The value of dynamic monitoring of procalcitonin in the early identification of pathogens and prognosis of bloodstream infections in the ICU
Introduction
Bloodstream infections are a leading cause of death in critically ill patients in the intensive care unit (ICU), with a mortality rate of 20% to 40% (1,2). Rapid and effective early identification of pathogens and their elimination by precise anti-infection treatment are key to improving the efficiency of bloodstream infection treatment and the patient survival rate. Currently, the identification of microorganisms in bloodstream infections still relies on blood culture, but this method has a low positive rate, false-positive misclassification, and delayed return of results, which limits its guiding value for the early diagnosis and treatment of bloodstream infections. Although peripheral blood C-reactive protein (CRP) and leukocyte count (WBC) tests need only a short time, they are less specific. It has been shown that the serum procalcitonin (PCT) assay is simple to operate and requires less time, which can be used to diagnose bloodstream infections in the early stage and help to distinguish between real bloodstream infection and false-positive blood culture caused by pollution (3,4). At the same time, it has high application value in distinguishing Gram-negative (G−) from Gram-positive (G+) bacteria infection.
Whether there are differences in PCT changes in bloodstream infections caused by different G− bacterial species and the relationship between PCT dynamics and prognosis have not been studied. Through a retrospective clinical analysis of 84 patients with bloodstream infections, this paper aims to explore the value of serum PCT dynamic monitoring in the early identification of different pathogenic bacteria genera and species and the prognosis of patients with bloodstream infections. We present the following article in accordance with the STARD reporting checklist (available at https://dx.doi.org/10.21037/apm-21-3232).
Methods
A nonrandomized retrospective cohort study was performed on 94 patients with sepsis accompanied by positive blood bacterial culture in the ICU of Shanghai Changzheng Hospital in China from January 2018 to December 2018. The sepsis diagnostic criteria followed the definition and diagnostic criteria of sepsis 3.0 jointly published by the American Society of Critical Care Medicine (SCCM) and the European Society of Intensive Care Medicine (ESICM) in 2016. The inclusion criteria were as follows: at least 1 positive blood culture result and consecutive positive blood culture results of the same patient with the same single strain of infection. The exclusion criteria were as follows: (I) patients with a single blood culture suggestive of mixed infection; (II) patients with multiple blood cultures suggestive of different strains of infection during the same course of illness; (III) patients with a high suspicion of contamination in the blood culture. Nine ineligible cases were removed, and 84 cases were qualified and included in this study. The study was approved by Medical Ethics Committee of Suixi County Hospital, Anhui Province, China (No. KY-2018003) and written informed consent was obtained from all patients. All procedures performed in this study involving human participants were in accordance with the Declaration of Helsinki (as revised in 2013). To increase the positive rate of each blood culture, venous blood was drawn from two sites separately and placed in aerobic and anaerobic vials for each send, and the volume of blood collected from adults was 8 to 10 mL, chosen before the application of antibiotics (5-8). To avoid contamination, blood was retained from various venous catheters that had been left in place, and in case of suspected catheter-associated bloodstream infection, 1 of the sites was retained from the catheter for blood culture. PCT testing was performed by chemiluminescence using a PCT kit manufactured by Roche Inc. The study was registered in the Chinese clinical trial registry (ChiCTR2100053407).
Observation targets
General clinical data such as gender and age of the enrolled patients were included. PCT values on the day of blood culture (i.e., the first day) and the third and fifth days, and infection indicators such as WBC and CRP on the day of treatment were recorded. The final blood culture results and the clinical outcome (survival/death) after treatment were recorded. This study was an open trial.
Patients with different bacteria were divided into the G− bacteria group and non-G− bacteria group (including G+ bacteria and fungi) using blood culture as the gold standard. The PCT values of the two groups on the first day were analyzed by a receiver operating characteristic (ROC) curve. The sensitivity and specificity of PCT in predicting G− bacteria bloodstream infection were determined by the ROC curve, with the maximum Youden index as the critical value. On the other hand, patients were divided into the survival group and death group according to their different final prognosis. Univariate analysis was conducted for each parameter between the two groups, and independent risk factors or protective factors affecting prognosis were determined by logistic binary regression analysis. Finally, an ROC curve was used to analyze the relationship between the factors and the prognosis. The sensitivity and specificity of this index for predicting the prognosis of G− bacteria bloodstream infection were determined by taking the maximum point of the Youden index as the critical value.
Statistical analysis
The data obtained were statistically analyzed using SPSS 25.0 statistical software. The measurement data were expressed as mean ± standard deviation (). The two independent sample t-test was used to analyze the differences between groups of continuous variables conforming to the normal distribution. The PCT distribution of different strains was more dispersed, so quartiles were selected for comparison, and the non-parametric rank-sum test was used to test the significance of median difference. Count data were expressed as rates, and the χ2 test was used for comparisons between groups. The correlation between each risk factor and prognosis was determined by logistic binary regression analysis. Differences were considered statistically significant at P≤0.05.
Results
General characteristics
According to the etiology of blood culture, 84 patients were divided into 3 groups, including 41 cases of G− bacteria (48.81%), 40 cases of G+ bacteria (47.62%), and 3 cases of fungi (3.57%). There were 27 males and 14 females in the G− bacteria group. The age distribution of patients was 19–87 years old, with an average age of 57.66±20.01 years old. There were 28 males and 12 females in the G+ bacteria group. The age distribution of patients was 15–95 years old, with an average age of 60.93±18.66 years old. In the fungal group, there were 2 males and 1 female. The patients were 52–80 years old with an average age of 65.33±14.05 years old. There was no significant difference in gender and age among the three groups (P>0.05).
Etiology of bloodstream infection
Among the 84 patients with bloodstream infections, 41 strains (48.81%) of G− bacteria, 40 (47.62%) of G+ bacteria, and 3 (3.57%) of fungi were found. The top 3 G− bacteria were Klebsiella pneumoniae, Escherichia coli, and Acinetobacter baumannii, and the top 3 G+ bacteria were Staphylococcus epidermidis, Staphylococcus cephalus, and Staphylococcus lentus. The specific composition ratio is shown in Table 1.
Table 1
Grouping | Number | Constituent ratio (%) |
---|---|---|
Gram-negative bacteria | 41 | 48.81 |
Klebsiella pneumoniae | 24 | 28.57 |
Escherichia coli | 5 | 5.95 |
Acinetobacter baumannii | 4 | 4.76 |
Serratia marcescens | 2 | 2.38 |
Stenotrophomonas maltophilia | 1 | 1.19 |
Pseudomonas aeruginosa | 1 | 1.19 |
Enterobacter cloacae | 1 | 1.19 |
Proteus mirabilis | 1 | 1.19 |
Bacteroides monoform | 1 | 1.19 |
Melanin-producing prevos | 1 | 1.19 |
Gram-positive bacteria | 40 | 47.62 |
Staphylococcus epidermidis | 11 | 13.10 |
Staphylococcus cephalus | 7 | 8.33 |
Staphylococcus lentus | 4 | 4.76 |
Staphylococcus hominis | 3 | 3.57 |
Hemolytic staphylococcus | 4 | 4.76 |
Staphylococcus aureus | 1 | 1.19 |
Other positive bacteria | 10 | 11.9 |
Fungi group | 3 | 3.57 |
Changes in serum PCT levels
PCT levels of different etiologies of bloodstream infection on the first day
The results showed that the median level of PCT on the first day in the G− bacteria group, the G+ bacteria group and the fungus group was 6.4, 0.54, and 2.12 ng/mL, respectively. The mean level of serum PCT in the G− bacteria group was the highest, while that in the fungal infection group was the lowest, and the mean level of PCT in patients with G+ bacteria was between these two groups. Comparison between groups showed that the PCT value of the G− bacteria group was higher than that of the G+ bacteria group and fungus group, and the difference was statistically significant (P<0.05). There was no significant difference in PCT values between the G+ group and the fungus group (P>0.05), as shown in Table 2.
Table 2
Group | n | P25 | P50 | P75 | P value | Mean ± standard deviation | P value |
---|---|---|---|---|---|---|---|
G− bacteria | 41 | 1.6 | 6.40 | 29.73 | <0.001 | 22.71±33.76 | 0.000#1 |
G+ bacteria | 40 | 0.23 | 0.54 | 1.53 | 2.26±7.49 | 0.00#2 | |
Fungus | 3 | 0.23 | 2.12 | 1.74±1.37 | 0.66#3 |
#1, the comparison between the G− bacteria group and the G+ bacteria group; #2, the comparison between the G− bacteria group and the fungi group; #3, the comparison between the G+ bacteria group and the fungi group. PCT, procalcitonin.
ROC curve analysis of PCT levels between patients with G− bacteria bloodstream infections and non-G− bacteria infections
Patients with different etiological bloodstream infections were divided into the G− bacteria group and the non-G− bacteria group (including G+ bacteria and fungi). The PCT values of the two groups on the first day were used for ROC curve analysis of G− bacteria infection. The maximum point of the Youden index was taken as the critical value. When the threshold value was 3.84 ng/mL, the sensitivity and specificity of PCT under the ROC curve for predicting bloodstream infection of G− bacteria were 61% and 92.5%, respectively, and the area under the ROC curve was 0.841, as shown in Figure 1. It is suggested that the significant increase in PCT level in the prediction of G− bacteria bloodstream infection has general sensitivity and good specificity.
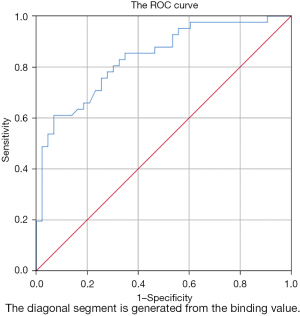
Changes in serum PCT levels of different G− strains on the first day of bloodstream infection
The three most common G− bacteria were Klebsiella pneumoniae, Acinetobacter baumannii, and Escherichia coli. The three groups of bacteria and the remaining G− bacteria were divided into four groups to compare the PCT levels between groups. Due to the small number of cases included in the statistics, PCT values showed a non-normal distribution after grouping. PCT values of each group were represented by quartiles, as shown in Table 3. The non-parametric K-W test between groups showed no significant difference in PCT values of patients with bloodstream infections caused by G− bacteria (P>0.05), as shown in Table 4.
Table 3
Percentile | E. coli | Acinetobacter baumannii | Klebsiella pneumoniae | Other G− bacteria |
---|---|---|---|---|
P25 | 9.36 | 0.62 | 2.7450 | 0.39 |
P50 | 25.40 | 5.07 | 7.23 | 0.86 |
P75 | 86.11 | 77.0 | 15.78 | 26.02 |
PCT, procalcitonin
Table 4
Strains | n | Median (ng/mL) | Chi-square | Degrees of freedom | P value |
---|---|---|---|---|---|
G− bacteria | 41 | 6.4 | 6.35 | 3 | 0.096 |
K-W test, Kruskal-Wallis test; PCT, procalcitonin.
Analysis of influencing factors of prognosis for patients with G− bacteria bloodstream infections
Patients with G− bacteria bloodstream infections were divided into the survival group (n=25) and death group (n=16) according to prognosis. Gender, age, WBC, and CRP values on the first day, and serum PCT values on the first, third, and fifth days were recorded. The decrease rate of PCT on the third day (PCT(D1-D3)/D1) and the decrease rate of PCT on the fifth day (PCT(D1-D5)/D1) of the two groups were calculated, and the differences of parameters between the two groups were compared. The two independent sample t-test was used to compare the continuous variables conforming to the normal distribution. There were no significant differences in age, sex, CRP on day 1, PCT on day 1 (PCTD1), and PCTD3 (P>0.05), but there were significant differences in WBC value on day 1, PCT on day 5 (PCTD5), PCT(D1-D3)/D1, and PCT(D1-D5)/D1 between the two groups (P<0.05), as shown in Table 5.
Table 5
Factors | Survival group | Death group | P value |
---|---|---|---|
Age | 58.68±18.56 | 56.06±22.64 | 0.69 |
Gender (male/female) | 16/9 | 11/5 | 0.75 |
WBC (×109/L) | 13.12±7.47 | 26.76±25.55 | 0.02 |
CRP (mg/L) | 100.13±108.34 | 155.56±116.56 | 0.14 |
PCTD1 (ng/mL) | 21.32±34.38 | 24.88±33.75 | 0.75 |
PCTD3 (ng/mL) | 6.10±11.06 | 14.39±21.07 | 0.11 |
PCTD5 (ng/mL) | 1.62±2.59 | 15.52±17.58 | 0.000 |
PCT(D1-D3)/D1 (%) | 53.44±27.28 | −15.06±145 | 0.03 |
PCT(D1-D5)/D1 (%) | 69.52±35.26 | −62.94±199.14 | 0.002 |
WBC, white blood cell; CRP, C-reactive protein; PCTD1, procalcitonin levels on the first day; PCTD3, procalcitonin levels on the third day; PCTD5, procalcitonin levels on the fifth day; PCT(D1-D3)/D1, the reduction rate of procalcitonin after 3 days of treatment; PCT(D1-D5)/D1, the reduction rate of procalcitonin after 5 days of treatment.
In the univariate analysis, the parameters that showed significant differences between the survival group and the death group were WBC, PCTD5, PCT(D1-D3)/D1, and PCT(D1-D5)/D1. Since PCTD5 was closely related to the decline rate of PCT(D1-D5)/D1, it was not included in the multivariate logistic binary regression analysis. Age may have a certain effect on the prognosis, so it was still included in the analysis to determine the independent risk factors for the prognosis of patients with G− bacteria bloodstream infections. The results showed that PCT(D1-D5)/D1 alone was a protective factor for the survival of patients with G− bacteria bloodstream infections, as shown in Table 6.
Table 6
Factors | B | Standard error | Wald | Degrees of freedom | P value | Exp(B) |
---|---|---|---|---|---|---|
WBC | 0.05 | 0.04 | 1.70 | 1 | 0.19 | 1.05 |
Age | 0.003 | 0.02 | 0.22 | 1 | 0.89 | 1.003 |
PCT(D1-D3)/D1 | 1.65 | 1.74 | 0.895 | 1 | 0.344 | 5.18 |
PCT(D1-D5)/D1 | −2.33 | 1.18 | 3.90 | 1 | 0.048 | 0.098 |
WBC, white blood cell; PCT(D1-D3)/D1, the reduction rate of procalcitonin after 3 days of treatment; PCT(D1-D5)/D1, the reduction rate of procalcitonin after 5 days of treatment.
The PCT(D1-D5)/D1 of patients in the survival group and the death group were analyzed by an ROC curve. The maximum point of the Youden index was taken as the critical value. When the threshold value was 36.02%, the sensitivity, specificity, and area under the ROC curve for predicting patient survival were 88%, 68.7%, and 0.768, respectively, as shown in Figure 2.
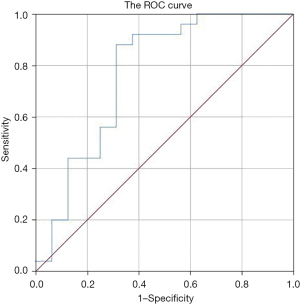
Discussion
BSI is a life-threatening condition caused by the invasion of pathogenic microorganisms into the blood. In recent years, with the widespread use of antibiotics and invasive treatment techniques, bloodstream infections caused by multi-drug resistant (MDR) bacteria have become an important factor threatening the lives of critically ill patients (9,10). In the early stage of bloodstream infection, rapid and accurate identification of the pathogenic species and precise antibiotic treatment to clear it can prevent patients from entering the pathophysiological stages which are difficult to treat, such as infectious shock and multi-organ dysfunction. These factors are key to improving the effective rate of bloodstream infection treatment.
Blood cultures are still the gold standard for identifying bloodstream infections. However, blood cultures themselves have certain drawbacks. Firstly, the positive rate of blood cultures is low, ranging from 10% to 25% for a single blood culture, and a certain percentage of patients with negative blood cultures still have unidentified bloodstream infections and thus fail to receive accurate anti-infective therapy (11,12). Secondly, blood cultures are at risk of contamination during collection, transit, and identification, especially for coagulase-negative gram-positive cocci, which can have a false-positive blood culture rate of 14–26% (13). In addition is the delay in the test report, as the waiting time for blood culture results usually takes more than 48 hours, and some positive results return even after 5 days. As these results are the basis for antibiotic selection, this can significantly delay treatment. Therefore, it is difficult to achieve the clinical principle of early, adequate, and accurate anti-infective treatment.
In recent years, the distribution of pathogens causing BSI has changed significantly, and g-bacterium has gradually occupied the first place of pathogens causing BSI (14,15). Among the 84 patients with bloodstream infection in this study, there were 41 strains of G− bacteria, accounting for 48.81%, and 40 strains of G+ bacteria, accounting for 47.62%, among which 24 cases of Klebsiella pneumoniae were the most, accounting for 28.57%, followed by 11 cases of Staphylococcus epidermidis, accounting for 13.1%.In addition, this study showed that in ICU bloodstream infections, the top 3 G− bacteria were Klebsiella pneumoniae, Escherichia coli, and Acinetobacter baumannii, and the top 3 G+ bacteria were Staphylococcus epidermidis, Staphylococcus cephalus, and Staphylococcus slowus. In the ICU, bloodstream infections were most commonly caused by Klebsiella pneumoniae and were mostly MDR, which in turn can make the accuracy of early empirical treatment difficult. There are some limitations in this study. Among the G+ bacteria, if only 1 vial is positive, colonization or contamination cannot be excluded. Furthermore, due to the small sample size for G+ bacteria, representative strains of clinically common G+ bacteria, such as Streptococcus as well as Enterococcus faecalis and Enterococcus faecalis, are lacking. Also, due to the small sample size, Klebsiella pneumoniae subspecies could not be divided into highly virulent and classical species for comparison.
Numerous studies have suggested that serum PCT level is significantly elevated in bacterial and fungal infections, and its specificity is superior to that of CRP as well as other short-acting reactants (16), which is highly reliable in the early diagnosis of sepsis, severe sepsis, and infectious shock. The present study showed that serum PCT level was most significantly elevated in G− bacteria bloodstream infection, followed by the G+ bacteria group, and was relatively lowest in the fungal group. However, the difference in PCT level between the fungal and G+ bacteria groups was not statistically significant. In other words, the more pronounced the elevation of serum PCT level accompanying bloodstream infection, the more likely G− bacteria infection and the less likely G+ bacteria or fungal infection. WBC, CRP, and PCT were elevated to varying degrees in patients with G+ bacteria, G− bacteria, and fungal infections, but serum CRP and plasma PCT were higher in G− bacteria BSI patients than in G+ bacteria BSI patients. The reason (17) for this difference is related to the different cell wall components of G− and G+ bacteria, where the specific cell wall component lipopolysaccharide (LPS) of G− bacteria is more likely to produce endotoxins, which can directly induce high levels of PCT and other factors from the target cells of the organism in vitro in the absence of inflammatory factors, while the cell wall of G+ bacteria does not have this component. Therefore, G− bacteria increase the levels of secreted inflammatory factors such as PCT under the dual influence of endotoxins and cytokines, resulting in a higher level of PCT expression in the G− bacteria group than in the G+ bacteria group. When serum PCT level ≥10 µg/L, G− bacteria were the main infection source.
One study showed that a 1 hour delay in effective antibacterial drugs and supportive treatment will lead to a 5–10% increase in mortality in patients with sepsis (18). Therefore, early accurate identification of pathogenic microorganisms and timely intervention is very important. Many studies have confirmed that PCT has high sensitivity and specificity in the diagnosis of sepsis (19-21), but the diagnostic threshold is different (22). A meta-analysis of 18 studies on the diagnostic value of plasma PCT in sepsis showed that the average sensitivity of plasma PCT was only 0.71, and the area under ROC curve (AUC) was only 0.78, indicating low diagnostic efficiency and low accuracy. It is concluded that the use of plasma PCT alone in the diagnosis of sepsis should be cautious.
A multicenter study in France showed that there were no significant differences in mortality and infection recurrence within 30 days between the PCT guided group and the control group, but the days of antibiotic avoidance in the PCT guided group increased significantly compared with the control group (14.3 and 11.6 days, respectively) (P<0.05) (23). In this study, the PCT values on the first day of G− bacteria group and non-G− bacteria group (including G+ bacteria and fungi) were analyzed by ROC curve. The results showed that when the PCT threshold was 3.84 ng/mL, the sensitivity, specificity and area under ROC curve for predicting gram-negative bacteria bloodstream infection were 61%, 92.5% and 0.841 respectively. Therefore, if the PCT threshold is set at 3.84 ng/mL, PCT has a high specificity in distinguishing whether the etiology of bloodstream infection is G− bacteria or not, which can be used as an auxiliary basis for strengthening the treatment plan for G− bacteria infection in clinic. The clinical effect of this protocol needs to be confirmed by further prospective studies.
To explore the pattern of changes in specific strains, this study also compared the changes in serum PCT values on the first day of bloodstream infection in different strains of G− bacteria. The results of the nonparametric K-S test showed that there was no statistical difference between the groups of Klebsiella pneumoniae, Acinetobacter baumannii, Escherichia coli, and other negative bacteria, indicating that simply observing the changes in PCT is not yet able to help the clinical identification of bloodstream infections with different strains of G− bacteria. Also, the sample size included in this study was small and false negatives might have occurred.
ICU patients with bloodstream infection were critically ill and had a high mortality rate. Among 41 patients with G− bacteria bloodstream infection included in this study, 25 died, with a mortality rate of 60.98%. Multiple studies (24,25) have found that APACHE II score, SOFA score, PCT level, number of organ failure and septic shock are independent risk factors affecting the prognosis of patients with sepsis. The plasma procalcitonin level can not only reflect the severity of infection in sepsis patients (26), but also serve as an indicator to evaluate the effectiveness of subsequent treatment (27). Some studies have found that serum PCT level is associated with the severity and mortality of trauma patients, but it cannot be used as a tool to differentiate between SIRS and sepsis (28). In particular, reduced PCT levels are closely associated with improved survival in trauma patients (29).
Karlsson et al. concluded that the degree of decrease in PCT during treatment did not correlate with prognosis, but a decrease in PCT by more than 50% suggested a good prognosis (30). In this study, patients were divided into the survival group and death group according to different prognostic factors. Single factor analysis was conducted on the prognostic factors of G− bacteria bloodstream infection. The results showed that there was no significant difference in gender, age, CRP value on the first day, PCTD1, and PCTD3 of patients with different prognostic factors (P>0.05). However, WBC on the first day, PCT on the fifth day (PCTD5), and the decrease rate of serum PCT on day 3 and 5 after treatment were significantly different between the two groups (P<0.05). Logistic binary regression analysis showed that the reduction rate of PCT on day 5 of treatment was an independent protective factor for patients with G− bacteria bloodstream infections. When the PCT reduction rate reached 36.02% or more on day 5, the sensitivity and specificity for predicting patient survival were 88% and 68.7%, respectively. Therefore, the greater the reduction of PCT on day 5 after treatment, the higher the survival rate, which is a strong predictor of survival.
In summary, dynamic monitoring of PCT levels can be a good adjunctive method for rapid determination of pathogenic microorganisms in bloodstream infections. Specifically, the more pronounced the elevation of serum PCT level in the early stage, the more likely the presence of G− bacteria infection compared with G+ bacteria or fungal infections, and this is valuable for guiding the rational selection of antibiotic treatment regimens. Therefore, the absolute value of PCT cannot be relied on to judge the prognosis in clinical diagnosis and treatment, but monitoring the dynamic changes of PCT levels (especially day 5) is valuable for predicting the effect of anti-infection treatment and prognosis.
This study is a retrospective analysis, which does not incur additional costs or pain for patients, and does not involve privacy or other medical ethics issues. However, due to the small sample size, especially the small number of fungal bloodstream infection cases, and the relationship between PCT blood collection time and disease onset time and other confounding factors, the results of this study might be biased to some extent. The next step should be a large prospective randomized controlled trial to clarify this issue.
Acknowledgments
Funding: This study was supported by Shanghai Municipal Health Commission (GWV-10.1-XK24), Shanghai Shenkang Hospital Management Group (SHDC2020CR6030-002), and Three-year action plan for talent construction of Changzheng Hospital—Pyramid Talent Project.
Footnote
Reporting Checklist: The authors have completed the STARD reporting checklist. Available at https://dx.doi.org/10.21037/apm-21-3232
Data Sharing Statement: Available at https://dx.doi.org/10.21037/apm-21-3232
Conflicts of Interest: All authors have completed the ICMJE uniform disclosure form (available at https://dx.doi.org/10.21037/apm-21-3232). The authors have no conflicts of interest to declare.
Ethical Statement: The authors are accountable for all aspects of the work in ensuring that questions related to the accuracy or integrity of any part of the work are appropriately investigated and resolved. The study was approved by Medical Ethics Committee of Suixi County Hospital, Anhui Province, China (No. KY-2018003) and written informed consent was obtained from all patients. All procedures performed in this study involving human participants were in accordance with the Declaration of Helsinki (as revised in 2013).
Open Access Statement: This is an Open Access article distributed in accordance with the Creative Commons Attribution-NonCommercial-NoDerivs 4.0 International License (CC BY-NC-ND 4.0), which permits the non-commercial replication and distribution of the article with the strict proviso that no changes or edits are made and the original work is properly cited (including links to both the formal publication through the relevant DOI and the license). See: https://creativecommons.org/licenses/by-nc-nd/4.0/.
References
- Ziegler MJ, Pellegrini DC, Safdar N. Attributable mortality of central line associated bloodstream infection: systematic review and meta-analysis. Infection 2015;43:29-36. [Crossref] [PubMed]
- Corcione S, Angilletta R, Raviolo S, et al. Epidemiology and risk factors for mortality in bloodstream infection by CP-Kp, ESBL-E, Candida and CDI: A single center retrospective study. Eur J Intern Med 2018;48:44-9. [Crossref] [PubMed]
- Liu K, Zhang C. Diagnostic value of serum procalcitonin in blood stream infection of Gram-negative or gram-positive bacteria. J Clin Pulmonol 2018;23:663-5.
- Ma L, Su J. Clinical value of procalcitonin in bloodstream infection. Beijing Medical 2017;39:1037-9.
- Mao M, Wang R. Comparison of positive rate of single and bilateral blood samples in blood culture and analysis of pathogen distribution and drug resistance. Laboratory Medicine 2009;24:911-3.
- Lin J, Gao X, Cui Y, et al. Increased multidrug resistant isolates: new clinical burdens for 66 hospitals in Shanghai, 2015 to 2017. Ann Transl Med 2020;8:112. [Crossref] [PubMed]
- Liu W, Yang Y, Zhang K, et al. Drug resistance of healthcare-associated pathogenic bacteria and carbapenem-resistant Acinetobacter baumannii homology in the general intensive care unit. Ann Palliat Med 2020;9:1545-55. [Crossref] [PubMed]
- Dellinger RP, Levy MM, Rhodes A, et al. Surviving Sepsis Campaign: international guidelines for management of severe sepsis and septic shock, 2012. Intensive Care Med 2013;39:165-228. [Crossref] [PubMed]
- Thomer L, Schneewind O, Missiakas D. Pathogenesis of Staphylococcus aureus Bloodstream Infections. Annu Rev Pathol 2016;11:343-64. [Crossref] [PubMed]
- Christaki E, Giamarellos-Bourboulis EJ. The complex pathogenesis of bacteremia: from antimicrobial clearance mechanisms to the genetic background of the host. Virulence 2014;5:57-65. [Crossref] [PubMed]
- Linsenmeyer K, Gupta K, Strymish JM, et al. Culture if spikes? Indications and yield of blood cultures in hospitalized medical patients. J Hosp Med 2016;11:336-40. [Crossref] [PubMed]
- Wu AP, Liu D, Chen J, et al. Multivariate analysis of blood culture positive rate of ICU patients. Zhonghua Yi Xue Za Zhi 2016;96:2161-4. [PubMed]
- Ramirez P, Gordón M, Cortes C, et al. Blood culture contamination rate in an intensive care setting: Effectiveness of an education-based intervention. Am J Infect Control 2015;43:844-7. [Crossref] [PubMed]
- Jiang ZQ, Wang SD, Feng DD, et al. Epidemiological risk factors for nosocomial bloodstream infections: A four-year retrospective study in China. J Crit Care 2019;52:92-6. [Crossref] [PubMed]
- Hu FP, Guo Y, Zhu D, et al. CHINET surveillance of bacterial resistance across China: report of the results in 2016. Chin J Infect Chemother 2017;17:481-91.
- Schroeder S, Hochreiter M, Koehler T, et al. Procalcitonin (PCT)-guided algorithm reduces length of antibiotic treatment in surgical intensive care patients with severe sepsis: results of a prospective randomized study. Langenbecks Arch Surg 2009;394:221-6. [Crossref] [PubMed]
- van der Geest PJ, Mohseni M, Nieboer D, et al. Procalcitonin to guide taking blood cultures in the intensive care unit; a cluster-randomized controlled trial. Clin Microbiol Infect 2017;23:86-91. [Crossref] [PubMed]
- Pomara C, Riezzo I, Bello S, et al. A Pathophysiological Insight into Sepsis and Its Correlation with Postmortem Diagnosis. Mediators Inflamm 2016;2016:4062829. [Crossref] [PubMed]
- Bele N, Darmon M, Coquet I, et al. Diagnostic accuracy of procalcitonin in critically ill immunocompromised patients. BMC Infect Dis 2011;11:224. [Crossref] [PubMed]
- al-Nawas B, Shah PM. Procalcitonin in patients with and without immunosuppression and sepsis. Infection 1996;24:434-6. [Crossref] [PubMed]
- Huang W, Huang C, Wen M, et al. Changes of procalcitonin and its relationship with prognosis in patients with septic shock. Chinese Critical Care Emergency Medicine 2013;25:467-70.
- Tang BM, Eslick GD, Craig JC, et al. Accuracy of procalcitonin for sepsis diagnosis in critically ill patients: systematic review and meta-analysis. Lancet Infect Dis 2007;7:210-7. [Crossref] [PubMed]
- Bouadma L, Luyt CE, Tubach F, et al. Use of procalcitonin to reduce patients' exposure to antibiotics in intensive care units (PRORATA trial): a multicentre randomised controlled trial. Lancet 2010;375:463-74. [Crossref] [PubMed]
- Zhang Y, Zhuo X, Zhou X, et al. Prognostic evaluation of serum procalcitonin, C-reactive protein and lactic acid in elderly patients with sepsis. Chinese Journal of Geriatric Multiorgan Diseases 2018;17:47-52.
- Zhang J, Guo L, Xie Z, et al. Risk factors influencing prognosis of multidrug-resistant acinetobacter baumannii bloodstream infection in ICU patients. Chinese Journal of Infection and Chemotherapy 2019;19:611-5.
- Londos C, Sztalryd C, Tansey JT, et al. Role of PAT proteins in lipid metabolism. Biochimie 2005;87:45-9. [Crossref] [PubMed]
- Tschaikowsky K. Predictive value of procalcitonin 'interleukin-6' and C-reactive protein for survival in postoperative patients with severe sepsis. J Crit Care 2011;26:54-64. [Crossref] [PubMed]
- Oconnor E, Venkatesh B, Mashongonyika C, et al. Serum procalcitonin and C-reactive protein as markers of sepsis and outcome in patients with neurotrauma and subarachnoid haemorrhage. Anaesth Intensive Care 2004;32:465-70. [Crossref] [PubMed]
- Claeys R, Vinken S, Spapen H, et al. Plasma procalcitonin and C-reactive protein in acute septic shock: clinical and biological correlates. Crit Care Med 2002;30:757-62. [Crossref] [PubMed]
- Karlsson S, Heikkinen M, Pettilä V, et al. Predictive value of procalcitonin decrease in patients with severe sepsis: a prospective observational study. Crit Care 2010;14:R205. [Crossref] [PubMed]
(English Language Editor: C. Betlzar)