Iron deficiency is an independent risk factor of increased myocardial energy expenditure in chronic heart failure patients
Introduction
Chronic heart failure (CHF) is a major public health burden worldwide and is associated with high morbidity, mortality, and cost. Despite great progress in CHF treatment over the years, the myocardial performance of many patients with CHF remains restricted (1). While it is well known that many aspects of myocardial energetics are altered in heart failure (2), our knowledge of the mechanisms and pathways that regulate myocardial energy metabolism is still limited.
Iron is an essential micronutrient. As a component of hemoglobin (HGB), myoglobin, oxidative enzymes, and respiratory chain proteins, it plays a crucial role in oxygen transport, storage, oxidative metabolism in skeletal muscle, and cellular energetics (3,4). The mechanisms are supposed to be involved in the development of iron deficiency (ID) in CHF: (I) insufficient dietary iron supply, (II) poor gastrointestinal absorption, impaired duodenal iron transport, drug interactions (e.g., omeprazole), or food reducing absorption, and (III) gastrointestinal blood loss (5). Traditionally, iron deficiency, a common comorbidity in CHF, was considered to have clinical consequences only in the presence of anemia (6-8). However, a decreased HGB content can be viewed as the result of the gradual depletion of iron storage (4,9). Recently, ID was found to be associated with increased disease severity and exercise intolerance, and may contribute to poor prognosis, regardless of the baseline anemia status (10-14). Several studies have shown that intravenous iron supplementation in CHF patients complicated by ID with or without anemia conferred favorable benefits to cardiac function, functional capacity, and quality of life (10-16). In spite of its undoubtable role in maintaining oxidative metabolism homeostasis, iron is also a key regulator of mitochondrial biogenesis (17,18) and is necessary for the maintenance of cellular energy metabolism (4,17,19,20). To perform contraction and active relaxation, cardiac myocytes require a high level of energy (21), and theoretically, ID and/or abnormal iron utilization may result in abnormal energy generation and utilization. However, data on this topic is limited and no research has directly studied myocardial energy metabolism in CHF patients with ID. Currently studies on myocardial energy metabolism concentrated in cardiac magnetic resonance imaging. However, it can not be applied in most hospital in clinical work. In our prior study, we found that myocardial energy metabolism can be conveniently accessed by echocardiography (22). Myocardial energy expenditure (MEE) is an important indicator reflecting myocardial energy metabolism (22). It has been reported that elevated MEE is associated with decreased left ventricular ejection fraction (LVEF) and can be used as an independent predictor of cardiovascular mortality (23).
The purpose of this study was to evaluate the correlation between iron metabolism and MEE in patients with CHF, and to explore the effect of iron status on MEE. We present the following article in accordance with the STROBE reporting checklist (available at https://dx.doi.org/10.21037/apm-21-2297).
Methods
Study population
Patients presenting to the Cardiovascular department of Nanfang Hospital of Southern Medical University between Jan. 2014 and Jan. 2015 were enrolled (n=130). Patients with acute coronary syndrome, coronary revascularization or any major surgery within 3 months preceding the study, severe valvular disease or arrhythmia, any acute/chronic illness that might influence iron metabolism (infection, malignancy, severe renal disease requiring dialysis, severe hepatic disease, autoimmune disease, and hematological disease), or any anemia or/and ID treatment either at the time of the study or in the past 12 months were excluded. Thirty-five sex and age matched healthy subjects were also recruited. The study protocol was approved by the Institutional Ethics Committee of Nanfang Hospital of Southern Medical University, China (No.: KTLL-20130425), and all subjects provided written informed consent. The study followed the protocols of the Declaration of Helsinki (as revised in 2013).
Iron status and other laboratory measurements
Venous blood samples were taken from all participants in the morning after a fasting period of more than 8 hours. Laboratory measurements were assessed from fresh venous blood with or without EDTA as an anticoagulant, respectively. After centrifugation (3,000 rpm for 10 min), serum and plasma samples were extracted and stored at −80 °C until being analyzed.
Laboratory parameters reflecting iron metabolism were measured, including ferritin, iron, transferrin, soluble transferrin receptor (sTfR), and total iron binding capacity (TIBC). The Transferrin saturation (Tsat) was estimated using the ratio of serum iron and TIBC multiplied by 100 and expressed as a percentage. Ferritin was measured using an immunoassay with ADVIA CENTAUR XP (Siemens, Healthcare Diagnostics Inc, USA), and serum iron, transferrin, sTfR, and TIBC were calculated using an Olympus AU5421 analyzer. ID was defined as serum ferritin <100 µg/L, or serum ferritin 100–300 µg/L with Tsat <20%.
HGB concentration (g/L) was measured using an Sysmex XE-2100 automated hematology analyzer (Toa medical Electronics, Kobe, Japan). Anemia was defined as HGB <110 g/L in women and <120 g/L in men.
N-terminal pro-B-type natriuretic peptide (NT-proBNP) was measured using immunoassay based on electrochemiluminescence on the Elecsys 2010 System (Roche Diagnostics, Mannheim, Germany), and estimated glomerular filtration rate (eGFR) was calculated based on the Modification of Diet in Renal Disease (MDRD) formula.
Myocardial energy expenditure (MEE) measurement
MEE was measured with a Siemens Sequoia 512 Encompass ultrasound system, using the method described previously (22-24). Systolic blood pressure (SBP), left ventricular internal diameter at systole (LVIDs), left ventricular posterior wall end-systolic thickness (PWTs), left ventricular ejection time (LVET), left ventricular mass index (LVMI), left ventricular ejection fraction (LVEF), and left ventricular stroke volume (LVSV) were measured, and MEE was calculated as MEE (cal/min) = left ventricular circumferential end-systolic wall stress (cESS) × LVET × LVSV × heart rate ×4.2×10−4.
Statistical analysis
Continuous normal variables were expressed as mean and standard deviations, while those with skewed distribution were presented as medians with the 25th to 75th percentiles. Categorical variables were expressed as numbers with percentages. Intergroup differences were analyzed using one-way ANOVA analysis, Kruskall-Wallis test, Mann-Whitney U test, or Pearson’s χ2 test, as appropriate. Univariable linear regression analysis was used to assess the associations between MEE levels and other study parameters including age, BMI, gender, NYHA class, etiology of CHF, medications, laboratory measurements, major comorbidities (renal function assessed using eGFR or the presence of diabetes mellitus), and the presence of ID. To explore the effect of iron metabolism on MEE levels, multivariable linear stepwise regression models adjusted for all covariates that showed significant association with MEE levels in univariable models was constructed.
All tests were two-sided, and P values <0.05 were considered statistically significant. All statistical analyses were carried out using SPSS version 19.0 (SPSS Inc., Chicago, IL).
Results
Baseline characteristics
The baseline characteristics of 130 CHF patients and 35 healthy subjects are shown in Table 1. Compared with healthy controls, patients with CHF had similar demographic characteristics (age, sex and BMI) (all P>0.05), and an increased prevalence of ID (36.9% vs. 14.3%, P=0.011) and MEE levels (75.6±25.2 vs. 52.4±8.7 cal/min, P=0.000). ID was present in 48 (36.9%) of patients with CHF, and its prevalence increased with the severity of CHF, reaching 80.0% in those with NYHA class IV (NYHA class II/III/IV: 17.9% vs. 46.5% vs. 80.0%, P=0.000) (Figure 1). Patients with CHF were divided into two groups based on the presence or absence of ID. Clinical parameters in the two groups were similar, except for higher NYHA class, smoking, MEE levels, and sTfR concentrations, as well as lower ferritin and Tsat in the ID group compared with the non-ID group (Table 2). In the subgroups analysis according to NYHA class, patients with NYHA class II-III had higher MEE levels in the ID group compared to the non-ID group (71.6±16.4 vs. 60.3±14.8 cal/min, P=0.022; 88.9±10.4 vs. 69.1±20.1 cal/min, P=0.000; respectively) (Figure 2). However, there was no difference in MEE levels between ID and non-ID patients with NYHA class IV (113.4±22.1 vs. 118.7±19.6 cal/min, P =0.668) (Figure 2).
Table 1
Variables | All patients (n=130) | Healthy subjects (n=35) | P values |
---|---|---|---|
Age, years | 66.2±11.5 | 63.5±6.3 | 0.184 |
Male gender, n (%) | 76 (58.5) | 20 (57.1) | 0.888 |
BMI, kg/m2 | 23.8±3.0 | 23.9±3.3 | 0.820 |
Systolic BP, mmHg | 128.4±18.5 | 123.7±12.3 | 0.076 |
Diastolic BP, mmHg | 73.6±12.4 | 71.7±6.6 | 0.211 |
Heart rate, b.p.m | 78.2±9.0 | 75.2±6.6 | 0.069 |
NYHA class (II/III/IV), n (%) | 67/43/20 (51.5/33.1/15.4) | – | – |
Smokers (past or present), n (%) | 67 (51.5) | 15 (42.9) | 0.362 |
Ischemic etiology of CHF, n (%) | 94 (72.3) | – | – |
Iron deficiency, n (%) | 48 (36.9) | 5 (14.3) | 0.011 |
Anemia, n (%) | 44 (33.8) | 5 (14.3) | 0.000– |
Diabetes mellitus, n (%) | 45 (34.6) | – | – |
Medications, n (%) | |||
ACEI or ARB | 122 (93.8) | – | – |
Beta-blocker | 119 (91.5) | – | – |
Aldosterone antagonist | 52 (40.0) | – | – |
Loop diuretic | 68 (52.3) | – | – |
Digoxin | 9 (6.9) | – | – |
Statin | 110 (84.6) | – | – |
Antiplatelet drug | 108 (83.1) | – | – |
Laboratory measurements | |||
Hemoglobin, g/L | 119.4±18.2 | 126.1±9.8 | 0.005 |
eGFR, mL/min/1.73 m2 | 73.6±28.4 | ||
CRP, mg/L | 4.1 (1.6–14.3) | ||
Ferritin, μg/L | 263.7 (135.9–428.3) | 315.5 (139.0–422.0) | 0.582 |
Transferrin, g/L | 2.0±0.5 | 2.1±0.4 | 0.308 |
Serum iron, μmol/L | 10.9±5.6 | 14.7±4.7 | 0.000 |
Tsat, % | 22.8 (15.4–32.1) | 36.3 (32.9–44.6) | 0.000 |
sTfR, mg/L | 1.3 (1.0–2.0) | 1.1 (0.9–1.3) | 0.004 |
TIBC, μmol/L | 45.4±10.8 | 39.5±6.8 | 0.000 |
Nt-proBNP, ng/L | 1,152.0 (436.3–5,689.6) | 119.7 (88.7–226.3) | 0.000 |
LVEF, % | 47.4±10.8 | 61.3±5.3 | 0.000 |
LVFS, % | 27.9±10.7 | 41.3±4.4 | 0.000 |
LVIDd, mm | 49.9±8.9 | 40.1±3.2 | 0.000 |
LVMI, g/m2 | 128.6±39.3 | 84.1±12.5 | 0.000 |
MEE, cal/min | 75.6±25.2 | 52.4±8.7 | 0.000 |
Data are presented as means ± SD, or median (inter quartile range). CHF, chronic heart failure; BMI, body mass index; BP, blood pressure; ACEI, angiotensin converting enzyme inhibitors; ARB, angiotensin receptor blocker; NYHA, New York Heart Association; eGFR, estimated glomerular filtration rate; NT-proBNP, N-terminal pro-B-type natriuretic peptide; CRP, C-reactive protein; Tsat, transferrin saturation; sTfR, soluble transferrin receptor; TIBC, total iron binding capacity; LVEF, left ventricular ejection fraction; LVFS, left ventricular fractional shortening; LVIDd, left ventricular internal diastolic diameter; LVMI, left ventricular mass index; MEE, myocardial energy expenditure.
Table 2
Variables | Patients with ID (n=48) | Patients without ID (n=82) | P values |
---|---|---|---|
Age, years | 64.1±12.6 | 67.5±10.7 | 0.109 |
Male gender, n (%) | 30 (62.5) | 35 (42.7) | 0.476 |
BMI, kg/m2 | 23.5±2.8 | 23.9±2.9 | 0.378 |
Systolic BP, mmHg | 128.4±18.5 | 126.4±18.6 | 0.111 |
Diastolic BP, mmHg | 74.9±12.6 | 72.9±12.2 | 0.361 |
Heart rate, b.p.m | 80.1±9.2 | 77.1±8.7 | 0.056 |
NYHA class (II/III/IV), n (%) | 12/20/16 (25.0/41.7/33.3) | 55/23/4 (67.1/28.0/4.9) | 0.000 |
Smokers (past or present), n (%) | 33 (68.8) | 34 (41.5) | 0.004 |
Ischemic etiology of CHF, n (%) | 40 (83.3) | 64 (78.0) | 0.505 |
Anemia, n (%) | 19 (39.6) | 20 (24.4) | 0.077 |
Diabetes mellitus, n (%) | 18 (37.5) | 27 (32.9) | 0.703 |
Medications, n (%) | |||
ACEI or ARB | 43 (89.6) | 79 (96.3) | 0.144 |
Beta-blocker | 42 (87.5) | 77 (93.9) | 0.327 |
Aldosterone antagonist | 21 (43.8) | 31 (37.8) | 0.579 |
Loop diuretic | 28 (58.3) | 40 (48.8) | 0.363 |
Digoxin | 4 (8.3) | 2 (2.4) | 0.193 |
Statin | 40 (83.3) | 70 (85.4) | 0.804 |
Antiplatelet drug | 40 (83.3) | 68 (82.9) | 0.952 |
Laboratory measurements | |||
Hemoglobin, g/L | 116.0±19.1 | 121.3±17.6 | 0.111 |
eGFR, mL/min/1.73 m2 | 76.3±29.0 | 71.9±28.2 | 0.398 |
CRP, mg/L | 3.9 (1.6–11.6) | 4.4 (1.5–15.6) | 0.765 |
Ferritin, μg/L | 82.0 (53.3–195.3) | 380.0 (250.8–516.7) | 0.000 |
Transferrin, g/L | 2.2±0.6 | 1.9±0.4 | 0.002 |
Serum iron, μmol/L | 8.1±5.2 | 12.5±5.2 | 0.000 |
Tsat, % | 14.3 (10.7–19.3) | 27.7 (21.3–35.4) | 0.000 |
sTfR, mg/L | 1.4 (1.1–2.3) | 1.2 (0.9–1.6) | 0.007 |
TIBC, μmol/L | 49.7±11.9 | 42.9±9.2 | 0.000 |
Nt-proBNP, ng/L | 2,634.6 (808.1–10,288.7) | 871.3 (254.6–3,781.0) | 0.003 |
LVEF, % | 47.4±10.8 | 47.4±10.9 | 0.997 |
LVFS, % | 25.4±11.3 | 29.4±10.2 | 0.042 |
LVIDd, mm | 52.0±8.1 | 48.7±9.1 | 0.036 |
LVMI, g/m2 | 137.9±38.6 | 123.1±38.9 | 0.038 |
MEE, cal/min | 92.7±23.0 | 65.6±20.8 | 0.000 |
Data are presented as means ± SD, or median (inter quartile range). CHF, chronic heart failure; BMI, body mass index; BP, blood pressure; ACEI, angiotensin converting enzyme inhibitors; ARB, angiotensin receptor blocker; NYHA, New York Heart Association; eGFR, estimated glomerular filtration rate; NT-proBNP, N-terminal pro-B-type natriuretic peptide; CRP, C-reactive protein; Tsat, transferrin saturation; sTfR, soluble transferrin receptor; TIBC, total iron binding capacity; LVEF, left ventricular ejection fraction; LVFS, left ventricular fractional shortening; LVIDd, left ventricular internal diastolic diameter; LVMI, left ventricular mass index; MEE, myocardial energy expenditure.
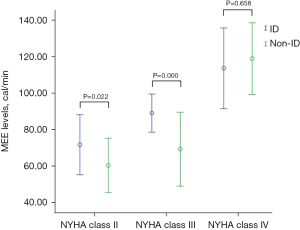
Univariable analyses
In univariable linear regression models, using ACEI/ARB and an aldosterone antagonist, the presence of ID, higher NYHA class, increased NT-proBNP, sTfR concentrations, left ventricular internal diastolic diameter (LVIDd), and LVMI, as well as reduced ferritin, Tsat levels, and lower LVEF, left ventricular fractional shortening (LVFS) were associated with elevated MEE levels (all P<0.05, Table 3). In addition, MEE levels did not change significantly corresponding to age, sex, the presence of anemia, or baseline HGB concentrations (all P>0.05, Table 3).
Table 3
Determinants | Univariable models | Multivariable model 1* | Multivariable model 2** | ||||||
---|---|---|---|---|---|---|---|---|---|
Sβc | R2 | P values | Sβc | P values | Sβc | P values | |||
Age, years | −0.125 | 0.016 | 0.157 | − | − | − | − | ||
Gender, male vs. female | −0.111 | 0.012 | 0.207 | − | − | − | − | ||
BMI, kg/m2 | −0.013 | 0.000 | 0.879 | − | − | − | − | ||
NYHA class, II/III/IV | 0.695 | 0.484 | 0.000 | 0.399 | 0.000 | 0.518 | 0.000 | ||
Smoker, yes vs. no | −0.115 | 0.013 | 0.192 | − | − | − | − | ||
Ischemic CHF etiology, yes vs. no | 0.099 | 0.010 | 0.263 | − | − | − | − | ||
Diabetes mellitus, yes vs. no | −0.106 | 0.011 | 0.231 | − | − | − | − | ||
ID, yes vs. no | −0.520 | 0.270 | 0.000 | −0.217 | 0.000 | − | − | ||
Anemia, yes vs. no | −0.145 | 0.021 | 0.101 | − | − | − | − | ||
ACEI/ARB, yes vs. no | 0.311 | 0.097 | 0.000 | − | − | − | − | ||
Β-blocker, yes vs. no | 0.089 | 0.008 | 0.314 | − | − | − | − | ||
Aldosterone antagonist, yes vs. no | −0.219 | 0.048 | 0.012 | − | − | − | − | ||
Loop diuretic, yes vs. no | −0.171 | 0.029 | 0.052 | − | − | − | − | ||
Digoxin, yes vs. no | −0.019 | 0.000 | 0.832 | − | − | − | − | ||
Statin, yes vs. no | 0.135 | 0.018 | 0.126 | − | − | − | − | ||
Antiplatelet drug, yes vs. no | 0.050 | 0.003 | 0.568 | − | − | − | − | ||
Hemoglobin, g/L | −0.107 | 0.011 | 0.228 | − | − | − | − | ||
eGFR, mL/min/1.73 m2 | −0.062 | 0.004 | 0.484 | − | − | − | − | ||
CRP, mg/L | 0.066 | 0.004 | 0.455 | − | − | − | − | ||
Ferritin, μg/L | −0.295 | 0.087 | 0.001 | − | − | − | − | ||
Tsat, % | −0.279 | 0.078 | 0.001 | − | − | −0.130 | 0.020 | ||
sTfR, mg/L | 0.463 | 0.215 | 0.000 | 0.134 | 0.027 | 0.137 | 0.015 | ||
Nt-proBNP, ng/L | 0.401 | 0.161 | 0.000 | − | − | − | − | ||
LVEF, % | −0.227 | 0.052 | 0.009 | −0.150 | 0.015 | −0.225 | 0.000 | ||
LVFS, % | −0.559 | 0.313 | 0.000 | −0.385 | 0.000 | −0.362 | 0.000 | ||
LVIDd, mm | 0.528 | 0.278 | 0.000 | 0.270 | 0.001 | 0.207 | 0.003 | ||
LVMI, g/m2 | 0.383 | 0.147 | 0.000 | 0.193 | 0.015 | − | − |
*, adjusted R2=0.685; **, adjusted R2=0.644. CHF, chronic heart failure; BMI, body mass index; BP, blood pressure; ACEI, angiotensin converting enzyme inhibitors; ARB, angiotensin receptor blocker; NYHA, New York Heart Association; eGFR, estimated glomerular filtration rate; NT-proBNP, N-terminal pro-B-type natriuretic peptide; CRP, C-reactive protein; Tsat, transferrin saturation; sTfR, soluble transferrin receptor; LVEF, left ventricular ejection fraction; LVFS, left ventricular fractional shortening; LVIDd, left ventricular internal diastolic diameter; LVMI, left ventricular mass index; MEE, myocardial energy expenditure; Sβc, standardized β coefficient.
Multivariable analyses
In a multivariable regression model with ID as a predefined category for iron status and adjusting for all associated clinical variables identified in the univariable analyses, the presence of ID, increased LVIDd, LVMI, and sTfR concentrations as well as reduced LVEF, LVFS significantly predicted the elevation of MEE levels (all P<0.05, Table 3). In the analogous multivariable regression model with ferritin and Tsat levels respectively reflecting iron status, lower Tsat, but not ferritin, remained an independent predictor of elevation of MEE levels (P=0.020, Table 3), and after adjustment for all variables that showed a significant association with MEE levels in univariable models. In both models, increased sTfR concentrations significantly predicted the elevation of MEE levels (both P<0.05, Table 3).
Discussion
In the present study, the prevalence of ID elevated significantly in patients with CHF compared with healthy subjects, affecting 36.9% of the CHF patients recruited. With the progression of heart failure as accessed by NYHA class, the prevalence of ID increased. MEE levels were significantly higher in CHF patients with ID compared with patients without ID, and MEE levels were adversely affected by the presence of ID as well as reduced Tsat and increased sTfR concentrations, independent of baseline anemia and other related clinical parameters.
Prevalence of ID
ID is one of the most common nutritional deficiencies worldwide, affecting one-third of the global population (19). Traditionally, ID has usually been considered to be a cause of anemia in patients with CHF (3,6,7), and until recently has been reported as a common complication of CHF associated with impaired exercise capacity, poor quality of life and worse prognosis, regardless of baseline anemia status (25-30). However, data on the epidemiology and pathophysiology of ID in patients with CHF are limited. The prevalence of ID in different CHF patients ranged from 21–73% (7,12,25,31-33). This wide variation might be explained by the different cut off levels and criteria for ID in previously reported studies and difference in the patient characteristics. The etiology of ID in patients with CHF is generally considered multifactorial, and may be a consequence of reduced iron absorption, increased gastro-intestinal loss, and impaired availability of iron from the reticuloendothelial system caused by pro-inflammatory activation with hepcidin overproduction (34,35). In the present study, we applied a definition of ID most commonly used in CHF: serum ferritin <100 µg/L, or serum ferritin 100–300 µg/L with Tsat <20%. Such a definition of ID has been applied in CHF, including clinical trials (10-12,16,25-28,30). With this criterion, a high prevalence of ID in patients with CHF was found, affecting 36.9% of the CHF patients. In addition to the severity of CHF accessed by NYHA class, patients with ID also appeared to have higher sTfR concentrations. In contrast with previously reported studies (12,25,26,31,32,36,37). CHF patients with ID in our study had similar demographic characteristics (age, sex, BMI, BP, and HR), eGFR, LVEF, HGB, NT-proBNP concentrations, and the prevalence of anemia, as well as medication treatment, compared with those without ID.
Roles of iron in CHF
Iron is essential for the maintenance of cellular energy and metabolism of extra-haematopoietic tissues (3,9,17-19). Cardiac myocytes with a high mitogenic potential and high-energy demand are particularly sensitive to depleted iron supplies and/or abnormal iron utilization. This is important in CHF, as abnormal energy generation and utilization in the myocardium and skeletal muscles contribute to CHF pathophysiology.
Alterations of MEE in CHF
MEE derived from standard echocardiographic measurements is a major indicator of myocardial energy metabolism, and several studies have shown that it was an effective parameter of myocardial bioenergetics and was significantly correlated with cardiac function in patients with CHF (23,24,38,39). Elevated MEE levels were proved to be more effective in predicting cardiac death than LVEF (23), and recently, we also found that a higher MEE level was an independent predictor of all-cause mortality and recurrent hospital admission due to CHF (40). In the current study, MEE levels also increased with the severity of HF assessed by NYHA class and NT-proBNP levels, which was consistent with prior reports and suggested that elevation of MEE levels might be a good indicator of compromised energy metabolism in CHF.
Correlation between iron metabolism and MEE in patients with CHF
In recent years, myocardial metabolic remodeling in CHF has attracted great attention, and studies have shown that impaired myocardial energy metabolism was a feature of CHF (2,41,42). Iron, as a key regulator of mitochondrial biogenesis and indispensable components of several enzyme cofactors in energy metabolism, plays a crucial role in mitochondrial function and energy metabolic homeostasis (17,43,44). Cardiac myocytes characterizing by high energy demand are prone to be susceptible to disorders of iron homeostasis (19,20,45), and in vitro experiments, ID could induce cardiac hypertrophy and/or dilation as an adaptive response, involvement of mitochondrial ultrastructural aberrations, and dysfunction (46-49). The effects of intravenous iron supplementation in CHF patients were reported in several studies (12-16,26), shown significant improvements in functional status, quality of life, and exercise capacity . Thus, we hypothesize that CHF patients complicated with ID would further worsen already impaired myocardial energy metabolism. In the multivariable regression models, and adjusting for all associated clinical variables identified in the univariable analyses, the presence of ID, as well as reduced Tsat, reflected the depletion of body iron storage (5,19,50,51), and significantly predicted the elevation of MEE levels, regardless of baseline anemia status. This suggested that ID may aggravate myocardial energy metabolism in patients with CHF. In addition to improvements in the symptoms of CHF, exercise capacity, and quality of life (10,11,13-16), iron supplementation may facilitate energy metabolic optimization in patients with CHF accompanying ID. In the subgroups analysis according to NYHA class, MEE levels significantly increased in the presence of ID in NYHA class II and III groups, although analogous variation was not found in the NYHA class IV group. This may be because serum ferritin increases in response to the activation of pro-inflammatory cytokines in the process of CHF, which may affect the diagnosis of ID using the present definition.
Compared with healthy subjects, sTfR concentrations were higher in patients with CHF, and similar variation was found in the CHF patients with ID as compared to those without ID. In addition, a higher concentration of sTfR was associated with increased MEE levels after adjustment for traditional covariates in the multivariable models. Consistent with the inverse correlation between iron metabolism and myocardial energy metabolism, sTfR, as a sensitive indicator of ID, increases when iron availability is insufficient for metabolic requirement (5,19,50,51). Thus, increased sTfR levels may be an indicator of abnormal iron utilization with restricted iron delivery to target cells, further aggravating already impaired energy metabolism in patients with CHF.
Study limitations
There are several limitations to this study. First, there are no standard criteria for the diagnosis of ID with serum iron biomarkers in patients with CHF. Using the criterion standard of bone marrow iron staining in all patients, as well as control subjects appears impractical and non-ethical. In the present study, we applied a definition of ID as serum ferritin <100 µg/L, or serum ferritin 100–300 µg/L with Tsat <20%, reflecting depleted iron storage and abnormal iron utilization despite abundant body iron stores. However, more studies are warranted to confirm the role of serum iron biomarkers in body iron status in patients with CHF. Second, the observational parameters of our study were single measurement after admission and variations in iron status over time were not studied. Third, only the correlation between iron metabolism and MEE levels was analyzed in the study, while the mechanism was not investigated. In addition, no information on prognosis in patients with CHF complicated with ID was available for the present study. However, several previously reported studies have confirmed that ID was an independent predictor of poor prognosis in patients with CHF. Finally, the effect of iron supplementation on improving myocardial energy metabolism in patients with CHF warrants further studies.
Conclusions
ID is common in patients with CHF. The presence of ID as well as reduced Tsat and increased sTfR levels is associated with elevation of MEE levels in patients suffering from CHF, suggesting iron supplementation in CHF patients with ID may be a potential therapeutic target for improving myocardial energy metabolism.
Acknowledgments
Funding: None.
Footnote
Reporting Checklist: The authors have completed the STROBE reporting checklist. Available at https://dx.doi.org/10.21037/apm-21-2297
Data Sharing Statement: Available at https://dx.doi.org/10.21037/apm-21-2297
Conflicts of Interest: All authors have completed the ICMJE uniform disclosure form (available at https://dx.doi.org/10.21037/apm-21-2297). The authors have no conflicts of interest to declare.
Ethical Statement: The authors are accountable for all aspects of the work in ensuring that questions related to the accuracy or integrity of any part of the work are appropriately investigated and resolved. The study protocol was approved by the Institutional Ethics Committee of Nanfang Hospital of Southern Medical University, China (No.: KTLL-20130425), and all subjects provided written informed consent. The study followed the protocols of the Declaration of Helsinki (as revised in 2013).
Open Access Statement: This is an Open Access article distributed in accordance with the Creative Commons Attribution-NonCommercial-NoDerivs 4.0 International License (CC BY-NC-ND 4.0), which permits the non-commercial replication and distribution of the article with the strict proviso that no changes or edits are made and the original work is properly cited (including links to both the formal publication through the relevant DOI and the license). See: https://creativecommons.org/licenses/by-nc-nd/4.0/.
References
- Jessup M, Brozena S. Heart failure. N Engl J Med 2003;348:2007-18. [Crossref] [PubMed]
- van Bilsen M, Smeets PJ, Gilde AJ, et al. Metabolic remodelling of the failing heart: the cardiac burn-out syndrome? Cardiovasc Res 2004;61:218-26. [Crossref] [PubMed]
- Opasich C, Cazzola M, Scelsi L, et al. Blunted erythropoietin production and defective iron supply for erythropoiesis as major causes of anaemia in patients with chronic heart failure. Eur Heart J 2005;26:2232-7. [Crossref] [PubMed]
- Fairbanks VF, Tefferi A. Correction to response to Pearson (Eur J Hematol 67(1): 56-69) on normal ranges for PCV and hemoglobin. Eur J Haematol 2001;67:203-4. [PubMed]
- Jankowska EA, von Haehling S, Anker SD, et al. Iron deficiency and heart failure: diagnostic dilemmas and therapeutic perspectives. Eur Heart J 2013;34:816-29. [Crossref] [PubMed]
- Ezekowitz JA, McAlister FA, Armstrong PW. Anemia is common in heart failure and is associated with poor outcomes: insights from a cohort of 12 065 patients with new-onset heart failure. Circulation 2003;107:223-5. [Crossref] [PubMed]
- Nanas JN, Matsouka C, Karageorgopoulos D, et al. Etiology of anemia in patients with advanced heart failure. J Am Coll Cardiol 2006;48:2485-9. [Crossref] [PubMed]
- Adlbrecht C, Kommata S, Hülsmann M, et al. Chronic heart failure leads to an expanded plasma volume and pseudoanaemia, but does not lead to a reduction in the body's red cell volume. Eur Heart J 2008;29:2343-50. [Crossref] [PubMed]
- Beard JL. Iron biology in immune function, muscle metabolism and neuronal functioning. J Nutr 2001;131:568S-579S; discussion 580S. [Crossref] [PubMed]
- Okonko DO, Grzeslo A, Witkowski T, et al. Effect of intravenous iron sucrose on exercise tolerance in anemic and nonanemic patients with symptomatic chronic heart failure and iron deficiency FERRIC-HF: a randomized, controlled, observer-blinded trial. J Am Coll Cardiol 2008;51:103-12. [Crossref] [PubMed]
- Anker SD, Comin Colet J, Filippatos G, et al. Ferric carboxymaltose in patients with heart failure and iron deficiency. N Engl J Med 2009;361:2436-48. [Crossref] [PubMed]
- Jankowska EA, Rozentryt P, Witkowska A, et al. Iron deficiency: an ominous sign in patients with systolic chronic heart failure. Eur Heart J 2010;31:1872-80. [Crossref] [PubMed]
- Toblli JE, Lombraña A, Duarte P, et al. Intravenous iron reduces NT-pro-brain natriuretic peptide in anemic patients with chronic heart failure and renal insufficiency. J Am Coll Cardiol 2007;50:1657-65. [Crossref] [PubMed]
- Bolger AP, Bartlett FR, Penston HS, et al. Intravenous iron alone for the treatment of anemia in patients with chronic heart failure. J Am Coll Cardiol 2006;48:1225-7. [Crossref] [PubMed]
- Usmanov RI, Zueva EB, Silverberg DS, et al. Intravenous iron without erythropoietin for the treatment of iron deficiency anemia in patients with moderate to severe congestive heart failure and chronic kidney insufficiency. J Nephrol 2008;21:236-42. [PubMed]
- Ponikowski P, van Veldhuisen DJ, Comin-Colet J, et al. Beneficial effects of long-term intravenous iron therapy with ferric carboxymaltose in patients with symptomatic heart failure and iron deficiency†. Eur Heart J 2015;36:657-68. [Crossref] [PubMed]
- Enko D, Moro T, Holasek S, et al. Branched-chain amino acids are linked with iron metabolism. Ann Transl Med 2020;8:1569. [Crossref] [PubMed]
- Rensvold JW, Ong SE, Jeevananthan A, et al. Complementary RNA and protein profiling identifies iron as a key regulator of mitochondrial biogenesis. Cell Rep 2013;3:237-45. [Crossref] [PubMed]
- Andrews PA. Disorders of iron metabolism. N Engl J Med 2000;342:1293-author reply 1294. [Crossref] [PubMed]
- Kell DB. Iron behaving badly: inappropriate iron chelation as a major contributor to the aetiology of vascular and other progressive inflammatory and degenerative diseases. BMC Med Genomics 2009;2:2. [Crossref] [PubMed]
- Shen W, Vatner DE, Vatner SF, et al. Progressive loss of creatine maintains a near normal DeltaG approximately (ATP) in transgenic mouse hearts with cardiomyopathy caused by overexpressing Gsalpha. J Mol Cell Cardiol 2010;48:591-9. [Crossref] [PubMed]
- Du Z, Shen A, Huang Y, et al. 1H-NMR-based metabolic analysis of human serum reveals novel markers of myocardial energy expenditure in heart failure patients. PLoS One 2014;9:e88102. [Crossref] [PubMed]
- Palmieri V, Roman MJ, Bella JN, et al. Prognostic implications of relations of left ventricular systolic dysfunction with body composition and myocardial energy expenditure: the Strong Heart Study. J Am Soc Echocardiogr 2008;21:66-71. [Crossref] [PubMed]
- Palmieri V, Bella JN, Arnett DK, et al. Associations of aortic and mitral regurgitation with body composition and myocardial energy expenditure in adults with hypertension: the Hypertension Genetic Epidemiology Network study. Am Heart J 2003;145:1071-7. [Crossref] [PubMed]
- Klip IT, Comin-Colet J, Voors AA, et al. Iron deficiency in chronic heart failure: an international pooled analysis. Am Heart J 2013;165:575-582.e3. [Crossref] [PubMed]
- Okonko DO, Mandal AK, Missouris CG, et al. Disordered iron homeostasis in chronic heart failure: prevalence, predictors, and relation to anemia, exercise capacity, and survival. J Am Coll Cardiol 2011;58:1241-51. [Crossref] [PubMed]
- Kasner M, Aleksandrov AS, Westermann D, et al. Functional iron deficiency and diastolic function in heart failure with preserved ejection fraction. Int J Cardiol 2013;168:4652-7. [Crossref] [PubMed]
- Jankowska EA, Kasztura M, Sokolski M, et al. Iron deficiency defined as depleted iron stores accompanied by unmet cellular iron requirements identifies patients at the highest risk of death after an episode of acute heart failure. Eur Heart J 2014;35:2468-76. [Crossref] [PubMed]
- van Veldhuisen DJ, Anker SD, Ponikowski P, et al. Anemia and iron deficiency in heart failure: mechanisms and therapeutic approaches. Nat Rev Cardiol 2011;8:485-93. [Crossref] [PubMed]
- Jankowska EA, Rozentryt P, Witkowska A, et al. Iron deficiency predicts impaired exercise capacity in patients with systolic chronic heart failure. J Card Fail 2011;17:899-906. [Crossref] [PubMed]
- Rangel I, Gonçalves A, de Sousa C, et al. Iron deficiency status irrespective of anemia: a predictor of unfavorable outcome in chronic heart failure patients. Cardiology 2014;128:320-6. [Crossref] [PubMed]
- Enjuanes C, Klip IT, Bruguera J, et al. Iron deficiency and health-related quality of life in chronic heart failure: results from a multicenter European study. Int J Cardiol 2014;174:268-75. [Crossref] [PubMed]
- Parikh A, Natarajan S, Lipsitz SR, et al. Iron deficiency in community-dwelling US adults with self-reported heart failure in the National Health and Nutrition Examination Survey III: prevalence and associations with anemia and inflammation. Circ Heart Fail 2011;4:599-606. [Crossref] [PubMed]
- Babitt JL, Lin HY. Molecular mechanisms of hepcidin regulation: implications for the anemia of CKD. Am J Kidney Dis 2010;55:726-41. [Crossref] [PubMed]
- Nemeth E, Ganz T. The role of hepcidin in iron metabolism. Acta Haematol 2009;122:78-86. [Crossref] [PubMed]
- Cook JD, Finch CA, Smith NJ. Evaluation of the iron status of a population. Blood 1976;48:449-55. [Crossref] [PubMed]
- Looker AC, Dallman PR, Carroll MD, et al. Prevalence of iron deficiency in the United States. JAMA 1997;277:973-6. [Crossref] [PubMed]
- Aquilani R, Opasich C, Verri M, et al. Is nutritional intake adequate in chronic heart failure patients? J Am Coll Cardiol 2003;42:1218-23. [Crossref] [PubMed]
- Liang J, Bai S, Xu D, et al. Effect of different doses of perindopril on myocardial energy expenditure in patients with heart failure following myocardial infarction. Nan Fang Yi Ke Da Xue Xue Bao 2012;32:1816-9; 1832. [PubMed]
- Chen PA, Xu ZH, Huang YL, et al. Increased serum 2-oxoglutarate associated with high myocardial energy expenditure and poor prognosis in chronic heart failure patients. Biochim Biophys Acta 2014;1842:2120-5. [Crossref] [PubMed]
- Ingwall JS. Energy metabolism in heart failure and remodelling. Cardiovasc Res 2009;81:412-9. [Crossref] [PubMed]
- Ardehali H, Sabbah HN, Burke MA, et al. Targeting myocardial substrate metabolism in heart failure: potential for new therapies. Eur J Heart Fail 2012;14:120-9. [Crossref] [PubMed]
- Huang ML, Lane DJ, Richardson DR. Mitochondrial mayhem: the mitochondrion as a modulator of iron metabolism and its role in disease. Antioxid Redox Signal 2011;15:3003-19. [Crossref] [PubMed]
- Galy B, Ferring-Appel D, Sauer SW, et al. Iron regulatory proteins secure mitochondrial iron sufficiency and function. Cell Metab 2010;12:194-201. [Crossref] [PubMed]
- Cairo G, Bernuzzi F, Recalcati S. A precious metal: Iron, an essential nutrient for all cells. Genes Nutr 2006;1:25-39. [Crossref] [PubMed]
- Turner LR, Premo DA, Gibbs BJ, et al. Adaptations to iron deficiency: cardiac functional responsiveness to norepinephrine, arterial remodeling, and the effect of beta-blockade on cardiac hypertrophy. BMC Physiol 2002;2:1. [Crossref] [PubMed]
- Tanne Z, Coleman R, Nahir M, et al. Ultrastructural and cytochemical changes in the heart of iron-deficient rats. Biochem Pharmacol 1994;47:1759-66. [Crossref] [PubMed]
- Naito Y, Tsujino T, Matsumoto M, et al. Adaptive response of the heart to long-term anemia induced by iron deficiency. Am J Physiol Heart Circ Physiol 2009;296:H585-93. [Crossref] [PubMed]
- Dong F, Zhang X, Culver B, et al. Dietary iron deficiency induces ventricular dilation, mitochondrial ultrastructural aberrations and cytochrome c release: involvement of nitric oxide synthase and protein tyrosine nitration. Clin Sci (Lond) 2005;109:277-86. [Crossref] [PubMed]
- Wish JB. Assessing iron status: beyond serum ferritin and transferrin saturation. Clin J Am Soc Nephrol 2006;1:S4-8. [Crossref] [PubMed]
- Pasricha SR, Flecknoe-Brown SC, Allen KJ, et al. Diagnosis and management of iron deficiency anaemia: a clinical update. Med J Aust 2010;193:525-32. [Crossref] [PubMed]
(English Language Editor: B. Draper)