Effects of circadian rhythms and treatment times on the response of radiotherapy for painful bone metastases
Introduction
Bone metastases are a common cause of cancer-related pain and occur in 65–75% of advanced breast and prostate cancer patients (1,2) and 15–40% of lung, thyroid and renal cell cancer patients (1,2). In addition to pain, bone metastases may cause complications including hypercalcemia, pathologic fracture, and spinal cord compression which can significantly impact patients’ quality of life (QOL) (1-3). Radiotherapy is a frequently prescribed treatment for the palliation of painful bone metastases, with a typical response rate of 72–74% (4). Radiation also benefits patient QOL by relieving pain and improving physical function, as well as reducing psychological distress (5). Radiation for bone metastases results in good response rates, however this may be improved by aligning treatment times with circadian rhythms, also referred to as chronotherapy.
Chronotherapy describes the delivery of anti-cancer treatments according to circadian rhythms in order to improve treatment outcomes (6). Previous studies have investigated the efficacy of chronotherapy in several patient populations treated with radiotherapy, but had inconsistent findings (7-15). Some studies reported differences in radiation-induced side effects (11-13,15) based on treatment time, while others have also observed an impact on treatment response (7,14). Research regarding the applications of chronotherapy in chemotherapy is more abundant, with studies showing that the efficacy of over 30 chemotherapy drugs can vary by over 50% based on administration time (16). Studies in non-small cell lung cancer (NSCLC), as well as colorectal cancer patients have shown that administering chemotherapy during the time in which normal cells are the least susceptible to damage can reduce treatment toxicities (6,17,18). This allows higher doses of chemotherapy to be administered resulting in improved efficacy. Particularly for colorectal cancer patients, chronotherapy has allowed them to undergo surgical resections of metastases that were previously unresectable (6). In one of several phase III multicentre trials in metastatic colorectal cancer patients, those with chronomodulated chemotherapy infusions had a 51% objective response rate (tumour shrinkage of 50% or more) in comparison to only 29% with flat infusions (P=0.003) (19). Potentially, chronotherapy could be extended to radiotherapy as a way of improving the therapeutic index.
The objective of our study was to determine the effect of treatment time on response rates in cancer patients with painful bone metastases treated with radiotherapy. Previous studies have noted differential responses to chronotherapy based on gender (11,20). As such our study examined response in males and females separately to elucidate these gender-specific outcomes.
Methods
Patients who received conventional radiation treatment for painful bone metastases from January 2000 to December 2010 were eligible for the study. Patients who received treatment to only one site of metastasis with corresponding treatment response data (analgesic information and pain score) were included. This study was approved by the Research Ethics Board.
Data collection
Demographic information including age, gender, Karnofsky Performance Status (KPS), primary cancer site, site of treatment, dose, fraction, treatment response, total daily oral morphine equivalent, and pain score were recorded in a prospectively collected database. Pain score was reported for each patient on a scale of 0 to 10 (where 0 indicates absence of pain and 10 worst possible severity). Treatment time was defined as the start of radiation delivery and was extracted from patient medical records.
Determination of treatment response
Treatment response was defined as complete response, partial response, pain progression, or indeterminate response as defined by the international consensus on palliative radiotherapy endpoints for future clinical trials in bone metastases (21). A complete response was achieved when patients scored pain at the treated site as 0, without any increase in analgesic intake (daily oral morphine equivalent). A reduction of 2 or more in pain on a scale from 0 to 10 without an increase in analgesics, or a 25% reduction in analgesics with no associated increase in pain from baseline was considered a partial response. Pain progression was defined as an increase in pain score of 2 or more with no change in analgesics, or a 25% increase in analgesics but no change or an increase of 1 in pain score above baseline. Any response that did not fall in the previous 3 categories was defined as an indeterminate response (21). Patients with complete or partial response were considered responders, and those with pain progression or an indeterminate response were considered non-responders.
Treatment response was collected at varying months following treatment; therefore, if patients had response data from 2 months following treatment, this response was used in analysis. For patients without 2-month follow-up, response from 3 months following treatment was used, and if patients did not have response at either of these periods, the response 1 month following treatment was used. Months to follow-up was defined as the difference between the date of first treatment and date of follow-up.
Segregation of treatment cohorts
Patients were assigned to either the 8:00 AM–11:00 AM (including before 8 AM), 11:01 AM–2:00 PM, or 2:01 PM–5:00 PM (including after 5 PM) cohort based on all of their treatment times falling into one of these time frames. If patients received multiple fractions of treatment that fell into different time frames, they were re-allocated and analysed requiring ≥80%, ≥70%, and ≥60% of their treatment times to fall into one time frame. Treatment time intervals were selected based on a prior study conducted by Bjarnason et al. that examined protein indicators of cell cycle activity expressed throughout the day (22). Radiotherapy is delivered at our center from 7:00 AM to 7:00 PM, therefore expression of late G1 phase, G1/S boundary, G2 phases were chosen which corresponded to peaks of 11:00 AM, 3:00 PM, 4:00 PM, respectively.
Statistical analyses
Descriptive statistics were conducted on demographic information for all patients using median and inter-quartiles for continuous variables, and proportions for categorical variables. Patient demographics were compared between treatment cohorts (8:00 AM–11:00 AM, 11:01 AM–2:00 PM, and 2:01 PM–5:00 PM) using the Kurskal-Wallis nonparametric or Fisher exact test for continuous or categorical variables, respectively. A two-sided P value of <0.05 was considered statistically significant.
Response type and the number of responders were compared between the three treatment cohorts using the Fisher exact test. For patients receiving multiple fractions, response was compared between those who received treatment at consistent or inconsistent times of day. Patients who received ≥80% of their treatment times within 2 hours of each other, regardless of which time frame they fell in, were considered to have consistent treatment times; those who did not fit this criterion were considered to have inconsistent treatment times. The statistical analyses described above were repeated with males or females exclusively in order to observe any gender differences. All statistical analyses were performed using statistical analysis software (SAS version 9.4 for Windows). A two-sided P value of <0.05 was considered statistically significant.
Results
Patient characteristics
A total of 194 patients treated with conventional radiotherapy were eligible for the study and were included in analysis with a median age of 68 years (inter-quartiles, 58–76 years; range, 33–88 years). The majority of patients were male (62.4%) and most patients had a KPS ≤70 (58.7%). The most prevalent cancer sites were prostate (30.4%), breast (25.3%), and lung (19.6%). Common treatment sites were the pelvis, hips, and lower limbs (45.4%). A majority of patients were treated with 8 Gy in 1 fraction (57.2%), 65 patients (33.5%) received 20 Gy in 5 fractions, 12 patients (6.19%) received 30 Gy in 10 fractions, and the remaining patients were treated with various other doses and fractions. When all treatment times were used to allocate patients to treatment cohorts, 113 patients were analysed; 128 patients were included when ≥80% of treatment times were used for allocation. Table 1 describes patient characteristics and Table 2 summarizes the number of patients allocated to each cohort based on the allocation method.
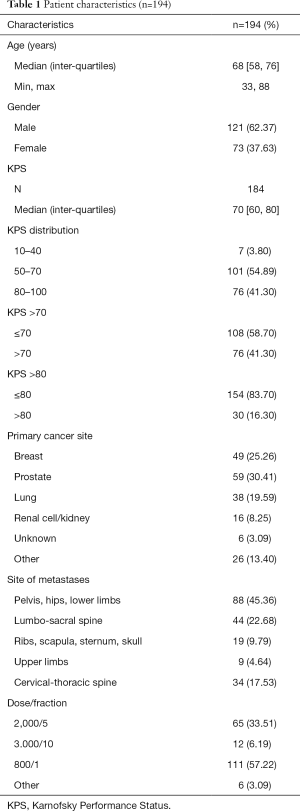
Full table
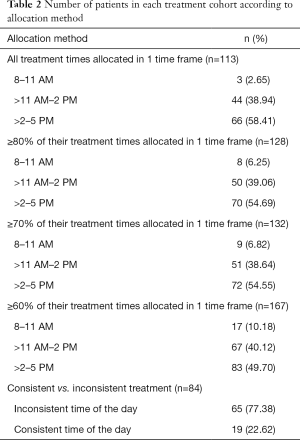
Full table
Using the available response data, 19.4% of patients achieved a complete response to treatment. Most patients had a partial response (36.1%) or indeterminate response (35.6%) to treatment. The remaining 8.9% of patients experienced pain progression. Overall, 106 patients were considered responders (55.5%).
The demographic information of the 8:00 AM–11:00 AM, 11:01 AM–2:00 PM, and 2:01 PM–5:00 PM cohorts did not differ statistically aside from the dose and fraction of radiation received whether all, ≥80%, ≥70%, and ≥60% of treatment times were used for allocation. No significant associations were found between treatment response (or number of responders) and treatment time for all allocation methods (Table 3).
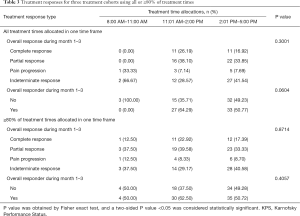
Full table
Females
In females, significant differences in dose and fraction of radiation were again seen between cohorts for all allocation methods except when all treatment times were used. Additionally, when ≥80% and ≥70% of treatment times were used, a significant difference in age was observed between the cohorts (P=0.03), in which the 8:00 AM–11:00 AM cohort consisted of older patients compared to the other two cohorts (Table 4).
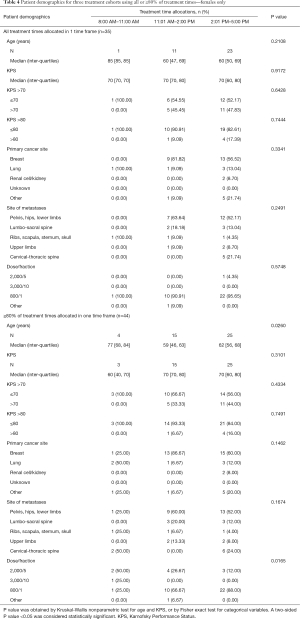
Full table
When female patients were allocated using all of their treatment times, a significant difference in treatment response type (P=0.03) and number of responders (P=0.02) was observed. Patients in the 11:01 AM–2:00 PM cohort had significantly higher proportions of responders (complete or partial response, 72.7%) in comparison to the other 2 cohorts (0% and 27.3% for the 8:00 AM–11:00 AM and 2:01 PM–5:00 PM cohorts, respectively). However, this was not significant when ≥80%, ≥70%, or ≥60% of treatment times were used (Table 5).
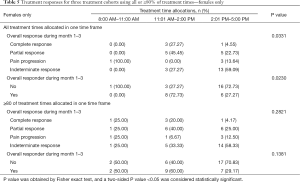
Full table
Males
Males exhibited no significant differences in demographic information except for primary site of malignancy when ≥80% (P=0.03) or ≥70% (P=0.04) of treatment times were used. With these allocation methods, the 11:01 AM–2:00 PM and 2:01 PM–5:00 PM cohorts had many more prostate and lung cancer patients, while the 8:00 AM–11:00 AM cohort had none due to low patient numbers. No differences in treatment response were seen at any time after treatment for all allocation methods.
Inconsistent vs. consistent treatment times
Treatment response types did not differ between patients who received treatment at consistent or inconsistent times of day (P=0.6), nor did the amount of responders when all patients were analysed together (P=0.4). When comparing the amount of responders for females, a significant difference was seen where patients treated at inconsistent times of day had a response rate of 71.4% in contrast to 33.3% in patients with consistent treatment times (P=0.04) (Table 6). No significant differences were seen in males for treatment response type (P=0.1) or amount of responders (P=0.4).
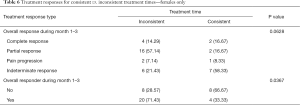
Full table
Discussion
The present study is the first to look at the effect of treatment time on radiotherapy response in patients with bony metastases. All patients were separated into the 3 time cohorts (8:00 AM–11:00 AM, 11:01 AM–2:00 PM, and 2:01 PM–5:00 PM) using different allocation methods. The overall response rate of the 194 patients analysed was 55.5%, which was lower than the reported 72–74% response rate for patients receiving radiotherapy for painful uncomplicated bone metastases (4). Females treated in the 11:01 AM–2:00 PM cohort exhibited a significantly higher proportion of patients with complete or partial response (P=0.03), and higher proportion of responders (P=0.02) in comparison to the remaining two cohorts. Also, significant differences in the proportion of responders were observed between females with inconsistent and consistent treatment times (P=0.04). However, these differences were not seen in males or when all patients were analysed together. Time of radiotherapy delivery and response may be correlated in female patients only.
To date, nine studies have looked at the relationship between radiation treatment time and outcomes in cancer patients with varying results (7-15). Of the eight studies that evaluated treatment response (7,9-15), only two retrospective studies observed significant differences in outcomes between cohorts based on treatment time (7,14). The retrospective study by Rahn et al. observed improved local control (LC) in NSCLC patients treated with stereotactic radiosurgery (SRS) for brain metastases in the morning (10:00 AM–12:30 PM) relative to those treated in the afternoon (12:30 PM–3:00 PM) (P=0.014) (7). However, a larger subsequent study by Badiyan et al. could not reproduce these results potentially indicating that when controlling for confounders and in large patient populations there is no true effect of chronoradiotherapy (8). In a retrospective study conducted by Hsu et al., receiving treatment after 5:00 PM was predictive of a worse 6-year rate of biochemical failure-free survival (BFFS) for prostate cancer patients in a matched cohort (HR =1.95, P=0.05) (14). There are conflicting studies in the literature in regards to the efficacy of chronoradiotherapy of which the true utility is still to be elucidated.
Bones are constantly undergoing bone formation processes by osteoblasts and bone resorption processes by osteoclasts (23). Several studies suggest that the regulation of these complementary processes may be influenced by circadian rhythmicity. Studies by Shao et al. on rats and Generali et al. on breast cancer patients with lytic bone metastases observed circadian rhythmicity in markers of both bone resorption and formation (24,25). The latter study compared these patterns to those in healthy women and found they were very similar (25). Bone mineralization also seems to exhibit circadian rhythmicity as shown in the study by McElderry et al. where 24-hour patterns in mineral deposition and Per1 expression were seen in calvarial organs (26). Additionally, the blocking of certain clock genes in mice resulted in increased bone mass, suggesting that the equilibrium of bone remodelling is dependent on circadian rhythms (27). The rate of production of osteoblasts by bone mesenchymal stem cells has also been correlated to the expression levels of specific clock genes (28). Circadian rhythms may alter rates of osteogenesis and impact bone remodelling; this effect could extend to osteolytic and osteoblastic metastases as well, thus potentially producing differential responses to radiotherapy depending on the time of day. Our study found that treatment time was correlated to response in females, supporting the theory that circadian rhythms can impact the radiosensitivity of bone metastases.
A previous prospective study by Bjarnason et al. observed that men and women exhibited opposing trends in the incidence of mucositis in response to timing of radiotherapy for head and neck cancer patients (11). In chemotherapy trials, female colon cancer patients had more toxicity, as well as worse survival in comparison to males undergoing the same chronomodulated treatment (18,20). Specifically, Lévi et al. saw that female patients experienced almost twice as many severe toxicities as men overall after treating patients at different times of day (18). The chronotolerance profile in females and males varied in that men experienced the greatest tolerability at the previously determined optimal treatment time, while females seemed to have the best tolerability 6 hours later (18). The underlying reason for these sex-dependent responses are unknown, but females may be more susceptible to external stimuli that impact the circadian system (18). For instance, one study used light to suppress melatonin rhythmicity and observed a larger degree of suppression in females than males (29). Therefore, suboptimal timing of chronotherapy may have a larger impact in females as they exhibit increased variability in circadian rhythmicity. This variability may explain why differences in radiotherapy treatment response as a function of treatment time only occurred in females, as reported by our study. The role of gender-specific rhythms in chronotherapy warrants further investigation in future studies.
Circadian rhythms may differ based on the area of the body. Scheving et al. looked at five regions of the gastrointestinal tract in male mice and found that the peak rates of DNA synthesis differed in amplitude and timing depending on the region studied (30). In our study, the sites of metastases included the pelvis, spine, ribs, skull, scapula, and limbs. Therefore, it is possible that we observed no significant difference in response based on treatment time because several sites with different circadian rhythms were analysed. It may be necessary in future prospective studies to compare response rates between time cohorts by treatment site, in order to account for the potential differences in circadian rhythms of these sites.
Disruptions in circadian rhythms may increase cell proliferation and genome instability, contributing to the progression and development of cancer (31,32). Potentially, restoring disrupted circadian rhythms or maintaining these rhythms may improve the efficacy of chronotherapy as a higher abundance of normal cells will be in a state less susceptible to damage during treatment administration. This circadian synchronization may be achieved using a variety of methodologies including regular scheduling of meals, ongoing physical activity, bright light therapy, and pharmaceuticals (31). Melatonin has been shown to influence rhythms in the circadian pacemaker, the suprachiasmatic nuclei (33), while glucocorticoids have been shown to entrain peripheral circadian clocks in other tissues (34). However, data is scarce regarding the ability of pharmaceuticals to synchronize rhythms in cancer patients (31). In the present study, the desynchronization of circadian rhythms between patients may have contributed to our negative findings. The efficacy of circadian synchronization and its subsequent impact on chronotherapy may be an endpoint of interest in future studies.
Our study analysed prospectively collected databases; however, this did not allow for patients to be segregated equally into the different time groups. As well the composition of the different groups was skewed, with certain time intervals consisting of a higher proportion of particular primary cancer sites, which may in fact impact response rates. The patients included in this study attended a morning rapid referral radiation clinic, where consultation and planning occurs in the morning and treatment is often delivered in the afternoon on the same day, which may account for the large deficiency of patients in the 8:00 AM–11:00 AM cohort. Due to low patient numbers and inconsistent follow-up times, it was difficult to assess differences in treatment response at follow-up months 1, 2, and 3 separately. Thus patients with differing times to follow-up were analysed together, even though patients’ response to treatment may change depending on how long after treatment it was assessed.
In our study, a significant difference in the dose and fraction of radiation received differed between the three treatment cohorts, independent of allocation method. However, studies have shown that patients treated with 20 Gy in 5 fractions or 8 Gy in 1 fraction for painful bone metastases have similar treatment response rates (4,35,36). Over 90% of our patients received either 20 Gy in 5 fractions or 8 Gy in 1 fraction, therefore the significant difference in radiation dose and fraction between cohorts is not a limitation of our study.
Conclusions
The present study is the first to investigate how treatment time affects radiotherapy response in cancer patients with painful bone metastases. We conclude that radiotherapy delivery between 11:01 AM and 2:00 PM may improve response rates for women. Future studies should prospectively allocate patients to different times of therapy, with stratification by gender, location and tumour type.
Acknowledgements
The authors thank the generous support of Bratty Family Fund, Michael and Karyn Goldstein Cancer Research Fund, Joey and Mary Furfari Cancer Research Fund, Pulenzas Cancer Research Fund, Joseph and Silvana Melara Cancer Research Fund, and Ofelia Cancer Research Fund.
Footnote
Conflicts of Interest: The authors have no conflicts of interest to declare.
Ethical Statement: This study was approved by the Research Ethics Board of 079-2016.
References
- Costa L, Badia X, Chow E, et al. Impact of skeletal complications on patients' quality of life, mobility, and functional independence. Support Care Cancer 2008;16:879-89. [Crossref] [PubMed]
- Chow E, Nguyen J, Zhang L, et al. International field testing of the reliability and validity of the EORTC QLQ-BM22 module to assess health-related quality of life in patients with bone metastases. Cancer 2012;118:1457-65. [Crossref] [PubMed]
- Coleman RE. Skeletal complications of malignancy. Cancer 1997;80:1588-94. [Crossref] [PubMed]
- Chow E, Zeng L, Salvo N, et al. Update on the systematic review of palliative radiotherapy trials for bone metastases. Clin Oncol (R Coll Radiol) 2012;24:112-24. [Crossref] [PubMed]
- McDonald R, Chow E, Rowbottom L, et al. Quality of life after palliative radiotherapy in bone metastases: A literature review. J Bone Oncol 2014;4:24-31. [Crossref] [PubMed]
- Giacchetti S. Chronotherapy of colorectal cancer. Chronobiol Int 2002;19:207-19. [Crossref] [PubMed]
- Rahn DA 3rd, Ray DK, Schlesinger DJ, et al. Gamma knife radiosurgery for brain metastasis of nonsmall cell lung cancer: is there a difference in outcome between morning and afternoon treatment? Cancer 2011;117:414-20. [Crossref] [PubMed]
- Badiyan SN, Ferraro DJ, Yaddanapudi S, et al. Impact of time of day of gamma knife radiosurgery on survival for non-small cell lung cancer brain metastases. Radiother Oncol 2012;103:S491-2. [Crossref]
- Badiyan SN, Ferraro DJ, Yaddanapudi S, et al. Impact of time of day on outcomes after stereotactic radiosurgery for non-small cell lung cancer brain metastases. Cancer 2013;119:3563-9. [Crossref] [PubMed]
- Kabolizadeh P, Wegner R, Bernard M, et al. The Effect of Treatment Time on Outcome in Non-small Cell Lung Cancer Brain Metastases Treated with Stereotactic Radiosurgery. Int J Radiat Oncol Biol Phys 2011;81:S301. [Crossref]
- Bjarnason GA, Mackenzie RG, Nabid A, et al. Comparison of toxicity associated with early morning versus late afternoon radiotherapy in patients with head-and-neck cancer: a prospective randomized trial of the National Cancer Institute of Canada Clinical Trials Group (HN3). Int J Radiat Oncol Biol Phys 2009;73:166-72. [Crossref] [PubMed]
- Goyal M, Shukla P, Gupta D, et al. Oral mucositis in morning vs. evening irradiated patients: a randomised prospective study. Int J Radiat Biol 2009;85:504-9. [Crossref] [PubMed]
- Shukla P, Gupta D, Bisht SS, et al. Circadian variation in radiation-induced intestinal mucositis in patients with cervical carcinoma. Cancer 2010;116:2031-5. [Crossref] [PubMed]
- Hsu FM, Hou WH, Huang CY, et al. Differences in toxicity and outcome associated with circadian variations between patients undergoing daytime and evening radiotherapy for prostate adenocarcinoma. Chronobiol Int 2016;33:210-9. [Crossref] [PubMed]
- Noh JM, Choi DH, Park H, et al. Comparison of acute skin reaction following morning versus late afternoon radiotherapy in patients with breast cancer who have undergone curative surgical resection. J Radiat Res 2014;55:553-8. [Crossref] [PubMed]
- Lévi F. Circadian chronotherapy for human cancers. Lancet Oncol 2001;2:307-15. [Crossref] [PubMed]
- Li J, Chen R, Ji M, et al. Cisplatin-based chronotherapy for advanced non-small cell lung cancer patients: a randomized controlled study and its pharmacokinetics analysis. Cancer Chemother Pharmacol 2015;76:651-5. [Crossref] [PubMed]
- Lévi F, Focan C, Karaboué A, et al. Implications of circadian clocks for the rhythmic delivery of cancer therapeutics. Adv Drug Deliv Rev 2007;59:1015-35. [Crossref] [PubMed]
- Lévi F, Zidani R, Misset JL. Randomised multicentre trial of chronotherapy with oxaliplatin, fluorouracil, and folinic acid in metastatic colorectal cancer. International Organization for Cancer Chronotherapy. Lancet 1997;350:681-6. [Crossref] [PubMed]
- Giacchetti S, Dugué PA, Innominato PF, et al. Sex moderates circadian chemotherapy effects on survival of patients with metastatic colorectal cancer: a meta-analysis. Ann Oncol 2012;23:3110-6. [Crossref] [PubMed]
- Chow E, Hoskin P, Mitera G, et al. Update of the international consensus on palliative radiotherapy endpoints for future clinical trials in bone metastases. Int J Radiat Oncol Biol Phys 2012;82:1730-7. [Crossref] [PubMed]
- Bjarnason GA, Jordan RC, Sothern RB. Circadian variation in the expression of cell-cycle proteins in human oral epithelium. Am J Pathol 1999;154:613-22. [Crossref] [PubMed]
- Florencio-Silva R, Sasso GR, Sasso-Cerri E, et al. Biology of Bone Tissue: Structure, Function, and Factors That Influence Bone Cells. Biomed Res Int 2015;2015:421746.
- Shao P, Ohtsuka-Isoya M, Shinoda H. Circadian rhythms in serum bone markers and their relation to the effect of etidronate in rats. Chronobiol Int 2003;20:325-36. [Crossref] [PubMed]
- Generali D, Berruti A, Tampellini M, et al. The circadian rhythm of biochemical markers of bone resorption is normally synchronized in breast cancer patients with bone lytic metastases independently of tumor load. Bone 2007;40:182-8. [Crossref] [PubMed]
- McElderry JD, Zhao G, Khmaladze A, et al. Tracking circadian rhythms of bone mineral deposition in murine calvarial organ cultures. J Bone Miner Res 2013;28:1846-54. [Crossref] [PubMed]
- Fu L, Patel MS, Bradley A, et al. The molecular clock mediates leptin-regulated bone formation. Cell 2005;122:803-15. [Crossref] [PubMed]
- He Y, Lin F, Chen Y, et al. Overexpression of the Circadian Clock Gene Rev-erbα Affects Murine Bone Mesenchymal Stem Cell Proliferation and Osteogenesis. Stem Cells Dev 2015;24:1194-204. [Crossref] [PubMed]
- Monteleone P, Esposito G, La Rocca A, et al. Does bright light suppress nocturnal melatonin secretion more in women than men? J Neural Transm Gen Sect 1995;102:75-80. [Crossref] [PubMed]
- Scheving LE, Burns ER, Pauly JE, et al. Circadian variation in cell division of the mouse alimentary tract, bone marrow and corneal epithelium. Anat Rec 1978;191:479-86. [Crossref] [PubMed]
- Innominato PF, Roche VP, Palesh OG, et al. The circadian timing system in clinical oncology. Ann Med 2014;46:191-207. [Crossref] [PubMed]
- Hanahan D, Weinberg RA. Hallmarks of cancer: the next generation. Cell 2011;144:646-74. [Crossref] [PubMed]
- Sack RL, Brandes RW, Kendall AR, et al. Entrainment of free-running circadian rhythms by melatonin in blind people. N Engl J Med 2000;343:1070-7. [Crossref] [PubMed]
- Balsalobre A, Brown SA, Marcacci L, et al. Resetting of circadian time in peripheral tissues by glucocorticoid signaling. Science 2000;289:2344-7. [Crossref] [PubMed]
- Sze WM, Shelley MD, Held I, et al. Palliation of metastatic bone pain: single fraction versus multifraction radiotherapy--a systematic review of randomised trials. Clin Oncol (R Coll Radiol) 2003;15:345-52. [Crossref] [PubMed]
- Wu JS, Wong R, Johnston M, et al. Meta-analysis of dose-fractionation radiotherapy trials for the palliation of painful bone metastases. Int J Radiat Oncol Biol Phys 2003;55:594-605. [Crossref] [PubMed]