), interleukin-1β (IL-1β), and tumor necrosis factor α (TNF-α) (6-8). In addition, Aβ-induced oxidative stress refers to cell overoxidation leading to cellular damage, which is carried out by reactive oxygen species (ROS) (9,10). Oxidative damage could lead to mitochondrial dysfunction and cell lysis in AD (11,12). Thus, drugs that scavenge oxygen radicals and reduce the production of inflammatory mediators are speculated to prevent or treat AD to some extent (13,14).
A series of 8-hydroxyquinoline-resveratrol derivatives, which were obtained by combining resveratrol with the metal chelator clioquinol (CQ), were reported to act as antioxidants, biometal chelators, and inhibitors of Aβ aggregation. In particular, (E)-5-(4-hydroxystyryl)quinolin-8-ol (10c) (Figure 1), a multi-target-directed small molecules against AD, showed potential anti-oxidant behaviour, good metal chelating ability, inhibition of copper(II)-induced Aβ aggregation with ability to cross blood-brain barrier (15). In this paper, in order to study the pharmacological effects and possible mechanism of 10c in Alzheimer’s disease, we further evaluated the antioxidant activity and anti-inflammatory activity of 10c, and also studied the anti-AD effect of 10c in an animal model. A protocol was prepared before the study without registration. We present the following article in accordance with the ARRIVE reporting checklist (available at https://dx.doi.org/10.21037/apm-21-1983).
Methods
Culture of SH-SY5Y cells and BV-2 cells
The human neuroblastoma cell line SH-SY5Y (Laboratory Animal Service Center at Sun Yat-sen University) was cultured in a 1:1 mixture of F-12 nutrient mixture (Ham12) and Eagle’s MEM containing 15 nonessential amino acids, 10% heat-inactivated fetal bovine serum (FBS, GIBCO, Invitrogen, USA), 50 mg/µL penicillin, 50 mg/µL streptomycin, and 1 mM glutamine. Cultures were maintained in a humidified incubator at 37 °C with 5% CO2 and 95% air. For assays, SH-SY5Y cells were plated into 96-well plates (1×104 cells/well).
BV-2 microglial cells (BeNa Culture Collection, BNCC) were routinely grown in a humidified incubator at 37 °C with 5% CO2 and 95% air in Dulbecco’s modified Eagle’s medium (DMEM, GIBCO) containing 10% heat-inactivated FBS (GIBCO), 50 mg/µL penicillin, 50 mg/µL streptomycin, and 1 mM glutamine. For assays, BV-2 cells were plated into 96-well plates (4×104 cells/well).
Determination of ROS
SH-SY5Y cells were plated into 96-well plates at a density of 1×104 cells/well, and 24 h later the medium was substituted with the tested compounds and maintained for an additional 24 h at 37 °C. The cells were washed with phosphate buffer solution (PBS), then loaded with 5 µM of DCFH-DA (a fluorescent probe) in PBS and incubated for 30 min at 37 °C in 5% CO2. After removing DCFH-DA and washing, the cells were treated with 0.1 mM t-BuOOH (a compound to induce oxidative stress) in PBS for 30 min. A multifunction microplate reader (Molecular Devices, Flex Station 3) was used to measure the fluorescence intensity of the cells from each well after incubation (λex =485 nm, λem =535 nm).The antioxidant activity, expressed as a percentage of the control cells, was calculated according to the formula (Ft-Fnt)/(Ft’-Fnt) ×100, where Ft = fluorescence intensity of neurons treated with the tested compound, Ft’ = fluorescence intensity of neurons treated without the tested compound, and Fnt = fluorescence intensity of neurons untreated with t-BuOOH.
Rotenone/oligomycin A-induced death of human neuroblastoma SH-SY5Y cells
The inhibitory effect of 10c on mitochondrial-triggered endogenous oxidative stress induced by rotenone/oligomycin A was evaluated with the human neuroblastoma cell line SH-SY5Y, while the mixture of rotenone and oligomycin A acted as the toxic insult (16,17). SH-SY5Y cells were exposed to 30 µM rotenone plus 10 µM oligomycin A for 24 h in the presence or absence of compound 10c and then measured with the MTT reduction method.
Measurement of NO production
The NO concentration was determined according to the Griess reaction (18,19). BV-2 cells were plated into 96-well plates at a density of 4×104 cells/well, and 24 h later cells were treated with 1.0 µg/mL lipopolysaccharide (LPS) in the presence or absence of compound 10c for 24 h. Then, 50 µL of culture supernatant was mixed with an equal volume of freshly prepared Griess reagent (1% sulfanilic acid in 5% phosphoric acid, 0.1% N-1-naphthylethylenediamine) at room temperature in the dark. After 10 min, the absorbance at 540 nm was determined by a multifunction microplate reader (molecular devices, flex station 3). Nitrite concentration was calculated with sodium nitrite as a standard. Inhibition (%) = [1− (ALPS+sample − Auntreated)/(ALPS −Auntreated)] ×100. These experiments were measured in quintuplicate, and the IC50 values were calculated using GraphPad Prism version 5.0. Quercetin was used as a positive control.
Rat IL-1β ELISA method
The quantification of IL-1β was performed using the IL-1β immunoassay kit provided by R&D Systems, which is a 4.5 h solid-phase ELISA designed to measure rat IL-1β levels. A rat IL-1β monoclonal antibody was pre-coated onto a microplate (20). The supernatant of BV-2 cells was treated with 1.0 µg/mL LPS in the presence or absence of compound 10c for 24 h. Then, standards and samples were pipetted into the wells and any rat IL-1β present was bound by the immobilized antibody. After washing to remove any unbound substances, an enzyme-linked polyclonal antibody specific for rat IL-1β was added to the wells. Following washing away any unbound antibody-enzyme reagent, a substrate solution was added to the wells, and the intensity of the color produced, which was measured after the color development was stopped, was proportional to the amount of rat IL-1β bound in the initial step. The experiments were measured in quintuplicate and analyzed using GraphPad Prism version 5.0.
Animal experiments
Chemicals and reagents
Aβ
Animals
Forty Wistar male rats weighing 250–300 g were purchased from the laboratory animal center of Sun Yat-sen University (Guangzhou, China). The rats were randomly divided into 4 groups: the sham group, the model group, the CQ group (21 mg/kg), and the 10c (35 mg/kg) group, with 10 rats in each group. Rats were provided with sterile food and water according to institutional guidelines, and raised on a 12 h light/dark cycle (light from 07:00 to 19:00) at a temperature of 20–22 °C and relative humidity of 60–70%. Rats were fasted overnight and allowed free access to water before each experiment. Animal experiments were performed under a project license (No. 20175000102) granted by the ethics committee of Sun Yat-sen University, in compliance with Chinese national guidelines for the care and use of animals.
Intrahippocampal injection of Aβ25–35
Stereotaxic surgery was performed under chloral hydrate (7%, i.p.) anesthesia. Aβ
Morris water maze test
The Morris water maze test was performed on day 29 for 6 days. Firstly, rats received visible-platform training for 5 consecutive days (4 trials/day), with the platform placed in a different location every time. Then, rats were allowed to swim to a flag-mounted platform above the water. The rat was permitted to stay on the platform for 15 s, and if it located the platform within 120 s, the seeking time (namely the escape latency) and the path it took were recorded. Otherwise, the rat was directed onto the platform and the searching time was recorded as 120 s. To assess memory retention, a spatial probe trial was performed 24 h after the last visible-platform trial. The platform was removed from the pool and the rats had 120 s to swim to locate the platform. The number of rats crossing the former platform and the time spent in the target quadrant were used to indicate the level of memory retention that occurred after learning.
Statistical analysis
Data were presented as mean ± standard deviation, and results were compared using a one-way ANOVA method with a Fisher LSD post-hoc comparison. A significant difference was defined as P<0.05, and a highly significant difference was defined as P<0.01.
Results
Intracellular antioxidant activity against exogenous ROS
Oxidative stress-induced damage has been proven to be a significant event in AD patients, which is associated with damage to DNA, protein oxidation, and lipid peroxidation. Therefore, the scavenging of ROS by some drugs may be an effective approach for the treatment of AD.
As shown in Figure 2, ROS production of SH-SY5Y cells treated with trolox at 20 µM was 86.15% of the control, indicating that trolox had moderate antioxidant activity. In contrast, compound 10c exhibited far more potent antioxidant activity than trolox, resveratrol, and CQ (ROS production-10c: 26.23% of the control at 1.5 µM, 35.13% of the control at 1 µM, and 60.63% of the control at 0.5 µM; resveratrol: 82.17% of the control at 2.5 µM; CQ: 78.52% of the control at 10 µM). The strong antioxidant activity of compound 10c further supports it as a promising compound for the treatment of AD.
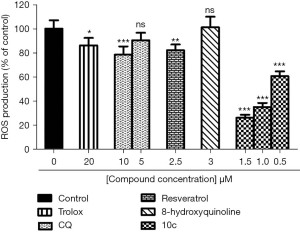
Neuroprotective effect of 10c
Rotenone and oligomycin A disrupt ATP synthesis by blocking complexes I and V, respectively, of the mitochondrial electron transport chain (18). Rotenone/oligomycin A is an efficient experimental method to evaluate endogenous mitochondria-triggered oxidative stress. As shown in Figure 3, with respect to control, cells treated only with Rotenone/oligomycin (R/O) showed a viability of 35.45%, while 10c could effectively improve cell viability from 1 to 20 µM (cell viability-10c: 41.09% of the control at 1 µM, 51.76% of the control at 2 µM, 71.58% of the control at 5 µM, 72.54% of the control at 10 µM, 76.58% of the control at 20 µM). Thus, 10c shows good neuroprotective effects as an endogenous antioxidant in neuroblastoma cells.
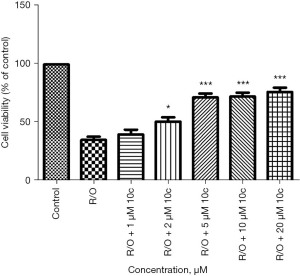
Compound 10c suppresses NO level in LPS-induced BV-2 microglial cells
NO, a proinflammatory mediator, is overexpressed in the neuroinflammatory process (21,22). NO and superoxide cause peroxynitrite formation, which leads to oxidative stress causing cell signaling pathway disruption, cellular dysfunction, and brain cell death (23,24). As shown in Figure 4, the positive control, quercetin, exhibited moderate activity with an IC50 value of 10.00 µM. Compound 10c demonstrated good inhibitory activity against NO production with an IC50 value of 3.10 µM, suggesting that it may be an efficient agent for the treatment of neurodegenerative diseases with neuroinflammatory involvement.
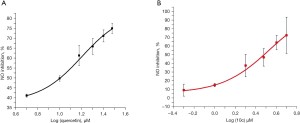
Compound 10c reduces IL-1β production in vitro
Neuroinflammation plays an important role in the pathogenesis of AD, and the proinflammatory factor IL-1β plays a central role in immune and inflammatory responses (25,26). As shown in Figure 5, 10c effectively reduced the release of IL-1β from 0 to 20 µM, and it was a potential inhibitor of IL-1β production.
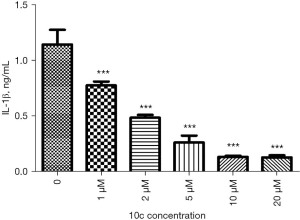
Protective effects of compound 10c on Aβ25–35-induced learning and memory dysfunction in rats
In order to evaluate the therapeutic effect of 10c in an AD animal model, we performed the Morris water maze test to assess Aβ25–35-induced learning and memory dysfunction in rats treated with compound 10c (27). CQ was used as the reference compound. As shown in Figure 6A, the number of model group rats who crossed the former platform was 1.9±1.2 in the spatial probe trial, which was much less than the sham group (7.1±4.4). These results suggest that intrahippocampal injection of Aβ25–35 could induce memory and spatial learning deficiency in rats. However, the numbers of rats who crossed the former platform in both the 10c (5.7±1.6) and CQ (5.1±1.7) treatment groups were significantly increased (P<0.05) compared to the model group. Meanwhile, the results of the time spent in the target quadrant were similar to the numbers of rats crossing the former platform. Compared with the sham group (44.8±11.1 s), the vehicle-treated model group (32.7±4.1 s) showed a significant reduction (P<0.05) in the time rats spent in the target quadrant (Figure 6B). Both 10c (43.8±5.5 s) and CQ (44.1±6.6 s) treatment could significantly prolong the time rats spent in the target quadrant compared to the vehicle-treated model group. These results demonstrated that 10c could alleviate the learning and memory dysfunction of rats induced by Aβ25–35 to a certain extent. Compared with well-established transgenic mouse model, the animal model we used in this study may just can mimic the early stage of Alzheimer’s disease. The long-term evaluation of 10c in AD transgenic mouse model would be carried out in our subsequent work.
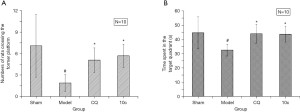
Conclusions
Based on previous research, the 8-hydroxyquinoline-resveratrol derivative 10c was studied for its intracellular antioxidative activities, anti-inflammatory activity, and protective effects on Aβ25–35-induced learning and memory dysfunction in rats. In summary, 10c showed good activity in inhibiting exogenous and endogenous ROS, and could effectively inhibit the release of proinflammatory mediators such as NO and IL-1β. Importantly, in vivo tests indicated that 10c has a good protective effect on the Aβ25–35-induced learning and memory dysfunction of rats. Altogether, compound 10c exhibited multi-funtional anti-AD activities, further research will focus on the development of compound 10c as a promising new drug for treatment of Alzheimer’s disease.
Acknowledgments
Funding: None.
Footnote
Reporting Checklist: The authors have completed the ARRIVE reporting checklist. Available at https://dx.doi.org/10.21037/apm-21-1983
Data Sharing Statement: Available at https://dx.doi.org/10.21037/apm-21-1983
Conflicts of Interest: All authors have completed the ICMJE uniform disclosure form (available at https://dx.doi.org/10.21037/apm-21-1983). The authors have no conflicts of interest to declare.
Ethical Statement: The authors are accountable for all aspects of the work in ensuring that questions related to the accuracy or integrity of any part of the work are appropriately investigated and resolved. Animal experiments were performed under a project license (No. 20175000102) granted by the ethics committee of Sun Yat-sen University, in compliance with Chinese national guidelines for the care and use of animals.
Open Access Statement: This is an Open Access article distributed in accordance with the Creative Commons Attribution-NonCommercial-NoDerivs 4.0 International License (CC BY-NC-ND 4.0), which permits the non-commercial replication and distribution of the article with the strict proviso that no changes or edits are made and the original work is properly cited (including links to both the formal publication through the relevant DOI and the license). See: https://creativecommons.org/licenses/by-nc-nd/4.0/.
References
- Kepp KP. Bioinorganic chemistry of Alzheimer's disease. Chem Rev 2012;112:5193-239. [Crossref] [PubMed]
- Zhou WW, Lu S, Su YJ, et al. Decreasing oxidative stress and neuroinflammation with a multifunctional peptide rescues memory deficits in mice with Alzheimer disease. Free Radic Biol Med 2014;74:50-63. [Crossref] [PubMed]
- Massa F, Meli R, Morbelli S, et al. Serum neurofilament light chain rate of change in Alzheimer’s disease: potentials applications and notes of caution. Ann Transl Med 2019;7:S133. [Crossref] [PubMed]
- Bolognesi ML, Matera R, Minarini A, et al. Alzheimer's disease: new approaches to drug discovery. Curr Opin Chem Biol 2009;13:303-8. [Crossref] [PubMed]
- Sachdeva AK, Chopra K. Lycopene abrogates Aβ(1-42)-mediated neuroinflammatory cascade in an experimental model of Alzheimer's disease. J Nutr Biochem 2015;26:736-44. [Crossref] [PubMed]
- Ferretti MT, Bruno MA, Ducatenzeiler A, et al. Intracellular Aβ-oligomers and early inflammation in a model of Alzheimer's disease. Neurobiol Aging 2012;33:1329-42. [Crossref] [PubMed]
- Nazem A, Sankowski R, Bacher M, et al. Rodent models of neuroinflammation for Alzheimer's disease. J Neuroinflammation 2015;12:74. [Crossref] [PubMed]
- Bungart BL, Dong L, Sobek D, et al. Nanoparticle-emitted light attenuates amyloid-β-induced superoxide and inflammation in astrocytes. Nanomedicine 2014;10:15-7. [Crossref] [PubMed]
- Behl C, Moosmann B. Antioxidant neuroprotection in Alzheimer's disease as preventive and therapeutic approach. Free Radic Biol Med 2002;33:182-91. [Crossref] [PubMed]
- Finkel T, Holbrook NJ. Oxidants, oxidative stress and the biology of ageing. Nature 2000;408:239-47. [Crossref] [PubMed]
- Sharma P, Jha AB, Dubey RS, et al. Reactive Oxygen Species, Oxidative Damage, and Antioxidative Defense Mechanism in Plants under Stressful Conditions. Journal of Botany 2012;2012:1-26. [Crossref]
- Praticò D. Oxidative stress hypothesis in Alzheimer's disease: a reappraisal. Trends Pharmacol Sci 2008;29:609-15. [Crossref] [PubMed]
- Lee HP, Casadesus G, Zhu X, et al. All-trans retinoic acid as a novel therapeutic strategy for Alzheimer's disease. Expert Rev Neurother 2009;9:1615-21. [Crossref] [PubMed]
- Wang X, Wang W, Li L, et al. Oxidative stress and mitochondrial dysfunction in Alzheimer's disease. Biochim Biophys Acta 2014;1842:1240-7. [Crossref] [PubMed]
- Mao F, Yan J, Li J, et al. New multi-target-directed small molecules against Alzheimer's disease: a combination of resveratrol and clioquinol. Org Biomol Chem 2014;12:5936-44. [Crossref] [PubMed]
- de Los Ríos C, Egea J, Marco-Contelles J, et al. Synthesis, inhibitory activity of cholinesterases, and neuroprotective profile of novel 1,8-naphthyridine derivatives. J Med Chem 2010;53:5129-43. [Crossref] [PubMed]
- Romero A, Egea J, González-Muñoz GC, et al. ITH12410/SC058: a new neuroprotective compound with potential in the treatment of Alzheimer's disease. ACS Chem Neurosci 2014;5:770-5. [Crossref] [PubMed]
- Egea J, Rosa AO, Cuadrado A, et al. Nicotinic receptor activation by epibatidine induces heme oxygenase-1 and protects chromaffin cells against oxidative stress. J Neurochem 2007;102:1842-52. [Crossref] [PubMed]
- Li J, Zeng KW, Shi SP, et al. Anti-neuroinflammatory constituents from Polygala tricornis Gagnep. Fitoterapia 2012;83:896-900. [Crossref] [PubMed]
- Allan SM, Tyrrell PJ, Rothwell NJ. Interleukin-1 and neuronal injury. Nat Rev Immunol 2005;5:629-40. [Crossref] [PubMed]
- Liu B, Hong JS. Role of microglia in inflammation-mediated neurodegenerative diseases: mechanisms and strategies for therapeutic intervention. J Pharmacol Exp Ther 2003;304:1-7. [Crossref] [PubMed]
- Robinson MA, Baumgardner JE, Otto CM. Oxygen-dependent regulation of nitric oxide production by inducible nitric oxide synthase. Free Radic Biol Med 2011;51:1952-65. [Crossref] [PubMed]
- Dong Z, Gu Q, Cheng B, et al. Natural nitric oxide (NO) inhibitors from Aristolochia mollissima. RSC Adv 2014;4:55036-43. [Crossref]
- Carret-Rebillat AS, Pace C, Gourmaud S, et al. Neuroinflammation and Aβ accumulation linked to systemic inflammation are decreased by genetic PKR down-regulation. Sci Rep 2015;5:8489. [Crossref] [PubMed]
- Lippai D, Bala S, Petrasek J, et al. Alcohol-induced IL-1β in the brain is mediated by NLRP3/ASC inflammasome activation that amplifies neuroinflammation. J Leukoc Biol 2013;94:171-82. [Crossref] [PubMed]
- Sims JE, Smith DE. The IL-1 family: regulators of immunity. Nat Rev Immunol 2010;10:89-102. [Crossref] [PubMed]
- Wang Z, Wang Y, Wang B, et al. Design, Synthesis, and Evaluation of Orally Available Clioquinol-Moracin M Hybrids as Multitarget-Directed Ligands for Cognitive Improvement in a Rat Model of Neurodegeneration in Alzheimer's Disease. J Med Chem 2015;58:8616-37. [Crossref] [PubMed]
(English Language Editor: C. Betlazar-Maseh)