Systematic review and meta-analysis of arterial spin-labeling imaging to distinguish between glioma recurrence and post-treatment radiation effect
Introduction
Glioma is a tumor that originates from glial cells. It is one of the most common primary intracranial tumors, and its incidence rate is increasing each year (1). High-grade gliomas, especially glioblastomas, have a high incidence rate, high recurrence rate after surgery, a high mortality rate, and a low cure rate (1,2). Currently, the standard treatment plan for glioma is surgery, followed by comprehensive treatment, such as radiotherapy and chemotherapy. Postoperative radiotherapy can prolong the overall survival of patients with high-grade glioma (3). Craniocerebral radiation brain injury, including common pseudo-progression and radiation necrosis, is a common complication after the comprehensive treatment of glioma (3-5).
The mechanism of radiation necrosis is not very clear. It may be related to factors such as direct damage to brain tissue by radiation, secondary brain tissue ischemia and necrosis caused by vascular damage, autoimmune response, and free radical damage (6-9). Most scholars believe that the above-mentioned mechanisms are not independent of each other, but that these multi-factors work together (9-11). Pseudo-progression is a treatment-related response that is not related to tumor progression, and its incidence is related to the dose of radiotherapy. The occurrence of pseudo-progression is more common within 6 months of the end of treatment (10,11). Most patients have no clinical symptoms and signs. Compared to the traditional concept of radiation necrosis, pseudo-progression can be reduced or kept stable even if it is not treated.
Distinguishing between tumor recurrence and related changes caused by radiotherapy is essential for the clinical management of patients with high-grade glioma, as it informs decisions such as whether second-line treatment drugs should be used and whether reoperation is necessary. The abundance of tumor neovascularization is an important indicator that determines the grade of tumor tissue, the degree of benign and malignant tumors, and the recurrence of tumors after chemotherapy or radiotherapy. Perfusion reflects the local microcirculation level of the lesion and can truly reflect the neovascularization of the tumor (12,13). Magnetic resonance perfusion weighted imaging (MRPWI) is an important non-invasive evaluation method for quantifying the degree of tumor angiogenesis, and it provides important reference values for distinguishing the enhancement of glioma after treatment or tumor recurrence (9). Arterial spin-labeling (ASL) is a perfusion imaging method that uses labeled blood hydrogen protons to measure the flow rate, does not require the injection of exogenous contrast agents, and can be used to assess tumor angiogenesis (10,14). ASL can accurately reflect and monitor brain perfusion information, and can be used as a supplementary examination for patients with impaired renal function who require long-term follow-up care. Currently, the use of ASL to assess glioma recurrence and post-treatment radiation effect (PTRE) is limited, and the sample sizes of individual studies have been very small (15,16). As various scanning techniques have been used in heterogeneous patient groups, it is difficult to draw clear conclusions to guide clinical practice.
This meta-analysis was conducted to compare the difference between ASL-derived parameters in tumor recurrence and PTRE in gliomas patients to assess whether ASL imaging can effectively distinguish between recurrent tumors and PTRE after radiotherapy. We present the following article in accordance with the PRISMA reporting checklist (available at https://dx.doi.org/10.21037/apm-21-3319).
Methods
Search strategy
Databases, including Medline, Cochrane Library, PubMed, Web of Science, Embase, China National Knowledge Infrastructure (CNKI), and Wan Fang, were searched to retrieve clinical studies on the use of ASL imaging to diagnose glioma recurrence and radiation brain injury. The search deadline was August 31, 2021. The search languages were limited to English and Chinese. The key search terms included: “gliomas”, “glioblastoma”, “GBM”, “astrocytoma”, “radiation injury”, “radiation necrosis”, “pseudo-progression”, “treatment effect”, “radiation change”, “post-treatment radiation effect” and “PTRE”.
Inclusion criteria
To be eligible for inclusion in the meta-analysis, the studies had to meet the following inclusion criteria: (I) adopt a study design in which ASL was used as the clinical diagnostic test to distinguish between the recurrence of glioma and radiation brain injury; (II) comprise patients whose diagnoses of glioma had been confirmed by surgery and pathology, who had received local or whole brain radiotherapy after surgery, and in whom abnormal enhancement lesions in the operation area were observed; (III) evaluate the diagnostic method of ASL; (IV) use the second surgical pathological results or clinical follow-up results as the “gold standard” to diagnose glioma recurrence or PTRE; and (V) include an evaluation index from which the diagnostic test data could be extracted based on the original literature data, including the number of true positives, false negatives, false positives, and true negatives. For repeated publications, recent articles were selected.
Exclusion criteria
Articles were excluded from the meta-analysis if they met any of the following exclusion criteria: (I) comprised a review, abstract, case report, or conference paper; (II) did not use a “gold standard” method to diagnose the study cases; (III) contained incomplete data from which the diagnostic data could not be extracted; (IV) were a duplicate; and/or (V) included <10 cases.
Paper screening and data extraction
Two reviewers independently screened the articles according to the set literature inclusion and exclusion criteria, and extracted the basic data from the articles. If a disagreement arose, the reviewers engaged in further discussion until a consensus was reached. The data required for the included literature extraction included basic information (e.g., first author, publication year, sample size, and research method), and patient information (e.g., age, treatment method, and diagnosis method).
Quality assessment
The quality of the literature was evaluated using the Cochrane risk-of-bias assessment tool. Evaluations were made according to the standards of “low risk”, “unclear risk”, and “high risk”. The 2 reviewers independently evaluated the quality of the included studies. Both reviewers had received statistical and relevant professional training, and both had the ability to independently complete quality evaluations. If any differences arose, the reviewers discussed the issue and resolved it between themselves, or asked a 3rd party to re-evaluate their assessments.
Statistical analysis
The general inverse variance method in RevMan 5.3 software (The Cochrane Collaboration, 2014) was used to merge the effect sizes. The categorical variables were analyzed using relative risk (RR). The continuous data were analyzed using mean difference (MD) or standardized mean difference (SMD), and the 95% confidence interval (CI) of each effect size was calculated. The I2 test was used to test the heterogeneity between studies. If the heterogeneity between studies was small (P>0.05, I2<50%), the fixed-effects model was used to combine the effect sizes. If there was obvious heterogeneity (P≤0.05, I2≥50%), the random-effects model was used to combine the effect sizes. And a sensitivity analysis was carried out according to the Cochrane systematic review method. A subgroup analysis was performed on the outcome indicators of the study. When 5 or more studies are available, a funnel chart asymmetry test was used to assess publication bias.
Results
Search results and study characteristics
Eight hundred and twenty six articles were retrieved (423 in English and 403 in Chinese). Ninety two articles with the same data were excluded. After excluding reviews, case reports, and expert letters, 215 articles were screened and obtained. The reviewers read the full text of the articles to exclude articles of low-quality, articles with incomplete data or articles about non-RCT studies according to the inclusion and exclusion criteria. Ultimately, 10 articles were included in the meta-analysis. The specific process is shown in Figure 1.
The 10 articles reported cerebral blood flow values for 288 patients, relative cerebral blood flow values for 235 patients, and relative cerebral blood volume for 244 patients. All the selected articles had a clear diagnosis criterion. The basic characteristics of the 10 articles are set out in Table 1. Random-sequence generation was described in all the articles. The allocation-hiding method adopted was not clear in any of the article descriptions. Nine articles were unclear about the use of the double-blind method. One article was deemed high risk in its use of the double-blind method. One article described the risk of bias in the evaluation of the blind method. All articles described the result data completely, and there was no selective reporting bias. Seven articles had a low risk of other biases, and 3 articles had a high risk of other biases. The evaluation results are shown in Figure 2.
Table 1
Author | Country | Year | Journal | Tumor recurrence (n) | Treatment effect (n) |
---|---|---|---|---|---|
Jovanovic et al. (15) | Belgrade | 2017 | JBUON | 20 | 20 |
Pan et al. (11) | China | 2018 | J Med Imaging | 34 | 18 |
Razek et al. (17) | Egypt | 2018 | Neuroradiology | 24 | 18 |
Ye et al. (18) | China | 2016 | Experimental and Therapeutic Medicine | 16 | 5 |
Seeger et al. (19) | Germany | 2013 | Academic Radiology | 23 | 17 |
Wang et al. (20) | China | 2018 | Radiotherapy and Oncology | 35 | 34 |
Xu et al. (9) | China | 2017 | Medicine | 17 | 12 |
Manning et al. (12) | USA | 2020 | Journal of Neuro-Oncology | 25 | 7 |
Ozsunar et al. (13) | Turkey | 2010 | Academic Radiology | 13 | 5 |
Wang et al. (21) | China | 2013 | Chinese PLA Medical School | 9 | 16 |
Meta-analysis results
Cerebral blood flow value
A total of 8 studies examined changes in cerebral blood flow between the tumor recurrence and treatment-effect groups. The results of the heterogeneity analysis showed that there was obvious homogeneity (I2=85.3%, P=0.000), so the random-effects model was used. The combined effect size of the random-effects model was MD =1.67, 95% CI: 0.89–2.46 (P=0.000). The results showed that the level of cerebral blood flow in patients in the tumor-recurrence group was higher than that in patients in the treatment-effect group, and the difference was statistically significant (see Figure 3).
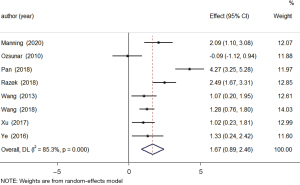
Cerebral relative blood flow
A total of 6 studies examined changes in the relative blood flow of the brain between patients in the tumor-recurrence and treatment-effect groups. The results of the heterogeneity analysis showed that there was obvious homogeneity (I2=48.9%, P=0.082), so the fixed-effects model was used. The combined effect size of the fixed-effects model was MD =1.20, 95% CI: 0.91–1.49 (P=0.000). The results showed that the level of relative blood flow in the brain of patients in the tumor-recurrence group was higher than that of patients in the treatment-effect group, and the difference was statistically significant (see Figure 4).
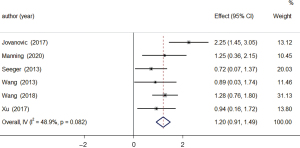
Relative cerebral blood volume
A total of 6 studies examined changes in relative cerebral blood volume between patients in the tumor-recurrence and treatment-effect groups. The results of the heterogeneity analysis showed that there was obvious homogeneity (I2=59.9%, P=0.029), so the fixed-effects model was used. The combined effect size of the fixed-effects model was MD =1.29, 95% CI: 0.98–1.59 (P=0.000). The results showed that the relative cerebral blood volume level of patients in the tumor-recurrence group was higher than that of patients in the treatment-effect group, and the difference was statistically significant (see Figure 5).
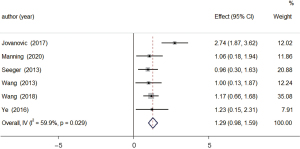
Publication bias
A funnel chart was used to detect relative cerebral blood flow values and relative cerebral blood volumes for publication bias. The relative cerebral blood flow funnel plot was basically symmetrical, indicating that the relative cerebral blood flow was have no publication bias (see Figure 6A). The relative cerebral blood volume funnel chart was asymmetrical, indicating that there may be publication bias in the articles that studied relative cerebral blood flow (see Figure 6B).
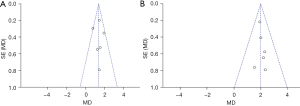
Risk of bias
All articles noted that the random-sequence generation was low risk. All articles had an unclear risk of allocation hidden bias. In relation to blinding participants and personnel, 9 articles (9,11-13,15,17-20) had an unclear risk of bias, and 1 had a high risk of bias (21). All articles described the risk of bias in the blinded outcome assessment [low risk =1 (21), and unclear risk =9 (9,11-13,15,17-20)]. All the articles had a low risk of bias for incomplete outcome data and selective reporting domains. All articles described the risk of other biases [low risk =7 (9,11,12,15,17,19,20), high risk =3 (13,18,21)] (see Figure 7).
Discussion
Glioma is a tumor derived from glial cells in the neuroectoderm. It is the most common primary central nervous system tumor in adults, and accounts for about half of all primary intracranial tumors, and 80% of intracranial malignant tumors (2). It has an incidence of 3–8 people per 100,000 (2). The World Health Organization’s classification of the central nervous system tumors classifies gliomas into grades I–IV; grades I–II include low-grade gliomas, common hairy cell astrocytomas, pleomorphic yellow astrocytoma and ependymal giant cell astrocytoma; grades III–IV are high-grade gliomas. Anaplastic astrocytoma and glioblastoma multiforme are the most common high-grade gliomas, of which glioblastoma accounts for about 50% (15). Treatment options for glioma are limited. At present, the global medical community mainly adopts surgery-based treatments, combined with postoperative radiation therapy, targeted chemotherapy and other comprehensive methods to relieve tumor space-occupying symptoms, delay the recurrence of glioma, and prolong the survival of patients (8,22).
Surgery can only remove tumors that can be seen with the naked eye, and it is impossible to judge the existence of latent cancer cells. The mortality rate of glioma patients is still high (1). After surgery, it is important that patients undergo radiation therapy to treat glioma. Postoperative radiation therapy can improve the survival rate of patients. However, brain radiation injury following radiotherapy, its clinical symptoms. And its appearance on magnetic reissuance imaging (MRI) enhanced scans are very similar to those of tumor recurrence. Brain radiation injury includes pseudo-progression and radiation necrosis (5,23). Radiation necrosis is a serious local tissue reaction to radiotherapy. It usually occurs 3–12 months after radiotherapy, and it may last as long as several years (23,24). Radiation necrosis is related to the range of radiation and radiation dose. Pseudo-progression refers to the early progression of patients after radiotherapy and chemotherapy that occurs within a few weeks of the completion of radiotherapy and chemotherapy. It may be related to the temporary interruption of oligodendrocyte myelin synthesis caused by radiation damage (3,24).
With its good soft tissue contrast, high spatial resolution, and wide applicability, MRI has become an important method for monitoring patients’ responses to glioma treatment. However, an important limitation of conventional enhanced MRI is related to the inability to distinguish between tumor and treatment-related changes. MRPWI is a functional imaging technique that reflects the distribution of micro-vessels and blood perfusion in brain tissue. It can accurately reflect the state of cerebral perfusion caused by recurrence and radiation necrosis, and can identify different perfusion changes caused by tumor neovascularization (3,4,25). There are 3 common MRI perfusion imaging methods: T2-weighted dynamic susceptibility contrast (DSC-MRI), T1-weighted dynamic contrast-enhanced technology (dynamic contrast-enhanced MRI; DCE-MRI), and ASL technology.
When the blood-brain barrier is severely damaged, DSC cannot accurately assess brain perfusion (26). DCE is based on a T1-weighted sequence, with a time resolution lower than DSC, and requires complex pharmacokinetic models to consider the difference between signal intensity and contrast agent concentration (27,28). ASL is a simple and non-invasive MRI perfusion imaging technique that does not require the injection of exogenous contrast agents. It can accurately reflect and monitor brain perfusion information. It is especially suitable for patients with poor renal function who need long-term follow-up care, and the parameters obtained through ASL not only reflect the results of perfusion but also reflect the process of perfusion (29). ASL does not rely on the blood-brain barrier model; thus, it can more sensitively detect changes in blood perfusion, which is helpful for the dynamic monitoring of glioma after surgery (17).
This study included 10 articles, comprising 216 patients with glioma recurrence and 152 patients with treatment effects, for analysis. After the comparative analysis, it was found that the cerebral blood flow, relative cerebral blood flow, and relative cerebral blood volume of patients with glioma recurrence were higher than those with treatment effect, and there were significant differences among the 3 indicators. Thus, ASL has potential value in identifying the recurrence of glioma and radiation-related effects after treatment. In further research, we will analyze whether ASL has similar application value to other tumors.
This study had several limitations. First, the number of studies included in the meta-analysis was relatively small, and the diagnostic thresholds used in each study were different. Further research, including prospective comparative evaluations of post-processing methods, and direct comparisons with other perfusion imaging methods is necessary to more accurately evaluate the diagnostic value of different perfusion imaging methods. Due to the limited number of included studies, this meta-analysis cannot start a deeper discussion.
Conclusions
ASL imaging does not require exposure to radioactivity or the injection of exogenous contrast agents. Its non-invasive and quantitative nature makes this technology especially suitable for patients who cannot tolerate contrast agents, such as elderly, children, and renal insufficient patients, and those who require long-term follow-up care. In combination with MRI structure images, it can be directly used to monitor the condition of patients after glioma surgery and evaluate the treatment effect. It has unique advantages in the early recognition of recurrence changes and the identification of radiation brain injury.
Acknowledgments
Funding: None.
Footnote
Reporting Checklist: The authors have completed the PRISMA reporting checklist. Available at https://dx.doi.org/10.21037/apm-21-3319
Conflicts of Interest: All authors have completed the ICMJE uniform disclosure form (available at https://dx.doi.org/10.21037/apm-21-3319). The authors have no conflicts of interest to declare.
Ethical Statement: The authors are accountable for all aspects of the work in ensuring that questions related to the accuracy or integrity of any part of the work are appropriately investigated and resolved.
Open Access Statement: This is an Open Access article distributed in accordance with the Creative Commons Attribution-NonCommercial-NoDerivs 4.0 International License (CC BY-NC-ND 4.0), which permits the non-commercial replication and distribution of the article with the strict proviso that no changes or edits are made and the original work is properly cited (including links to both the formal publication through the relevant DOI and the license). See: https://creativecommons.org/licenses/by-nc-nd/4.0/.
References
- Le Rhun E, Taillibert S, Chamberlain MC. Anaplastic glioma: current treatment and management. Expert Rev Neurother 2015;15:601-20. [Crossref] [PubMed]
- Stupp R, Brada M, van den Bent MJ, et al. High-grade glioma: ESMO Clinical Practice Guidelines for diagnosis, treatment and follow-up. Ann Oncol 2014;25:iii93-101. [Crossref] [PubMed]
- Xu F, Gao Y, Ni W, et al. Patterns of local failure in patients with high-grade glioma after postoperative radiotherapy with or without chemotherapy. Transl Cancer Res 2019;8:985-91. [Crossref]
- Fatterpekar GM, Galheigo D, Narayana A, et al. Treatment-related change versus tumor recurrence in high-grade gliomas: a diagnostic conundrum--use of dynamic susceptibility contrast-enhanced (DSC) perfusion MRI. AJR Am J Roentgenol 2012;198:19-26. [Crossref] [PubMed]
- Zikou A, Sioka C, Alexiou GA, et al. Radiation Necrosis, Pseudoprogression, Pseudoresponse, and Tumor Recurrence: Imaging Challenges for the Evaluation of Treated Gliomas. Contrast Media Mol Imaging 2018;2018:6828396. [Crossref] [PubMed]
- Bao Z, Wang Y, Wang Q, et al. Intratumor heterogeneity, microenvironment, and mechanisms of drug resistance in glioma recurrence and evolution. Front Med 2021;15:551-61. [Crossref] [PubMed]
- Haider AS, van den Bent M, Wen PY, et al. Toward a standard pathological and molecular characterization of recurrent glioma in adults: a Response Assessment in Neuro-Oncology effort. Neuro Oncol 2020;22:450-6. [Crossref] [PubMed]
- Winter SF, Vaios EJ, Muzikansky A, et al. Defining Treatment-Related Adverse Effects in Patients with Glioma: Distinctive Features of Pseudoprogression and Treatment-Induced Necrosis. Oncologist 2020;25:e1221-32. [Crossref] [PubMed]
- Xu Q, Liu Q, Ge H, et al. Tumor recurrence versus treatment effects in glioma: A comparative study of three dimensional pseudo-continuous arterial spin labeling and dynamic susceptibility contrast imaging. Medicine (Baltimore) 2017;96:e9332. [Crossref] [PubMed]
- Falk Delgado A, De Luca F, van Westen D, et al. Arterial spin labeling MR imaging for differentiation between high- and low-grade glioma-a meta-analysis. Neuro Oncol 2018;20:1450-61. [Crossref] [PubMed]
- Pan F, Zhu Y, Yao L, et al. Application of 3D-ASL in the diagnosis and differential diagnosis of postoperative recurrence in high-grade glioma. J Med Imaging 2018;28:1234-7.
- Manning P, Daghighi S, Rajaratnam MK, et al. Differentiation of progressive disease from pseudoprogression using 3D PCASL and DSC perfusion MRI in patients with glioblastoma. J Neurooncol 2020;147:681-90. [Crossref] [PubMed]
- Ozsunar Y, Mullins ME, Kwong K, et al. Glioma recurrence versus radiation necrosis? A pilot comparison of arterial spin-labeled, dynamic susceptibility contrast enhanced MRI, and FDG-PET imaging. Acad Radiol 2010;17:282-90. [Crossref] [PubMed]
- Telischak NA, Detre JA, Zaharchuk G. Arterial spin labeling MRI: clinical applications in the brain. J Magn Reson Imaging 2015;41:1165-80. [Crossref] [PubMed]
- Jovanovic M, Radenkovic S, Stosic-Opincal T, et al. Differentiation between progression and pseudoprogresion by arterial spin labeling MRI in patients with glioblastoma multiforme. J BUON 2017;22:1061-7. [PubMed]
- Abdel Razek AAK, Talaat M, El-Serougy L, et al. Clinical Applications of Arterial Spin Labeling in Brain Tumors. J Comput Assist Tomogr 2019;43:525-32. [Crossref] [PubMed]
- Razek AAKA, El-Serougy L, Abdelsalam M, et al. Differentiation of residual/recurrent gliomas from postradiation necrosis with arterial spin labeling and diffusion tensor magnetic resonance imaging-derived metrics. Neuroradiology 2018;60:169-77. [Crossref] [PubMed]
- Ye J, Bhagat SK, Li H, et al. Differentiation between recurrent gliomas and radiation necrosis using arterial spin labeling perfusion imaging. Exp Ther Med 2016;11:2432-6. [Crossref] [PubMed]
- Seeger A, Braun C, Skardelly M, et al. Comparison of three different MR perfusion techniques and MR spectroscopy for multiparametric assessment in distinguishing recurrent high-grade gliomas from stable disease. Acad Radiol 2013;20:1557-65. [Crossref] [PubMed]
- Wang YL, Chen S, Xiao HF, et al. Differentiation between radiation-induced brain injury and glioma recurrence using 3D pCASL and dynamic susceptibility contrast-enhanced perfusion-weighted imaging. Radiother Oncol 2018;129:68-74. [Crossref] [PubMed]
- Wang Y. Study on radiation-induced brain injury in postoperative glioma patients using advanced functional MRI techniques. Beijing: Chinese PLA Medical School 2013:16-42.
- Zhang Q, Cao J, Zhang J, et al. Differentiation of Recurrence from Radiation Necrosis in Gliomas Based on the Radiomics of Combinational Features and Multimodality MRI Images. Comput Math Methods Med 2019;2019:2893043. [Crossref] [PubMed]
- Kong Z, Yan C, Zhu R, et al. Imaging biomarkers guided anti-angiogenic therapy for malignant gliomas. Neuroimage Clin 2018;20:51-60. [Crossref] [PubMed]
- Delgado-López PD, Riñones-Mena E, Corrales-García EM. Treatment-related changes in glioblastoma: a review on the controversies in response assessment criteria and the concepts of true progression, pseudoprogression, pseudoresponse and radionecrosis. Clin Transl Oncol 2018;20:939-53. [Crossref] [PubMed]
- van Dijken BRJ, van Laar PJ, Smits M, et al. Perfusion MRI in treatment evaluation of glioblastomas: Clinical relevance of current and future techniques. J Magn Reson Imaging 2019;49:11-22. [Crossref] [PubMed]
- Muto M, Frauenfelder G, Senese R, et al. Dynamic susceptibility contrast (DSC) perfusion MRI in differential diagnosis between radionecrosis and neoangiogenesis in cerebral metastases using rCBV, rCBF and K2. Radiol Med 2018;123:545-52. [Crossref] [PubMed]
- Metaweh NAK, Azab AO, El Basmy AAH, et al. Contrast-Enhanced Perfusion MR Imaging to Differentiate Between Recurrent/Residual Brain Neoplasms and Radiation Necrosis Asian Pac J Cancer Prev 2018;19:941-8.
- Paldino MJ, Barboriak DP. Fundamentals of quantitative dynamic contrast-enhanced MR imaging. Magn Reson Imaging Clin N Am 2009;17:277-89. [Crossref] [PubMed]
- Williams DS, Detre JA, Leigh JS, et al. Magnetic resonance imaging of perfusion using spin inversion of arterial water. Proc Natl Acad Sci U S A 1992;89:212-6. [Crossref] [PubMed]