A narrative review of multimodal imaging of white matter lesions in type-2 diabetes mellitus
Introduction
Diabetes mellitus (DM) is a complex and heterogeneous group of chronic metabolic diseases characterized by hyperglycemia which has become a serious public health concern. The incidence of DM has been increasing annually in recent decades, with an overall prevalence of up to 9.1% in adults worldwide (1,2). Type-2 DM (T2DM) is the most common type of DM and accounts for about 90% of all diabetic cases. Insulin resistance and decreased insulin response are the main features of T2DM (3). The most common complications caused by T2DM include diabetic neuropathy and vasculopathy, which includes macrovascular and microvascular complications. Due to the slow blood flow associated with such symptoms, small cerebral blood vessels are more susceptible to the effects of DM and cause early and widely distributed lesions that appear early in disease progression.
White matter lesion (WML) is a common type of cerebral small vessel disease, and is one of the chronic vascular complications of T2DM that can play an important role in stroke, cognitive decline, and age-related dysfunction (4). In general, many of these dysfunctions are associated with a pathogenesis of demyelination, axonal loss, neurogliosis, and so on (5). Previous studies have shown that T2DM is an independent risk factor for WML. It can affect white matter by influencing the regulation of cerebral blood flow and endothelial function, exacerbating atherosclerosis, reducing the number of oligodendrocytes and other mechanisms, ultimately leading to high signal intensity in white matter (6). The symptoms of WML are insidious and are risk factors for cognitive dysfunction, neuromental status, and functional deficits (7,8). Both clinical practice and animal modeling suggest that diabetes predisposes to cognitive decline leading to dementia, increasing the risk of dementia by nearly 1.5–2.5-fold (9-11). T2DM is associated with a risk of cognitive impairment and is manifested in impaired attention, motor and movement speed, executive function, and verbal memory. Recent studies have shown that the prevalence of WML has increased from approximately 5% in people aged 50 years to nearly 100% in people aged 90 years. This shows that the number of people with both T2DM and WML is also quite large (12). In recent years, the correlation between T2DM and WML has become a major hotspot in the field of neurological research. Recent reviews have also illustrated the link between T2DM and WML in that patients with T2DM have decreased white matter integrity, mainly in the corpus callosum, but there are fewer summaries of structural and functional changes in the brain of patients with T2DM and WML (13). While traditional computed tomography (CT) and conventional magnetic resonance imaging (MRI) imaging methods are common tools used to assess white matter (WM) fiber tracts associated with WML, the sensitivity of these techniques is low and makes it difficult to detect the presence and location of WML early in disease progression. Due to this limitation, more sensitive MRI techniques have recently emerged to assess early WM fiber integrity. In this paper, we reviewed the research progress made for evaluating WML in T2DM patients between 2008 and 2020 using multimodal MRI techniques to deepen clinicians’ understanding of WMLs in DM patients. We present the following article in accordance with the Narrative Review reporting checklist (available at https://dx.doi.org/10.21037/apm-21-3299).
Diabetes and white matter lesions
The pathogenesis of WMLs includes decreased vascular reactivity, leakage of the blood-brain barrier, decreased removal of toxic waste due to dysfunction of the lymphatic system, myelin damage, and reduced numbers of oligodendrocytes (14). According to the location of a WML, as seen via structural MRI, the lesion can be divided into either a deep or paraventricular WML. The location and morphology of a WML can reflect the etiology thereof and help distinguish putative WMLs of vascular origin from other causes such as multiple sclerosis. Most T2DM patients exhibit fusion WMLs with higher eccentricity and have more WMLs than healthy individuals (15). The MRI of a woman with T2DM in her 60 s is shown in Figure 1.
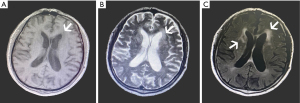
The presence of T2DM is an independent risk factor for WML, which affects the tissue by inducing endothelial cell dysfunction and impaired vasodilation. These processes accelerate arteriosclerosis and cause inflammation, oxidative stress, blood flow dysregulation, and so on, which ultimately lead to high WM signal intensity (6). Some findings have shown that a history of diabetes and high blood glucose can predict WML progression (16). Moreover, pre-DM conditions are also associated with structural brain abnormalities that further exacerbate T2DM. In fact, structural brain abnormalities already appear in the pre-DM period, in which they are significantly associated with larger WMLs and smaller WM volume. This suggests that the treatment of early dysglycemia may help prevent brain diseases (17).
At present, clinical studies examining T2DM and WML include structural imaging, molecular neuroimaging, neuropsychology, and so on. However, it is thought that multimodal MRI can more accurately and comprehensively evaluate WML in T2DM patients at the structural and functional levels. In clinical practice, commonly used multimodal MRI techniques include diffusion tensor imaging (DTI), diffusion kurtosis imaging (DKI), neurite orientation dispersion and density imaging (NODDI), diffusion spectrum imaging (DSI), functional magnetic resonance imaging (fMRI), and magnetic resonance spectroscopy (MRS).
Structural MRI
DTI
The DTI enables the detailed visualization of WM fiber tracts and their individual connections to reflect the microstructural integrity of brain tissue. This is done by measuring the differences in water molecule diffusion in biological tissues and fibers that constitute WM. In this sense, DTI has been shown to better define anatomical targets compared to CT and conventional MRI (18). It can also detect WM changes prior to than the manifestation of their clinical symptoms in patients with T2DM, which is considered an important biomarker for WMLs and a predictor of disease progression. The parameters of DTI include fractional anisotropy (FA), mean diffusivity (MD), axial diffusivity (AD), and radial diffusivity (RD). The FA parameter is highly sensitive to changes in WM microarchitecture, and can describe the extent, direction, and scale of water molecule diffusion in biological tissues. In general, higher FA values indicate higher WM integrity. The MD value describes the water molecule diffusion velocity, and a higher MD value indicates less restricted water molecule diffusion. Finally, AD and RD reflect the integrity of axons and the myelin sheath, respectively. Overall, reduced FA and increased MD, AD, and RD values reflects impaired WM microarchitecture.
A systematic review of 29 DTI studies showed that there was widespread brain microstructural impairment in the WM, frontal, temporal, and parietal lobes, as well as the cerebellum, corpus callosum, and thalamus (19). A recent study also showed that there was a reduced FA and an increased MD value in the corpus callosum, anterior limb of the internal capsule, posterior thalamic radiation, superior corona radiata, right lentiform nucleus, right superior longitudinal fasciculus, and left middle frontal gyrus in T2DM patients with peripheral microvascular complications. Similarly, these patients exhibited a reduced FA and increased MD value in the corpus callosum, fornix, right lentiform nucleus, middle cerebral peduncle, right superior longitudinal fasciculus, right posterior thalamic radiation, and left middle frontal gyrus compared with T2DM patients without peripheral microvascular complications. This study also reported that there was no significant difference in FA values between healthy controls and T2DM patients without peripheral microvascular complications (20). Zhang et al. (21) found that the underlying organization of T2DM brain networks can be extracted by DTI and that changes in node characteristics indicate reduced connectivity in several brain regions. These alterations demonstrate the presence of WM structural network changes and the association between structural properties and cognitive status in patients with T2DM. Li et al. (22) also performed DTI in rat models of DM and found that thalamic FA values were lower in T2DM rats compared to normal controls. The results of our previous study showed that DTI of the brains of patients with T2DM showed impaired WM microstructural integrity, as shown in Figure 2.
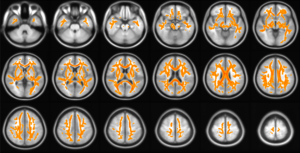
The above studies suggest that DTI is a powerful technique that emphasizes the disruption of structural WM networks in T2DM patients. These changes make it feasible to assess WMLs in patients with T2DM and to detect damage to the WM microarchitecture before clinical diagnosis. However, some methodological (image resolution), physiological (alignment and density of axons and complex fibrous structures), and pathological changes may cause confounding changes in DTI metrics and therefore require additional and complementary techniques.
DKI
The DKI approach is an extension of the popular DTI technique that takes into account major deviations from Gaussian diffusion which may result from many effects related to microarchitecture and compartmentalization in biological tissues. Therefore, DKI exhibits increased sensitivity to microstructural alterations in biological tissues compared with DTI. In biological tissues, extremely complex environments and structures affect water molecule diffusion, in which case the diffusion displacement distribution appears in a non-Gaussian form. The DKI has been proposed to characterize such non-Gaussian diffusion effects (23) and provides diffusion metrics compatible with DTI along with additional kurtosis metrics, including mean kurtosis (MK), axial kurtosis (AK), radial kurtosis (RK), and kurtosis anisotropy (KA). In general, these kurtosis values quantify the degree of diffusion limitation and tissue complexity, with the MK metric being the most widely used. Reduced MK values indicate impaired microarchitecture characteristics, such as the thinning of fiber bundles or axons and reduced tissue complexity. By contrast, reduced RK values reflect decreased axonal membrane permeability, and reduced AK values are associated with cytoarchitectural defects along the axis of WM fibers.
Several DKI studies have indicated the existence of WML in patients with T2DM. Xie et al. (24) found a reduced FA value in the right frontal WM and splenium of the corpus callosum, an increased MD in the frontal WM, right temporal lobe, left external capsule, splenium of the corpus callosum, and pons, and a reduced MK, RK, and AK value in the splenium of the corpus callosum and pons in patients with T2DM using DKI. In addition, it has been shown that microarchitectural abnormalities in WM occur before cognitive decline and can be used as neuroimaging markers to predict early cognitive impairment in patients with T2DM. Xiong et al. (25) found that 29.6%, 30.4%, 35.4%, 10.5%, and 26.0% of voxels in WM regions of T2DM patients exhibited a reduced FA, MD, MK, AK, and RK value, respectively. Detailed analysis showed that a reduced MK value is likely caused by a reduced RK value, rather than a reduced AK value. In an atlas-based analysis, MK predicted more areas of altered WM microarchitecture than FA or MD. Through the above DKT and DTI comparative study, it was again demonstrated that DKI can detect more WM microstructural changes in T2DM patients compared with DTI indicators. This work provides valuable information for studying the pathology of diabetic encephalopathy and may lead to better imaging biomarkers to monitor the progression of the disease. Furthermore, MK was shown to be related to disease severity and impaired neuropsychological function in T2DM patients, and has the potential to assess cognitive impairment and predict disease progression in patients with T2DM.
All the above DKI studies indicate that WML exists in T2DM patients and that DKI is sensitive to microstructural changes in biological tissues. However, the sample size of the current research was relatively small, and the results obtained by different magnetic resonance equipment and post-processing software may induce variability across studies and patient populations. Therefore, the conclusions drawn have certain limitations. It is therefore necessary to establish unified scanning parameters and measurement schemes, and to expand the sample size to further verify the value of DKI in the diagnosis of WML in patients with T2DM and to apply it in clinical practice.
NODDI
The NODDI technique is an in vivo diffusion MRI method used to detect tissue compartments and to distinguish 3 microarchitectural environments: intracellular, extracellular, and cerebrospinal fluid. This technique is also used to quantify neurite density index (NDI) and orientation dispersion index (ODI) of brain tissues (26). Importantly, NODDI measurements are biologically significant and can improve the shortcomings of DTI affected by nerve density or spatial changes. The microstructural indices of NODDI include: (I) intracellular volume fraction (Vic), which quantifies neurite density; (II) ODI, which characterizes the dispersion characteristics of neurites and reflects the directional distribution of fibers in WM; (III) intra-cellular volume fraction (ICVF), which is directly related to neuronal density; (IV) degree parameter, which measures the degree of direction dispersion and is correlated with ODI analysis in a nonlinear manner; and (V) volume fraction of the isotropic compartment (ISO), that is, isotropic freely diffusing water molecule volume fraction in voxels (27,28).
Wang et al. (29) compared the sensitivity of NODDI with DTI and DKI, and found that NODDI can effectively assess microstructural changes in brain tissue of ischemic stroke patients with increased sensitivity. This work also showed that this advanced diffusion technique could further elucidate the underlying pathological mechanism of ischemia. Xiong et al. (30) used the NODDI approach to characterize brain microstructural alterations in T2DM patients with mild cognitive impairment, and showed that 2.29% and 2.02% of WM regions exhibited a reduced FA and Vic, respectively, between cognitively normal T2DM patients and healthy controls. Nevertheless, in T2DM patients with mild cognitive impairment, a considerable number of WM regions exhibited a reduced FA (38.38%) and Vic (34.64%) value. However, the ODI values were not significantly different among the 3 groups. In addition, this work found that a reduced Vic value in the genu of the corpus callosum and thalamus correlated with glycosylated hemoglobin levels, disease duration, and neuropsychological scores.
The above study demonstrated that NODDI can aid in detecting microstructural changes in WM and provide information on diabetic encephalopathy in patients with cognitive impairment. However, NODDI requires a high b value, which can reduce the signal-to-noise ratio of the acquired images, and thus induce higher requirements for the hardware and software configuration of MRI scanners. This results in long scan times, which may increase the burden on the patients. Such shortcomings have led to slow adoption of this technology in clinical practice in China. Nevertheless, it is believed that continuous optimization and improvement of NODDI protocols will in the future provide more effective information for clinicians regarding the early diagnosis and treatment of neurological diseases, disease progression, and prognosis.
DSI
The low spatial resolution of DTI results in the inaccurate detection of low anisotropy in monomers and fiber direction in various complex structural brain regions (31). In this sense, a single fiber detected by DTI may actually include multiple fibers or fiber crossings. The DSI technique exhibits a higher spatial resolution than DTI and can therefore better detect and delineate the fiber microstructure in individual voxels. Generalized fractional anisotropy (GFA) is a DSI index equivalent to the FA index of DTI that characterizes the microstructural integrity of WM fiber tracts. The GFA ranges between 0 and 1, and a higher GFA value indicates stronger structural integrity.
Zhang et al. (32) found that the GFA values of the left uncinate fasciculus and right superior cingulum bundle were significantly reduced in T2DM patients compared with healthy controls. Meanwhile, the GFA value of the right superior cingulum bundle was negatively correlated with word fluency test scores and blood cholesterol levels.
The above study indicated that DSI tractography can assess the microstructural integrity of WM bundles in T2DM and is related to clinical cognitive scores and related biochemical parameters. This is notable as such a tool can help predict early WM abnormalities in T2DM. Not only is DSI a non-invasive examination, but it also strengthens the study and diagnosis of the pathophysiological mechanism of the nervous system by accurately displaying the complex fibrous structure. However, there have been few studies focusing on the application of DSI to WML in T2DM patients, so evidence is still lacking.
Structural MRI can also describe structure of WML and assess the diseased brains by quantifying brain structure characteristics. Various problems with this method exist in clinical application, such as how to select the appropriate b value, how to reduce the scanning time, and the lack of a mature gold-standard protocol that can be used in clinical trials. Therefore, the application of structural MRI to assessing WMLs of T2DM patients is still in the early stage. However, based on the sensitivity and specificity to brain microstructures, structural MRI may in the future be more widely used in clinical practice with the more in-depth study of WML of T2DM patients. Such an approach would provide guidance for the diagnosis, treatment, follow-up efficacy, and prognosis of T2DM patients in clinical practice.
fMRI
Changes in cerebral blood oxygen levels can be detected by changes in magnetic resonance signals over time and reflect the activity of brain regions. The fMRI is designed to show local time-varying changes in brain metabolism and has the advantages of exhibiting extensive practicability, low cost, and good spatial resolution. Functional correlations between different and distant brain regions can be studied using resting-state fMRI; however, because it involves complex physics and data analysis, little progress has been made from research environments to daily clinical use (33).
A recent study aimed to investigate the aberration of spontaneous brain activity and synchrony abnormalities in patients with T2DM. This work showed that T2DM patients exhibited a higher amplitude of low frequency fluctuations in the left middle occipital gyrus, and lower functional connectivity between the left middle occipital gyrus and the left caudate nucleus and left inferior parietal gyrus. Nevertheless, no significant correlation was observed (34). At present, DTI and fMRI fusion techniques are increasingly favored by clinicians because such a combination can establish functional connectivity network maps of activated regions and facilitate the interpretation of the relationship between structure and function, which has largely driven the expansions of functional network research. Hoogenboom et al. (35) studied T2DM patients using DTI and fMRI techniques, and found that T2DM patients exhibit WM abnormalities associated with disrupted functional connectivity that underlie both structural and functional connectivity. Early detection of brain abnormalities in the preclinical stage can be useful for developing preventive interventions to slow the progression of neuropathology and thereby mitigate clinical symptoms such as cognitive decline. Qi et al. (36) demonstrated that T2DM patients with mild cognitive impairment had abnormal functional connectivity patterns and decreased WM integrity. These patients also exhibited stronger functional connectivity in the left precuneus and weaker functional connectivity in the left calcarine, as well as decreased integrity of the left cingulate bundle and bilateral uncinate fasciculus. These characteristics could potentially serve as dementia biomarkers for the early detection of elderly individuals with T2DM.
It can be inferred from the above studies that the combination of various MRI techniques has promoted the deepening of functional network research. In general, due to the existence of compensatory mechanisms in the early stage of T2DM, the changes in functional networks cannot be fully explained. Therefore, the in-depth study of the mechanism of WML occurrence and development in T2DM patients in the future needs to involve a multimodal MRI approach.
MRS
In MRS, MRI is combined with spatial coding methods to enable the mapping of spectral information in a non-invasive manner. Currently, MRS is the only technique that can visualize and quantitatively analyze metabolic changes associated with disease or injury in living tissues, with 1H-MRS being the most widely used technique worldwide. Most clinical studies using MRS have focused on the detection of significant spectral components, namely N-Acetyl aspartic acid (NAA), creatine (Cr), Choline (Cho) compounds, and Myo-inositol (MI) (37), to reflect the metabolism in individual brain regions. The decreased NAA and MI values indicate that neurons are lost and glial cells are damaged. Energy metabolism disorder and WM demyelination often occur in the WM of WML patients who are in a state of chronic ischemia. Evaluation of these patients with MRS shows that the peak value of NAA is reduced and that the concentration of Cho is increased compared to healthy controls.
Our previous study found that patients with T2DM exhibited reduced NAA and NAA/MI values in the right prefrontal cortex, an increased Cho in the right prefrontal WM, and an increased MI in the bilateral prefrontal cortex. In T2DM patients, there was a negative correlation between the NAA value and glycated hemoglobin concentration in the right prefrontal cortex. These results suggested that recent blood glucose levels can affect changes in brain metabolites, and that the reasonable control of blood glucose can effectively delay brain lesions caused by T2DM (38). Cao et al. (39) studied the possible metabolic changes in the frontal cortex and parietal WM in patients with diabetic hypertension (DHT). The authors found that the NAA/Cr ratio and Cho/Cr ratio in the prefrontal cortex, and the NAA/Cr ratio in the left parietal WM were significantly lower in DHT patients compared to healthy controls. Therefore, metabolic disorders in the frontal cortex and parietal WM occur in DHT patients, and the ratios of NAA/Cr and Cho/Cr are potential metabolic indicators of brain damage in DHT patients (39). In their meta-analysis of 10 studies, Wu et al. (40), found that metabolite levels were altered in different regions of the brain in T2DM patients compared with healthy controls. Specifically, an increase in MI level in frontal WM and a decrease in NAA/Cr ratios in the frontal and lentiform nuclei were found in these patients. In summary, the results of multiple studies have suggested that MI and Cho levels are increased, and that NAA, NAA/Cr, Cho/Cr, and NAA/Cho values are reduced in the WM of T2DM patients.
As these previous studies have demonstrated, MRS can reflect the metabolic information of cells at the molecular level and has great potential for clinical application. However, there is a lack of horizontal and longitudinal comparative studies using this technique. The standardization of acquisition protocols and the application of high-field MRI will greatly improve the availability and clinical utility of MRS in the future.
Functional MRI methods in general are noninvasive approaches to detect WML in patients with T2DM. These methods are characterized by high reproducibility, high sensitivity, and high acceptance across the field. Functional approaches are expected to be used to characterize brain dysfunction and thus provide a new method for the diagnosis and treatment of neuropsychiatric diseases. However, due to the small sample sizes, insufficient statistical significance, and inaccurate and unreliable brain image data analysis methods, the field is temporarily unable to use image indicators as the basis for diagnosis and treatment. The complex post-processing and long examination time associated with these techniques also limit the application in clinical practice.
The abovementioned studies have described the various MRI methods used to study WML in T2DM patients in recent years. Each single-modality MRI technique has its own imaging characteristics and advantages, and can provide a specific image biomarker with diagnostic value for identifying WML in patients with early T2DM. However, all of the techniques also have their own limitations. Therefore, to understand the complex and diverse neurological mechanisms of WMLs in T2DM patients, multimodal MRI techniques must be used to obtain comprehensive evaluations from multiple perspectives. However, it is difficult to directly compare which approaches can best identify differential features between healthy and diseased individuals due to the differences in MRI modalities. Each MRI modality provides different imaging features, and various studies have utilized different feature selection methods to predict disease state. Different classification methods should therefore be compared to define an optimal protocol within the multimodal MRI framework to provide a more rational diagnostic approach for WML in patients with T2DM.
Summary and future prospects
Both T2DM and WMLs are highly prevalent worldwide. The WML in patients with T2DM exhibit changes in cerebral perfusion, functional networks, nerve fiber structure, and brain tissue metabolism compared to healthy individuals. The presence of WML can be an early marker of brain injury in T2DM patients; however, the clinical manifestations of these lesions are insidious and often difficult to detect. The long-term presence and progression of lesions can cause adverse consequences and therefore, the early detection of brain abnormalities in the preclinical stage is imperative for the implementation of preventive measures. Such early interventions may help to mitigate complications associated with WMLs by slowing the progression of neuropathy.
Multimodal MRI provides multi-parameter information about T2DM patients using non-invasive and sensitive imaging techniques that can be useful in clinical practice. Specifically, the various MRI approaches can reflect the microscopic damage of nerve myelin structure and the pathological changes of neuronal metabolic function of WM in patients with T2DM. Multimodal MRI provides a significant opportunity to study the pathogenesis and early diagnosis of WML in T2DM patients. The following directions should be emphasized in the future to enable practical clinical use. First, multicenter, large-sample, and prospective longitudinal studies must be carried out to comprehensively explore the process of disease occurrence, development, and outcome. Second, it is necessary to further improve and standardize multimodal MRI processing methods to obtain reliable and accurate imaging data that can be easier to use in clinical practice. Third, the combination of multimodal MRI technology, neuropsychological evaluations, serum or cerebrospinal fluid content analysis, and other biological markers should be expanded to comprehensively describe the complex interaction between neuro-vascular-tissue function and disease. These techniques can all contribute to identifying an objective basis for the pathophysiological mechanism of WML in T2DM and can help to establish a foundation for the early treatment and improved prognosis these patients.
Acknowledgments
The authors would like to extend their sincere gratitude to their supervisor, Changhao Yin, who made useful suggestions for the paper. We are deeply grateful for his help in completing our paper.
Funding: This work was supported by the National Natural Science Foundation of China (No.: 81771795), Project of Heilongjiang Provincial Finance Department for Excellent Young Talents Training, Basic Scientific Research Business Fee Project of Education Department of Heilongjiang Province (Key grant) (No. 2018-KYYWFMY-0002).
Footnote
Reporting Checklist: The authors have completed the Narrative Review reporting checklist. Available at https://dx.doi.org/10.21037/apm-21-3299
Conflicts of Interest: All authors have completed the ICMJE uniform disclosure form (available at https://dx.doi.org/10.21037/apm-21-3299). The authors have no conflicts of interest to declare.
Ethical Statement: The authors are accountable for all aspects of the work in ensuring that questions related to the accuracy or integrity of any part of the work are appropriately investigated and resolved.
Open Access Statement: This is an Open Access article distributed in accordance with the Creative Commons Attribution-NonCommercial-NoDerivs 4.0 International License (CC BY-NC-ND 4.0), which permits the non-commercial replication and distribution of the article with the strict proviso that no changes or edits are made and the original work is properly cited (including links to both the formal publication through the relevant DOI and the license). See: https://creativecommons.org/licenses/by-nc-nd/4.0/.
References
- Padilla-Martínez F, Collin F, Kwasniewski M, et al. Systematic Review of Polygenic Risk Scores for Type 1 and Type 2 Diabetes. Int J Mol Sci 2020;21:1703. [Crossref] [PubMed]
- Hu C, Jia W. Diabetes in China: Epidemiology and Genetic Risk Factors and Their Clinical Utility in Personalized Medication. Diabetes 2018;67:3-11. [Crossref] [PubMed]
- Pasupuleti VR, Arigela CS, Gan SH, et al. A Review on Oxidative Stress, Diabetic Complications, and the Roles of Honey Polyphenols. Oxid Med Cell Longev 2020;2020:8878172. [Crossref] [PubMed]
- Li Q, Yang Y, Reis C, et al. Cerebral Small Vessel Disease. Cell Transplant 2018;27:1711-22. [Crossref] [PubMed]
- Wardlaw JM, Smith C, Dichgans M. Small vessel disease: mechanisms and clinical implications. Lancet Neurol 2019;18:684-96. [Crossref] [PubMed]
- Sun J, Xu B, Zhang X, et al. The Mechanisms of Type 2 Diabetes-Related White Matter Intensities: A Review. Front Public Health 2020;8:498056. [Crossref] [PubMed]
- Puzo C, Labriola C, Sugarman MA, et al. Independent effects of white matter hyperintensities on cognitive, neuropsychiatric, and functional decline: a longitudinal investigation using the National Alzheimer's Coordinating Center Uniform Data Set. Alzheimers Res Ther 2019;11:64. [Crossref] [PubMed]
- Hu HY, Ou YN, Shen XN, et al. White matter hyperintensities and risks of cognitive impairment and dementia: A systematic review and meta-analysis of 36 prospective studies. Neurosci Biobehav Rev 2021;120:16-27. [Crossref] [PubMed]
- Fiore V, De Rosa A, Falasca P, et al. Focus on the Correlations between Alzheimer's Disease and Type 2 Diabetes. Endocr Metab Immune Disord Drug Targets 2019;19:571-9. [Crossref] [PubMed]
- Geijselaers SLC, Sep SJS, Stehouwer CDA, et al. Glucose regulation, cognition, and brain MRI in type 2 diabetes: a systematic review. Lancet Diabetes Endocrinol 2015;3:75-89. [Crossref] [PubMed]
- Liu Y, Tan W, Chen C, et al. A Review of the Application of Virtual Reality Technology in the Diagnosis and Treatment of Cognitive Impairment. Front Aging Neurosci 2019;11:280. [Crossref] [PubMed]
- Chatterjee S, Mudher A. Alzheimer's Disease and Type 2 Diabetes: A Critical Assessment of the Shared Pathological Traits. Front Neurosci 2018;12:383. [Crossref] [PubMed]
- Wang DQ, Wang L, Wei MM, et al. Relationship Between Type 2 Diabetes and White Matter Hyperintensity: A Systematic Review. Front Endocrinol (Lausanne) 2020;11:595962. [Crossref] [PubMed]
- Joutel A, Chabriat H. Pathogenesis of white matter changes in cerebral small vessel diseases: beyond vessel-intrinsic mechanisms. Clin Sci (Lond) 2017;131:635-51. [Crossref] [PubMed]
- de Bresser J, Kuijf HJ, Zaanen K, et al. White matter hyperintensity shape and location feature analysis on brain MRI; proof of principle study in patients with diabetes. Sci Rep 2018;8:1893. [Crossref] [PubMed]
- Gouw AA, van der Flier WM, Fazekas F, et al. Progression of white matter hyperintensities and incidence of new lacunes over a 3-year period: the Leukoaraiosis and Disability study. Stroke 2008;39:1414-20. [Crossref] [PubMed]
- van Agtmaal MJM, Houben AJHM, de Wit V, et al. Prediabetes Is Associated With Structural Brain Abnormalities: The Maastricht Study. Diabetes Care 2018;41:2535-43. [Crossref] [PubMed]
- Tohyama S, Walker MR, Sammartino F, et al. The Utility of Diffusion Tensor Imaging in Neuromodulation: Moving Beyond Conventional Magnetic Resonance Imaging. Neuromodulation 2020;23:427-35. [Crossref] [PubMed]
- Sanjari Moghaddam H, Ghazi Sherbaf F, Aarabi MH. Brain microstructural abnormalities in type 2 diabetes mellitus: A systematic review of diffusion tensor imaging studies. Front Neuroendocrinol 2019;55:100782. [Crossref] [PubMed]
- Zhuo Y, Fang F, Lu L, et al. White matter impairment in type 2 diabetes mellitus with and without microvascular disease. Neuroimage Clin 2019;24:101945. [Crossref] [PubMed]
- Zhang Y, Cao Y, Xie Y, et al. Altered brain structural topological properties in type 2 diabetes mellitus patients without complications. J Diabetes 2019;11:129-38. [Crossref] [PubMed]
- Li J, Guo Y, Li Q, et al. Presence of White Matter Lesions Associated with Diabetes-Associated Cognitive Decline in Male Rat Models of Pre-Type 2 Diabetes. Med Sci Monit 2019;25:9679-89. [Crossref] [PubMed]
- Zhu LH, Zhang ZP, Wang FN, et al. Diffusion kurtosis imaging of microstructural changes in brain tissue affected by acute ischemic stroke in different locations. Neural Regen Res 2019;14:272-9. [Crossref] [PubMed]
- Xie Y, Zhang Y, Qin W, et al. White Matter Microstructural Abnormalities in Type 2 Diabetes Mellitus: A Diffusional Kurtosis Imaging Analysis. AJNR Am J Neuroradiol 2017;38:617-25. [Crossref] [PubMed]
- Xiong Y, Sui Y, Zhang S, et al. Brain microstructural alterations in type 2 diabetes: diffusion kurtosis imaging provides added value to diffusion tensor imaging. Eur Radiol 2019;29:1997-2008. [Crossref] [PubMed]
- Lynch KM, Cabeen RP, Toga AW, et al. Magnitude and timing of major white matter tract maturation from infancy through adolescence with NODDI. Neuroimage 2020;212:116672. [Crossref] [PubMed]
- Deligianni F, Carmichael DW, Zhang GH, et al. NODDI and Tensor-Based Microstructural Indices as Predictors of Functional Connectivity. PLoS One 2016;11:e0153404. [Crossref] [PubMed]
- Merluzzi AP, Dean DC 3rd, Adluru N, et al. Age-dependent differences in brain tissue microstructure assessed with neurite orientation dispersion and density imaging. Neurobiol Aging 2016;43:79-88. [Crossref] [PubMed]
- Wang Z, Zhang S, Liu C, et al. A study of neurite orientation dispersion and density imaging in ischemic stroke. Magn Reson Imaging 2019;57:28-33. [Crossref] [PubMed]
- Xiong Y, Zhang S, Shi J, et al. Application of neurite orientation dispersion and density imaging to characterize brain microstructural abnormalities in type-2 diabetics with mild cognitive impairment. J Magn Reson Imaging 2019;50:889-98. [Crossref] [PubMed]
- Lee MB, Kim YH, Jahng GH, et al. Angular resolution enhancement technique for diffusion-weighted imaging (DWI) using predicted diffusion gradient directions. Neuroimage 2018;183:836-46. [Crossref] [PubMed]
- Zhang Q, Xiao Y, Lin L, et al. Diffusion spectrum imaging in white matter microstructure in subjects with type 2 diabetes. PLoS One 2018;13:e0203271. [Crossref] [PubMed]
- McClung Pasqualino H. Functional MRI: Basic Principles and Emerging Clinical Applications for Anesthesiology and the Neurologic Sciences, 1st Edition. Anesthesiology 2020. [Epub ahead of print].
- Liu D, Duan S, Wei P, et al. Aberrant Brain Spontaneous Activity and Synchronization in Type 2 Diabetes Mellitus Patients: A Resting-State Functional MRI Study. Front Aging Neurosci 2020;12:181. [Crossref] [PubMed]
- Hoogenboom WS, Marder TJ, Flores VL, et al. Cerebral white matter integrity and resting-state functional connectivity in middle-aged patients with type 2 diabetes. Diabetes 2014;63:728-38. [Crossref] [PubMed]
- Qi D, Wang A, Chen Y, et al. Default Mode Network Connectivity and Related White Matter Disruption in Type 2 Diabetes Mellitus Patients Concurrent with Amnestic Mild Cognitive Impairment. Curr Alzheimer Res 2017;14:1238-46. [Crossref] [PubMed]
- Maudsley AA, Andronesi OC, Barker PB, et al. Advanced magnetic resonance spectroscopic neuroimaging: Experts' consensus recommendations. NMR Biomed 2021;34:e4309. [Crossref] [PubMed]
- Li S, Yin C, Zhao W, et al. Application of Hydrogen Proton Magnetic Resonance Technology Combined with Brain Neurometabolite Analysis in the Treatment of Cognitive Impairment Caused by Type 2 Diabetes Mellitus. World Neurosurg 2020;138:654-62. [Crossref] [PubMed]
- Cao Z, Ye BD, Shen ZW, et al. 2D-1H proton magnetic resonance spectroscopic imaging study on brain metabolite alterations in patients with diabetic hypertension. Mol Med Rep 2015;11:4232-8. [Crossref] [PubMed]
- Wu GY, Zhang Q, Wu JL, et al. Changes in cerebral metabolites in type 2 diabetes mellitus: A meta-analysis of proton magnetic resonance spectroscopy. J Clin Neurosci 2017;45:9-13. [Crossref] [PubMed]