Programmed cell death 1 inhibitors with or without concurrent brain radiotherapy for lung cancer patients with brain metastases
Introduction
Approximately 50–65% of lung cancer patients have brain metastases (BMs) during the course of their illness (1). The presence of BM is an indication of a poor prognosis (2), especially for untreated BM patients, who have a median survival time of only 1–2 months (3).
Immune checkpoint inhibitors (ICIs), such as programmed death receptor 1 (PD-1) inhibitors, improve survival in patients with advanced lung cancer (4-7). However, patients with unstable BMs have been excluded in most pivotal immunotherapy clinical trials. An analysis of clinical trials for advanced non-small cell lung cancer (NSCLC) showed only 27.7% of studies allowed enrolment of patients with untreated asymptomatic BMs, and only 3.7% included patients with symptomatic or progressive BMs (8,9). To determine the activity of PD-1 blockade in the central nervous system (CNS), a phase II trial enrolled 42 NSCLC patients with untreated BMs receiving pembrolizumab. The results showed that 29.7% of patients with a PD-L1 expression of at least 1% achieved an intracranial response (10).
Although the CNS was once considered an immune-privileged site, BMs can exist in an inflammatory microenvironment and contain pre-existing tumor-infiltrating lymphocytes (11,12). T cells within the tumor microenvironment are responsible for the activity of PD-1 inhibitors in treating intracranial lesions (11,12). Several preclinical reports have noted the synergistic effects of radiotherapy and anti-PD-1 immunotherapy on local control and the abscopal response (13,14). Preclinical studies have suggested radiotherapy enhances many of the steps needed for generating antigen-specific immune responses, including dendritic cell activation, antigen cross-presentation, inflammatory tumor-cell death, and cytotoxic T-cell activation and proliferation (13). This suggests radiotherapy and immunotherapy may be synergistic. Clinical data focusing on the synergistic effect of PD-1 inhibitors and brain radiotherapy for BMs in lung cancer is limited. This study evaluated the efficacy of concurrent PD-1 inhibitors with brain radiotherapy for BMs in patients with lung cancer in clinical practice. We present the following article in accordance with the STROBE reporting checklist (available at https://dx.doi.org/10.21037/apm-21-2334).
Methods
Patients
This was a retrospective observational study, and patient information was collected from the hospital database. There was no direct intervention in patients’ treatment. From August 2018 to April 2020, lung cancer patients with BMs receiving PD-1 inhibitors were identified at the Cancer Center, Union Hospital, Tongji Medical College, Huazhong University of Science and Technology, Wuhan, Hubei, China. Sixty-eight patients were identified, with eight excluded, leaving 60 patients for analysis. The inclusion criteria for this study were as follows: (I) histologically or cytologically confirmed lung cancer, including lung adenocarcinoma, squamous cell lung carcinoma, and small cell lung cancer (SCLC); (II) BMs confirmed by computerized tomography or magnetic resonance imaging, including treated or newly diagnosed, symptomatic or asymptomatic; (III) the patients had received immediate or delayed PD-1 inhibitor immunotherapy after BM. The key exclusion criteria included the following: (I) a previous malignancy other than lung cancer; (II) leptomeningeal metastases; (III) receipt of immunotherapy before the diagnosis of BM; (IV) absence of detailed treatment information. All procedures performed in this study involving human participants were in accordance with the Declaration of Helsinki (as revised in 2013). The study was approved by the Ethics Committee of Union Hospital, Tongji Medical College, Huazhong University of Science and Technology. Patient data were retrieved from the hospital medical record system, so an informed consent form was not required. The patients’ personal data has been secured.
Treatment
Baseline assessments included complete blood count, serum tumor marker, hepatic function, renal function electrolytes, computed tomography (CT) scanning, and magnetic resonance imaging (MRI). PD-1 inhibitors included nivolumab, pembrolizumab, or other PD-1 inhibitors approved in China. The PD-1 inhibitors were administered until disease progression or unacceptable toxicity. Conventional chemotherapy was allowed at the physicians’ discretion. Brain radiotherapy used in this study included stereotactic radiosurgery/stereotactic radiotherapy (SRS/SRT) and whole brain radiotherapy (WBRT). SRS/SRT was administered with CyberKnife 10.5 (American Accuray Company CyberKnife VSI). Prescription doses were determined by the treating physicians. SRS/SRT was typically delivered with 16–36 gray (Gy) by 1–4 fractions (F). WBRT was generally delivered with 30 Gy/10 F and 36 Gy/12 F. The tumor response was evaluated using the Response Evaluation Criteria in Solid Tumors version 1.1 (RECIST).
Statistical analysis
The primary endpoints of our analysis were intracranial progression-free survival (iPFS), extracranial progression-free survival (PFS), and OS. iPFS was defined as the time from the first day of immunotherapy to the date of intracranial disease progression, recurrence, or death due to any cause. PFS was defined as the time from the first day of immunotherapy to the date of extracranial disease progression, recurrence, or death due to any cause. OS was measured from the first day of immunotherapy to the date of death or the last follow-up. The iPFS, PFS, and OS were calculated with the Kaplan-Meier method, and comparisons between groups were analyzed using log-rank tests with 95% confidence intervals (CIs). All tests of statistical significance were 2-sided, and a P value <0.05 was considered statistically significant. Multivariate analyses using the Cox proportional hazards model were performed, and variables with P<0.05 in the univariate analysis were included. All analyses were performed using SPSS Statistics (version 24.0; IBM Corporation, Armonk, NY, USA).
Results
Patient characteristics
The 60 patients had a median age of 60 years (range, 31–76 years), and 46 patients (76.7%) were male. Forty-six patients had lung adenocarcinoma, nine patients had SCLC, and three patients had other histological types. Most patients (88.3%) had an Eastern Cooperative Oncology Group (ECOG) performance status (PS) score of 0–1. Thirty-eight (63.3%) and 22 (36.7%) patients were ever smokers and never smokers, respectively. Ten patients (16.7%) had epidermal growth factor receptor (EGFR) positive mutations, and nine of these patients previously received EGFR-TKIs and progressed before enrolment in this study. Patients with synchronous and metachronous BMs accounted for 63.3% and 36.7%, respectively. The proportion of symptomatic BMs was 55%. Forty-four out of 60 patients had extracranial metastasis. The majority of patients were in the diagnosis-specific Graded Prognostic Assessment (DS-GPA) (15) class 0–2 (70%), whereas 18 patients (30%) were in class 2.5–4.0. The baseline characteristics of patients in the concurrent group, non-concurrent group, and PD-1 inhibitors alone group are shown in Table 1. Except for intracranial symptoms and lines of immunotherapy, other clinical features were well balanced between the groups.
Table 1
Characteristics | Concurrent group, n=21 (%) | Non-concurrent group, n=20 (%) | PD-1 inhibitors alone group, n=19 (%) | P |
---|---|---|---|---|
Sex | 1.000 | |||
Male | 16 (76.2) | 15 (75.0) | 15 (78.9) | |
Female | 5 (23.8) | 5 (25.0) | 4 (21.1) | |
Age (y) | 0.207 | |||
>60 | 10 (47.6) | 6 (30.0) | 11 (57.9) | |
≤60 | 11 (52.4) | 14 (70.0) | 8 (42.1) | |
Smoking status | 0.833 | |||
Ever smoker | 14 (66.7) | 13 (65.0) | 11 (57.9) | |
Never smoker | 7 (33.3) | 7 (35.0) | 8 (42.1) | |
ECOG score | 0.234 | |||
0–1 | 20 (95.2) | 18 (90.0) | 15 (78.9) | |
≥2 | 1 (4.8) | 2 (10.0) | 4 (21.1) | |
Histological type | 0.863 | |||
Adenocarcinoma | 17 (81.0) | 15 (75.0) | 14 (73.7) | |
Others | 4 (19.0) | 5 (25.0) | 5 (26.3) | |
EGFR mutation status | 0.519 | |||
Wild type | 12 (57.1) | 14 (70.0) | 12 (63.2) | |
Positive mutation | 6 (28.6) | 2 (10.0) | 2 (10.5) | |
Not examined | 3 (14.3) | 4 (20.0) | 5 (26.3) | |
Number of brain metastases | 0.274 | |||
≤3 | 13 (61.9) | 11 (55.0) | 15 (78.9) | |
>3 | 8 (38.1) | 9 (45.0) | 4 (21.1) | |
Brain metastases time | 0.204 | |||
Synchronous | 11 (52.4) | 12 (60.0) | 15 (78.9) | |
Metachronous | 10 (47.6) | 8 (40.0) | 4 (21.1) | |
Intracranial symptoms | 0.001 | |||
Symptomatic | 18 (85.7) | 10 (50.0) | 5 (26.3) | |
Asymptomatic | 3 (14.3) | 10 (50.0) | 14 (73.7) | |
Extracranial metastasis | 0.839 | |||
Present | 16 (76.2) | 15 (75.0) | 13 (68.4) | |
Absent | 5 (23.8) | 5 (25.0) | 6 (31.6) | |
DS-GPA | 0.725 | |||
0–2 | 16 (76.2) | 13 (65.0) | 13 (68.4) | |
2.5–4 | 5 (23.8) | 7 (35.0) | 6 (31.6) | |
Immunotherapy | 0.010 | |||
1st line | 9 (42.9) | 4 (20.0) | 13 (68.4) | |
≥2nd line | 12 (57.1) | 16 (80.0) | 6 (31.6) | |
Chemotherapy | 0.192 | |||
Yes | 10 (47.6) | 10 (50.0) | 14 (73.7) | |
No | 11 (52.4) | 10 (50.0) | 5 (26.3) | |
Brain radiotherapy | ||||
SRS/SRT | 12 (57.1) | 11 (55.0) | — | 0.890 |
WBRT | 9 (42.9) | 9 (45.0) | — |
DS-GPA, diagnosis-specific Graded Prognostic Assessment; ECOG, Eastern Cooperative Oncology Group; EGFR, epidermal growth factor receptor; PD-1, programmed cell death 1; SRS, stereotactic radiosurgery; SRT, stereotactic radiotherapy; WBRT, whole brain radiotherapy.
Treatment outcomes
All 60 lung cancer patients with BMs received PD-1 inhibitors. PD-1 inhibitors were delivered as first-line therapy in 26 patients (43.3%). Among the 60 patients, 21 patients received PD-1 inhibitors and concurrent brain radiotherapy (concurrent group), 20 patients were treated with PD-1 inhibitors and non-concurrent brain radiotherapy (non-concurrent group), and 19 patients were treated with PD-1 inhibitors alone (PD-1 inhibitors alone group). In the concurrent group, the median interval between PD-1 inhibitors and brain radiotherapy was 9 days (range, 0–37 days). In the concurrent group, non-concurrent group, and PD-1 inhibitors alone group, the intracranial objective response rates (iORR) were 61.1%, 29.4%, and 25.0%, respectively, and the intracranial disease control rates (iDCR) were 83.3%, 58.8%, and 56.3%, respectively (Table 2).
Table 2
Variable | Concurrent group (n=21) | Non-concurrent group (n=20) | PD-1 inhibitors alone group (n=19) |
---|---|---|---|
Intracranial efficacy | |||
iORR | 61.1% | 29.4% | 25.0% |
iDCR | 83.3% | 58.8% | 56.3% |
Survival | |||
median iPFS (months) | 9.8 | 5.7 | 4.8 |
median PFS (months) | 9.2 | 5.7 | 4.6 |
median OS (months) | Not reached | 12.1 | 6.9 |
iORR, intracranial objective response rate; iDCR, intracranial disease control rate; iPFS, intracranial progression-free survival; OS, overall survival; PFS, progression-free survival; PD-1, programmed cell death 1.
The median iPFS was significantly longer in the concurrent group than in the non-concurrent and PD-1 inhibitors alone groups (9.8 vs. 5.7 vs. 4.8 months, P=0.039, respectively). The median PFS of the three groups was 9.2, 5.7, and 4.6 months (P=0.347), respectively, and the median OS was not reached, 12.1, and 6.9 months (P=0.206), respectively (Figure 1). Subgroup analysis showed that first-line immunotherapy showed a trend toward a higher iPFS (7.8 vs. 4.3 months, P=0.079), PFS (10.9 vs. 5.7 months, P=0.051), and OS (13.1 vs. 8.0 months, P=0.097) compared with ≥ second-line immunotherapy (Figure 2).
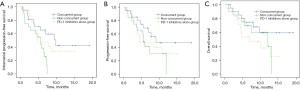
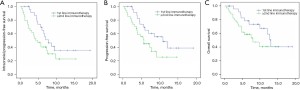
Prognostic factors
Clinical features were evaluated to determine their prognostic significance on survival. Univariate analysis showed that lack of concurrent brain radiotherapy and never smoking were adverse prognostic factors for iPFS in all 60 patients (Table 3). Adverse prognostic factors for PFS included the female sex, never smoking, and an ECOG score ≥2. Never smoking and an ECOG score ≥2 were also adverse prognostic factors for OS. Multivariate analysis revealed that lack of concurrent brain radiotherapy was independently associated with a shorter iPFS (Table S1). Never smoking was an independent adverse prognostic factor for iPFS (HR, 1.968, 95% CI, 1.023–3.785, P=0.043) and OS (HR, 2.293, 95% CI, 1.049–5.011, P=0.038). An ECOG score ≥2 was an independent adverse prognostic factor for iPFS (HR, 2.650, 95% CI, 1.049–6.696, P=0.039) and PFS (HR, 2.437, 95% CI, 1.076–5.520, P=0.033). We also explored the survival of never smokers and ever smokers. The median iPFS (7.0 vs. 3.9 months, P=0.015), PFS (12.1 vs. 4.0 months, P=0.009), and OS (13.1 vs. 7.5 months, P=0.029) were all significantly longer in ever smokers compared with never smokers (Figure 3).
Table 3
Factors | iPFS | PFS | OS | |||||
---|---|---|---|---|---|---|---|---|
HR | P | HR | P | HR | P | |||
Sex (female |
1.878 | 0.082 | 2.283 | 0.028 | 1.723 | 0.206 | ||
Age ≤60 ( |
1.070 | 0.841 | 1.106 | 0.778 | 0.735 | 0.439 | ||
Never smoker ( |
2.184 | 0.018 | 2.415 | 0.012 | 2.323 | 0.034 | ||
ECOG score ≥2 ( |
2.216 | 0.050 | 2.360 | 0.037 | 2.431 | 0.046 | ||
Adenocarcinoma ( |
0.832 | 0.633 | 0.720 | 0.406 | 0.603 | 0.237 | ||
EGFR wild type ( |
0.571 | 0.180 | 0.451 | 0.069 | 0.396 | 0.059 | ||
Brain metastases >3 ( |
1.204 | 0.580 | 1.113 | 0.764 | 0.929 | 0.855 | ||
Metachronous BM ( |
1.298 | 0.437 | 1.844 | 0.083 | 1.717 | 0.168 | ||
Asymptomatic BM ( |
1.357 | 0.357 | 1.283 | 0.476 | 1.164 | 0.694 | ||
Extracranial metastasis ( |
1.784 | 0.171 | 2.215 | 0.083 | 2.592 | 0.082 | ||
DS-GPA (0–2 |
1.949 | 0.113 | 2.253 | 0.075 | 2.208 | 0.115 | ||
Immunotherapy (1st line |
0.555 | 0.084 | 0.492 | 0.056 | 0.507 | 0.103 | ||
Chemotherapy (yes |
1.176 | 0.628 | 1.382 | 0.371 | 1.155 | 0.714 | ||
Brain radiotherapy (SRS/SRT |
1.132 | 0.765 | 0.779 | 0.571 | 0.887 | 0.818 | ||
Concurrent brain radiotherapy | 1 (ref) | 0.049 | 1 (ref) | 0.358 | 1 (ref) | 0.221 | ||
|
2.780 | 1.851 | 1.619 | |||||
|
1.483 | 1.583 | 2.325 |
BM, brain metastases; DS-GPA, diagnosis-specific Graded Prognostic Assessment; ECOG, Eastern Cooperative Oncology Group; EGFR, epidermal growth factor receptor; iPFS, intracranial progression-free survival; OS, overall survival; PFS, progression-free survival; PD-1, programmed cell death 1; RT, radiotherapy.
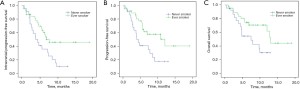
Discussion
Although PD-1 inhibitors are being studied extensively in various cancers with encouraging outcomes, the efficacy of immunotherapy and radiotherapy for BMs is still unconfirmed. This study evaluated the outcomes of 60 lung cancer patients receiving PD-1 inhibitors with or without brain radiotherapy. PD-1 inhibitors combined with concurrent brain radiotherapy resulted in a significantly longer iPFS compared to non-concurrent brain radiotherapy and PD-1 inhibitors immunotherapy alone. The PFS and OS were also longer in the concurrent group, though the difference did not reach statistical significance.
Historically, most therapeutic approaches have provided limited clinical benefit for CNS metastases patients, highlighting the need to explore the intracranial efficacy of novel therapeutics. Pembrolizumab demonstrated activity in BMs of NSCLC and melanoma patients in a phase II trial (10,16). Results indicated that 11 (29.7%) NSCLC patients with a PD-L1 expression ≥1% and 6 (26%) melanoma patients had a BM response (16). But there were no responses in NSCLC patients with PD-L1 less than 1% or unevaluable (10). Nivolumab is also active in NSCLC patients with BMs, and the intracerebral activity is similar to extracerebral efficacy with iOOR ranging from 9–28.1%, a median iPFS of 2.2–3.9 months, and a median OS of 7.5–14.8 months (17-19). Exploratory analyses of the phase III OAK study indicated atezolizumab showed a trend toward OS benefit and led to a prolonged time to radiographic identification of new symptomatic brain lesions compared with docetaxel in patients who had a history of asymptomatic, treated BMs (20).
Several retrospective studies have described the synergistic effects on local and distant tumor control when radiotherapy was combined with immune checkpoint inhibitors (ICIs). Stereotactic radiosurgery (SRS) with concurrent ICIs demonstrated a superior best objective lesion-specific response (BOR), overall objective response (OOR), and response durability than lesions treated with SRS and delayed ICIs (21). The concurrent use of ICIs and SRS to treat NSCLC patients with BMs achieved more rapid BM regression. Patients receiving concurrent ICIs showed increased rates of CNS complete response for BMs treated with SRS. There was no increased rate of radiation necrosis or intratumoral hemorrhage in the patients receiving concurrent ICIs (22), but there was no statistically significant difference in OS or iPFS between concurrent ICI and SRS alone groups in this study (22). The ICIs in these two studies included anti-CTLA4, anti-PD-1, and anti-PD-L1 (21,22). In addition, different cancers such as lung cancer, melanoma, renal cancer, and breast cancer were included for analysis (21), which might have created heterogeneity within the treatment cohort.
A retrospective study enrolled a total of 17 NSCLC patients receiving SRT and anti-PD-1/PD-L1 therapy with nivolumab or durvalumab for the treatment of BMs. The 6-month rate of distant brain control following SRT during or prior to anti-PD-1/PD-L1 therapy was 57% compared to 0% for patients who received anti-PD-1/PD-L1 therapy before SRT (23). Chen et al. (12) retrospectively analyzed the efficacy of concurrent ICI and SRS/SRT for BMs in NSCLC, melanoma, and renal cell carcinoma. ICIs used in this study included anti-CTLA4 (ipilimumab) and anti-PD-1 (nivolumab and pembrolizumab). Concurrent SRS/SRT and ICI treatment was associated with an improved OS compared to SRS/SRT only and non-concurrent SRS/SRT and ICI, with a median OS of 24.7, 12.9, and 14.5 months, respectively (12). Another study also showed that 37 lung patients treated with concurrent SRS and PD-1 pathway inhibitors had longer OS and reduced rates of distant brain failure (DBF) as compared with patients treated with SRS prior to or after PD-1 pathway inhibitors (24). A secondary analysis of the KEYNOTE-001 phase I trial showed that previous extracranial or thoracic radiotherapy before pembrolizumab in patients with advanced NSCLC resulted in a longer PFS and OS than that in patients who did not have previous radiotherapy (25). However, another study suggests that extracranial or prolonged courses of radiotherapy increase the risk of severe lymphopenia, which is associated with poorer survival in patients treated with ICIs (26). The present study was restricted to lung cancer, and all 60 patients were treated with PD-1 inhibitors with or without brain radiotherapy. Our results showed that PD-1 inhibitors combined with concurrent brain radiotherapy achieved a significantly longer iPFS than non-concurrent brain radiotherapy or PD-1 inhibitor immunotherapy alone. There was also a trend toward a longer PFS and OS in the concurrent group.
Tumor PD-L1 expression is associated with an increased likelihood of an NSCLC response to ICIs. Téglási (27) observed that there was no or only limited concordance of the proportion of PD-1 or PD-L1 positive tumor-associated immune cells between the paired primary lung adenocarcinoma and BM samples, which suggested that BMs developed their own immune environment. However, a strong correlation of PD-L1 positive tumor cells between primary lung adenocarcinoma cases and their corresponding BMs was found. The result suggested PD-L1 positivity in the primary tumor could serve as a therapeutic criterion for BMs (27). In addition, increased density or number of CD3+ and CD8+ tumor- infiltrating lymphocytes in brain tumor specimens is correlated with improved survival (8). Other putative predictive biomarkers for PD-1/PD-L1 checkpoint inhibitors include smoking history, tumor mutational burden (TMB), oncogenic driver status, and tumor histology (28,29). A multicenter, real-world, retrospective cohort study demonstrated that a smoking history might be the most important predictor of benefit from anti-PD-1/PD-L1 monotherapy in patients with oncogene-driven NSCLC (30). This result is consistent with our study. Tobacco smoke induces PD-L1 expression on lung epithelial cells, so smokers have a higher PD-L1 expression than nonsmokers. The aryl hydrocarbon receptor mediates tobacco-induced PD-L1 and predicts the response to immunotherapy (31).
This study is limited by the retrospective analysis and small sample size. In addition, PD-L1 status was not available for many patients, so we could not evaluate whether there was a correlation between PD-L1 expression and response to PD-1 inhibitors. However, data focusing on the synergistic effect of PD-1 inhibitors and brain radiotherapy for BMs in lung cancer is still scarce. This study indicates PD-1 inhibitors combined with concurrent brain radiotherapy can improve iPFS. A trend toward a PFS and OS benefit was also observed in the concurrent group. These findings should be further investigated in prospective analyses.
Acknowledgments
We thank Haiying Hu from MSD China for editorial assistance and text proofreading.
Funding: This study was supported by the grants from the National Key R&D Program of China (Grant No. 2019YFC1316205).
Footnote
Reporting Checklist: The authors have completed the STROBE reporting checklist. Available at https://dx.doi.org/10.21037/apm-21-2334
Data Sharing Statement: Available at https://dx.doi.org/10.21037/apm-21-2334
Conflicts of Interest: All authors have completed the ICMJE uniform disclosure form (available at https://dx.doi.org/10.21037/apm-21-2334). The authors have no conflicts of interest to declare.
Ethical Statement: The authors are accountable for all aspects of the work in ensuring that questions related to the accuracy or integrity of any part of the work are appropriately investigated and resolved. All procedures performed in this study involving human participants were in accordance with the Declaration of Helsinki (as revised in 2013). The study was approved by the Ethics Committee of Union Hospital, Tongji Medical College, Huazhong University of Science and Technology. Patient data were retrieved from the hospital medical record system, so an informed consent form was not required. The patients’ personal data has been secured.
Open Access Statement: This is an Open Access article distributed in accordance with the Creative Commons Attribution-NonCommercial-NoDerivs 4.0 International License (CC BY-NC-ND 4.0), which permits the non-commercial replication and distribution of the article with the strict proviso that no changes or edits are made and the original work is properly cited (including links to both the formal publication through the relevant DOI and the license). See: https://creativecommons.org/licenses/by-nc-nd/4.0/.
References
- Mulvenna P, Nankivell M, Barton R, et al. Dexamethasone and supportive care with or without whole brain radiotherapy in treating patients with non-small cell lung cancer with brain metastases unsuitable for resection or stereotactic radiotherapy (QUARTZ): results from a phase 3, non-inferiority, randomised trial. Lancet 2016;388:2004-14. [Crossref] [PubMed]
- Huang Y, Wang Y, Hu D, et al. The influence of pemetrexed-based continuous maintenance therapy on survival of locally advanced and metastatic lung adenocarcinoma. Ann Transl Med 2019;7:524. [Crossref] [PubMed]
- Wang S, Hu C, Xie F, et al. Use of Programmed death receptor-1 and/or programmed death ligand 1 inhibitors for the treatment of brain metastasis of lung cancer. Onco Targets Ther 2020;13:667-83. [Crossref] [PubMed]
- Travert C, Tomasini P, Jeanson A, et al. First-line pembrolizumab in programmed death ligand 1 positive non-small cell lung cancer. Transl Cancer Res 2019;8:2514-6. [Crossref]
- Gandhi L, Rodríguez-Abreu D, Gadgeel S, et al. Pembrolizumab plus chemotherapy in metastatic non-small-cell lung cancer. N Engl J Med 2018;378:2078-92. [Crossref] [PubMed]
- Schouten RD, Egberink L, Muller M, et al. Nivolumab in pre-treated advanced non-small cell lung cancer: long term follow up data from the Dutch expanded access program and routine clinical care. Transl Lung Cancer Res 2020;9:1736-48. [Crossref] [PubMed]
- Paz-Ares L, Dvorkin M, Chen Y, et al. Durvalumab plus platinum-etoposide versus platinum-etoposide in first-line treatment of extensive-stage small-cell lung cancer (CASPIAN): a randomised, controlled, open-label, phase 3 trial. Lancet 2019;394:1929-39. [Crossref] [PubMed]
- Kamath SD, Kumthekar PU. Immune Checkpoint inhibitors for the treatment of central nervous system (CNS) metastatic disease. Front Oncol 2018;8:414. [Crossref] [PubMed]
- Lin NU, Prowell T, Tan AR, et al. Modernizing clinical trial eligibility criteria: recommendations of the American Society of Clinical Oncology-friends of cancer research brain metastases working group. J Clin Oncol 2017;35:3760-73. [Crossref] [PubMed]
- Goldberg SB, Schalper KA, Gettinger SN, et al. Pembrolizumab for management of patients with NSCLC and brain metastases: long-term results and biomarker analysis from a non-randomised, open-label, phase 2 trial. Lancet Oncol 2020;21:655-63. [Crossref] [PubMed]
- Cohen JV, Kluger HM. Systemic Immunotherapy for the treatment of brain metastases. Front Oncol 2016;6:49. [Crossref] [PubMed]
- Chen L, Douglass J, Kleinberg L, et al. Concurrent immune checkpoint inhibitors and stereotactic radiosurgery for brain metastases in non-small cell lung cancer, melanoma, and renal cell carcinoma. Int J Radiat Oncol Biol Phys 2018;100:916-25. [Crossref] [PubMed]
- Sharabi AB, Lim M, DeWeese TL, et al. Radiation and checkpoint blockade immunotherapy: radiosensitisation and potential mechanisms of synergy. Lancet Oncol 2015;16:e498-509. [Crossref] [PubMed]
- Zeng J, See AP, Phallen J, et al. Anti-PD-1 blockade and stereotactic radiation produce long-term survival in mice with intracranial gliomas. Int J Radiat Oncol Biol Phys 2013;86:343-9. [Crossref] [PubMed]
- Sperduto PW, Kased N, Roberge D, et al. Summary report on the graded prognostic assessment: an accurate and facile diagnosis-specific tool to estimate survival for patients with brain metastases. J Clin Oncol 2012;30:419-25. [Crossref] [PubMed]
- Kluger HM, Chiang V, Mahajan A, et al. Long-term survival of patients with melanoma with active brain metastases treated with pembrolizumab on a phase II trial. J Clin Oncol 2019;37:52-60. [Crossref] [PubMed]
- Crinò L, Bronte G, Bidoli P, et al. Nivolumab and brain metastases in patients with advanced non-squamous non-small cell lung cancer. Lung Cancer 2019;129:35-40. [Crossref] [PubMed]
- Gauvain C, Vauléon E, Chouaid C, et al. Intracerebral efficacy and tolerance of nivolumab in non-small-cell lung cancer patients with brain metastases. Lung Cancer 2018;116:62-6. [Crossref] [PubMed]
- Zhang G, Cheng R, Wang H, et al. Comparable outcomes of nivolumab in patients with advanced NSCLC presenting with or without brain metastases: a retrospective cohort study. Cancer Immunol Immunother 2020;69:399-405. [Crossref] [PubMed]
- Gadgeel SM, Lukas RV, Goldschmidt J, et al. Atezolizumab in patients with advanced non-small cell lung cancer and history of asymptomatic, treated brain metastases: Exploratory analyses of the phase III OAK study. Lung Cancer 2019;128:105-12. [Crossref] [PubMed]
- Kotecha R, Kim JM, Miller JA, et al. The impact of sequencing PD-1/PD-L1 inhibitors and stereotactic radiosurgery for patients with brain metastasis. Neuro Oncol 2019;21:1060-8. [Crossref] [PubMed]
- Shepard MJ, Xu Z, Donahue J, et al. Stereotactic radiosurgery with and without checkpoint inhibition for patients with metastatic non-small cell lung cancer to the brain: a matched cohort study. J Neurosurg 2019;26:1-8. [PubMed]
- Ahmed KA, Kim S, Arrington J, et al. Outcomes targeting the PD-1/PD-L1 axis in conjunction with stereotactic radiation for patients with non-small cell lung cancer brain metastases. J Neurooncol 2017;133:331-8. [Crossref] [PubMed]
- Schapira E, Hubbeling H, Yeap BY, et al. Improved overall survival and locoregional disease control with concurrent PD-1 pathway inhibitors and stereotactic radiosurgery for lung cancer patients with brain metastases. Int J Radiat Oncol Biol Phys 2018;101:624-9. [Crossref] [PubMed]
- Shaverdian N, Lisberg AE, Bornazyan K, et al. Previous radiotherapy and the clinical activity and toxicity of pembrolizumab in the treatment of non-small-cell lung cancer: a secondary analysis of the KEYNOTE-001 phase 1 trial. Lancet Oncol 2017;18:895-903. [Crossref] [PubMed]
- Pike LRG, Bang A, Mahal BA, et al. The impact of radiation therapy on lymphocyte count and survival in metastatic cancer patients receiving PD-1 immune checkpoint inhibitors. Int J Radiat Oncol Biol Phys 2019;103:142-51. [Crossref] [PubMed]
- Téglási V, Pipek O, Lózsa R, et al. PD-L1 expression of lung cancer cells, unlike infiltrating immune cells, is stable and unaffected by therapy during brain metastasis. Clin Lung Cancer 2019;20:363-369.e2. [Crossref] [PubMed]
- Sacher AG, Gandhi L. Biomarkers for the clinical use of PD-1/PD-L1 inhibitors in non-small-cell lung cancer: A Review. JAMA Oncol 2016;2:1217-22. [Crossref] [PubMed]
- Willis C, Fiander M, Tran D, et al. Tumor mutational burden in lung cancer: A systematic literature review. Oncotarget 2019;10:6604-22. [Crossref] [PubMed]
- Ng TL, Liu Y, Dimou A, et al. Predictive value of oncogenic driver subtype, programmed death-1 ligand (PD-L1) score, and smoking status on the efficacy of PD-1/PD-L1 inhibitors in patients with oncogene-driven non-small cell lung cancer. Cancer 2019;125:1038-49. [Crossref] [PubMed]
- Wang GZ, Zhang L, Zhao XC, et al. The Aryl hydrocarbon receptor mediates tobacco-induced PD-L1 expression and is associated with response to immunotherapy. Nat Commun 2019;10:1125. [Crossref] [PubMed]
(English Language Editor: D. Fitzgerald)